DINOSAURS have been extinct for 66 million years, so we must study their fossils to understand the history of this fascinating group of animals. Dinosaur fossils were formed in the environments in which the dinosaurs lived and died. The rocks that contain dinosaur fossils provide clues to the climate and habitats in which they lived. The reign of the dinosaurs began 225 to 230 million years ago and ended 66 million years ago. In this chapter, I examine the nature of dinosaur fossils, the relationship between rocks and ancient sedimentary environments, and the geologic time scale of dinosaur evolution.
The word
fossil literally means “something dug up.” Today, the word generally refers to any physical trace of past life. Most dinosaur fossils are mineralized bones. But, dinosaur fossils also include tracks, eggs, skin impressions, tooth marks, stomach stones (
gastroliths), and fossilized feces (
coprolites) (
figure 3.1). These fossils are called
trace fossils (or ichnofossils, from the Greek word
ichnos, which means “trace”) in contrast to mineralized dinosaur bones, which are termed
body fossils.
Chapter 12 of this book discusses dinosaur trace fossils.
How are fossils formed? How long does it take for a bone to become a fossil? These are common questions asked of paleontologists, the scientists who study fossils. Key to the process of fossilization is for the dinosaur bone (or footprint, egg, etc.) to be buried in sediment (rock particles) (
figure 3.2). When a dinosaur died, it was either buried in sediment immediately or its remains, perhaps after scavenging, weathering, or being transported by running water, were buried somewhat later (
box 3.1). The soft tissues of the dinosaur—skin, muscles, and internal organs—rotted away quickly, whether the dinosaur was buried immediately upon or long after death. Only rarely are soft tissues preserved, as in the case of the duck-billed dinosaur tail fossil with skin impressions shown in
figure 3.3.
FIGURE 3.1
Dinosaur fossils comprise bones and skeletons, tracks, eggs, skin impressions, tooth marks, gastroliths, and coprolites. (© Scott Hartman)
FIGURE 3.2
The process of fossilization involves death, decay, burial, and mineralization. (Drawing by Network Graphics)
FIGURE 3.3
These skin impressions surround part of the tail of a duck-billed dinosaur found in the Upper Cretaceous of New Mexico and are a rare instance of the fossilization of dinosaur soft tissues.
In 1943, Soviet paleontologist Ivan Yefremov (1908–1972) combined the Greek words taphos, meaning “burial,” and nomos, meaning “laws,” to describe a branch of paleontology he called taphonomy. Taphonomy is the study of how fossils are formed and what biases are inherent in the fossil record. By undertaking taphonomic studies, paleontologists gain insight into the behavior and ecology of extinct organisms. They also come to grips with the kinds of information contained in a collection of fossils. They do these things by trying to understand the processes that have taken place between the time an organism, or group of organisms, was alive and the present, when we collect fossils of that organism or group of organisms.
If we imagine a population of dinosaurs alive, say, 100 million years ago, the first step toward fossilization of these animals is, of course, their death (
box figure 3.1). If they die in an accident and their bodies are buried rapidly, such as in a flash flood, then the dinosaurs immediately become a “burial assemblage.” Most dinosaurs, however, probably died in other ways that did not result in the immediate burial of their bodies. They may have been preyed upon by other dinosaurs or died from disease or old age, or in other types of accidents. Also, many dinosaur carcasses must have been scavenged by other animals long before burial. Because most forms of death for dinosaurs did not include burial, taphonomists speak of a “death assemblage” made up of all the dead dinosaurs—whether they were whole carcasses or partial and preyed upon and/or scavenged carcasses—that were not buried at the time of death. Only later, usually through geological processes, such as the accumulation of river sediments around and over bones, did burial occur. The death assemblage is eventually converted into a burial assemblage.
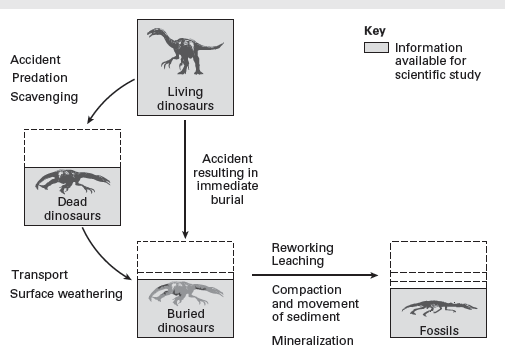
BOX FIGURE 3.1
Taphonomic processes destroy information between the life of a dinosaur and the dinosaur fossils we collect and study. (Drawing by Network Graphics)
This is an important distinction. We would expect the fossils of dinosaurs that enter the burial assemblage immediately upon death to consist of complete or virtually complete skeletons. And, by contrast, we would expect the fossils of dinosaurs that did not immediately enter the burial assemblage to consist of much less complete skeletons. Indeed, the fossils of such dinosaurs would consist mainly of parts of skeletons, isolated bones, and bone fragments. This is because any number of taphonomic processes, including surface weathering of bone, destruction of bone by predators and scavengers, and damage to bone by running water and moving sediment, would have reduced the integrity of a dinosaur skeleton prior to burial. These simple observations show that complete dinosaur skeletons are mostly those of animals buried immediately upon death or very soon after. Many dinosaurs are represented by less complete specimens, whereas the overwhelming majority of dinosaurs left no fossils at all, simply because their skeletons were totally destroyed before they were buried.
Just because a dinosaur skeleton or bone was buried does not mean it necessarily became a fossil. Other taphonomic processes may have intervened between the burial assemblage and the fossil assemblage (see
box figure 3.1). For example, buried dinosaur bones could be exhumed by erosion and weather away before being fossilized. Or, even though buried, a skeleton might not become a fossil because of the fact that the chemistry of the soil and ground water were of the wrong type to lead to fossilization.
We can see that many factors may prevent a dinosaur from becoming a fossil that we can collect and study. Taphonomists stress this aspect of the fossil record by pointing out how much information taphonomic processes have destroyed between the time when the dinosaur was alive and when it became a fossil in a museum. When a dinosaur was alive—a flesh-and-blood, moving animal—it was in a state of 100 percent information. But, as death, burial, and fossilization took place, much information was lost, leaving us with only a small percentage of the original information available from the living dinosaur. Taphonomy helps paleontologists to understand how much information is removed between the life of a dinosaur and the dinosaur fossils we collect and study.
After burial, the remaining bones and teeth typically must undergo some form of
mineralization to become fossils. Bones and teeth are not just inorganic minerals, but contain a significant amount of organic matter. This matter either decays and is replaced by minerals, such as silica, calcite, or iron, or forms complex compounds by combining with these minerals. The mineral replacement or combination takes place on a microscopic scale, so that tiny spaces around the inorganic matrix of the bone are filled with the new minerals. The resulting mineralized bone is thus a combination of the original inorganic bone matrix (a mineral called
calcium phosphate) and the new minerals. However, in some cases, the original inorganic bone matrix is also partly or completely replaced by the new mineral. In most mineralized bones, the quality of microscopic structures is very high, allowing them to be studied under the microscope (
figure 3.4).
The length of time it takes to mineralize bone is not well established. Most paleontologists believe it takes 10,000 years or more for a bone to fossilize. This is because most bones that are 10,000 years old or younger—mainly those excavated at archaeological sites—show little or no mineralization. However, some bones much older than 10,000 years also show little mineralization. Clearly, the rate at which a bone is mineralized depends on the type and chemistry of the sediment within which it is buried, the amount of water in the sediment, and other aspects of the sedimentary environment in which the bone is buried. This makes it difficult to generalize about the rate at which fossilization takes place. But, 10,000 years still remains a good minimum estimate of the time it takes for most bones to fossilize.
The preservation of delicate organic structures, such as skin or eggs, requires a special type of sedimentary environment in which these structures will not be damaged or destroyed. Dinosaurs must have made literally trillions of footprints during the 160 or so million years of their existence. But, with few exceptions, only those footprints made in moist sediment and buried quickly, before they could erode, were preserved as fossil footprints.
FIGURE 3.4
This cross-section of a fossilized dinosaur bone seen under high magnification shows the microscopic detail preserved. Here you can see small vascular canals (dark oval or round holes) that were filled with blood and soft tissue. The lighter-colored areas are mostly the inorganic mineral matrix of the bone. (Courtesy J. M. Rensberger)
Dinosaur fossils of all types are discovered and collected by fossil hunters worldwide. People who collect and study fossils professionally are called paleontologists, from the Greek words for “ancient-life studies.” Dinosaur fossils collected by paleontologists are cleaned and studied in laboratories in museums and universities.
Geologists recognize three types of rock on the surface of the earth. Igneous rocks are those that cool from a molten state. They include all volcanic rocks. Metamorphic rocks are those that have been altered (metamorphosed) by temperature and/or pressure. Common metamorphic rocks are marble, which is limestone before metamorphism, and quartzite, which is sandstone before metamorphism. Sedimentary rocks are those formed by the accumulation and cementation of mineral grains or by chemical (including organic) precipitation at the surface of the Earth. Sedimentary rocks are often the by-products of the erosion of igneous or metamorphic rocks. Sandstone, limestone, shale, and siltstone are common sedimentary rocks. Fossils are very rarely found in igneous or metamorphic rocks. Almost all fossils, and those of dinosaurs are no exception, are found in sedimentary rocks.
The type of sedimentary rock within which a dinosaur fossil is found contains much information about the environment in which the dinosaur lived and died. This is because different types of sedimentary rocks are characteristic of the distinct sedimentary (depositional) environments in which the rocks formed. The process of rock formation encompasses the deposition of sediment (sedimentation) and subsequent compaction and cementation.
The study of sedimentary rocks and their formation is called
sedimentology. Sedimentologists make a broad distinction between
marine (in the sea) and nonmarine (on the land) sedimentary environments (
figure 3.5). Dinosaurs did not live in the sea, so their fossils are seldom found in marine sedimentary rocks. Therefore, we need not concern ourselves very much with marine sedimentary environments. Instead, we can focus mainly on the nonmarine (also called terrestrial) sedimentary environments—fluvial, lacustrine, eolian, and deltaic—where almost all dinosaurs were fossilized.
FIGURE 3.5
Sedimentologists distinguish marine (in the sea) from nonmarine or terrestrial (on the land surface) sedimentary environments. (Drawing by Network Graphics)
Fluvial environments are those in which rivers and streams are the dominant agents of sedimentation (deposition) (
figure 3.6). Because the water is moving in one direction, often quite rapidly, sediments deposited in fluvial environments are typically made up of relatively large particles of sand and gravel. These particles, when cemented, become sandstone and conglomerate. Fluvial sandstones and conglomerates often display structures characteristic of running water, such as ripple marks formed by currents or cross-beds formed on underwater dunes (
figure 3.7). Coarse particle size, ripple marks, and cross-beds are the primary features by which geologists identify fluvial sedimentary rocks.
Most dinosaur fossils are found in fluvial sedimentary rocks. They are especially common in river
floodplain deposits. This is because rapid deposition of sediments is typical of fluvial sedimentary environments. These environments allowed for the large bones of dinosaurs to be buried most rapidly, thus making these bones the most likely to fossilize.
FIGURE 3.6
Fluvial environments are those in which rivers dominate sedimentation. (Drawing by Network Graphics)
FIGURE 3.7
Running water produces cross-beds (A and B) and ripple marks (C). (Drawing by Network Graphics)
FIGURE 3.8
Lacustrine environments are those in which sedimentation takes place in a lake. (Drawing by Network Graphics)
Lacustrine sedimentary environments are those in which deposition takes place in or on the margins of lakes (
figure 3.8). There, the action of water is much gentler than in rivers, so lacustrine sedimentary rocks (consisting of mud and silt) have much smaller particle sizes than fluvial sedimentary rocks. Lacustrine rocks also have different sedimentary structures than fluvial rocks. Typically, they are laminated in thin layers of varying thicknesses that reflect periodic (sometimes seasonal) fluctuations in deposition. And, in lakes where not much mud or silt is involved in deposition, chemical precipitation of calcium carbonate, silica, or gypsum can become the dominant mode of deposition.
A fair number of dinosaur fossils have been found in lacustrine sedimentary rocks. Most of these dinosaur fossils, however, were found in the rocks that represent shoreline or river-delta deposits on lake margins. This suggests that few, if any, dinosaurs were actually aquatic and lived in lakes.
Eolian environments are those in which wind is the major agent of deposition. Wind-blown sediments typically display the same types of sedimentary structures—ripple marks and cross-beds—as fluvial sediments. But, wind generally cannot move larger particles like running water can, so eolian sedimentary rocks are fine-grained sandstones and siltstones. Also, wind tends to sort and abrade the particles it moves, giving the particles a more even size distribution and rounder shapes than particles moved by water. Cross-bedded, ripple-marked, fine-grained, rounded, well-sorted (consisting of grains of similar size) sandstones are typical rocks formed in ancient deserts (
figure 3.9).
FIGURE 3.9
This Middle Jurassic cross-bedded sandstone in New Mexico was deposited in an eolian environment.
Few body fossils of dinosaurs were formed in eolian sediments because very little water was available for mineralization, but fossil footprints of dinosaurs abound in some eolian sedimentary rocks.
A delta is a triangular body of sediment formed where a river enters a large, quiet body of water, either a sea or lake. So,
deltaic sediments are similar to fluvial sediments in many features. But the overall shape (geometry) of deltaic sediments and their proximity to lacustrine or
marine sediments reveal their place of origin. Because deposition is often rapid in deltas, many dinosaur fossils, especially those of dinosaurs that lived near or along seashores, are preserved in deltaic deposits (
figure 3.10).
FIGURE 3.10
These rocks in New Mexico, formed by river deltas 73 million years ago, are loaded with dinosaur fossils.
When we talk about the “when” and “how fast” (or slow) of dinosaur evolution, we have to think in terms of geologic time—millions of years. Paleontologists and geologists, however, don’t think about geologic time just in terms of numbers, but also in terms of a relative time scale. In a relative time scale, the goal is simply to determine whether one event occurred before (is older than) or after (is younger than) another. The statement of the age of an event is thus an age relative to the age of another event. For example, Stegosaurus lived before, or is geologically older than, Tyrannosaurus. This is a statement of the relative geologic age of these two dinosaurs.
This contrasts with a numerical time scale in which the goal is to assign a numerical age, usually expressed in millions of years, to an event. In a numerical time scale, we say Stegosaurus lived 150 million years ago, whereas Tyrannosaurus lived 67 million years ago. It might seem simplest and most precise to assign numerical ages to all the events in the age of dinosaurs. But the fact is that this is not possible, although most events in dinosaur evolution can be dated to within about 5 million years. Because of this general inability to assign more precise numerical ages, paleontologists use a relative geologic time scale to discuss the age of a dinosaur or an event involving dinosaurs.
The relative geologic time scale is a hierarchy of names applied to intervals of geologic time (
figure 3.11). These names resemble the names of the months of the year or the days of the week. In a given week, we know Thursday comes after Tuesday, and, in a given year, we know March follows February. The names and their succession on the relative geologic time scale similarly allow paleontologists to be certain that the Cretaceous followed the Jurassic and that the Triassic came before the Cenozoic.
FIGURE 3.11
This geologic time scale shows both the major divisions of relative geologic time and the approximate numerical ages of the division boundaries. (Drawing by Network Graphics)
The construction of a relative geologic time scale by geologists and paleontologists was heavily rooted in two principles. The first, the
principle of superposition, states that in layered rocks (strata), the oldest rocks are at the bottom and younger layers are on top. The second, the
principle of biostratigraphic correlation, states that rocks that contain the same types of fossil are of the same age (
box 3.2). This principle is one of the basic ideas behind
biostratigraphy, the identification and organization of strata based on their fossil content and the use of fossils in stratigraphic correlation. Stratigraphic correlation is the process of determining the equivalence of age or position of strata in different areas. It is one of the fundamental goals of
stratigraphy, the study of layered rocks.
Geologists and paleontologists long ago realized that much of the vast thickness of
strata exposed on Earth’s surface is full of fossils, many of which are present only in specific layers and are thus considered to represent organisms distinctive of a particular interval of geologic time. The study of strata and fossils began in western Europe during the late eighteenth century, so many of the names used in the relative geologic time scale were coined by European geologists and based on European rocks and fossils (see
figure 3.11).
One useful aspect of the time scale is that it is a hierarchy of time intervals from long to short. For the purposes of this book, the longest intervals used are the eras. During the past 542 million years, there were three eras: the Paleozoic (ancient life), Mesozoic (intermediate life), and Cenozoic (recent life). The boundaries of these eras coincide with major extinctions, such as the Permo-Triassic extinction, which eliminated many characteristic Paleozoic organisms, and the terminal Cretaceous, or Cretaceous–Tertiary extinction, which eliminated the dinosaurs and some other characteristic Mesozoic organisms. Because dinosaurs lived only during the Mesozoic Era, we need concern ourselves here with just the three geologic periods of the Mesozoic: the Triassic, Jurassic, and Cretaceous.
German geologist Friedrich August von Alberti (1795–1878) coined the term “Triassic” in 1834. In studying the salt deposits of Germany, Alberti found three different rock sequences, an older one dominated by sandstone, an intermediate one mostly of limestone, and a younger one mostly of shale. All three sequences were younger than rocks identified as Permian but older than those identified as Jurassic. Thus, Alberti established a distinct time interval between the Permian and Jurassic Periods and called it the Triassic for the three rock sequences he had noted (triad being Latin for “three”). The Triassic is itself subdivided into three time intervals: Early, Middle, and Late Triassic. Geologists, however, refer to Triassic rocks as being Lower, Middle, or Upper Triassic. This convention—using “Lower” and “Upper” for rocks, but “Early” and “Late” for time (“Middle” is used for both)—is applied to all portions of the rock record and geologic time.
Today, Tanzania in eastern Africa, Portugal in western Europe, and the western United States are many thousands of kilometers apart. But, during the Late Jurassic, when dinosaurs lived in these three areas, they were somewhat closer together because of continental drift, though still thousands of kilometers apart. In the western United States—especially Wyoming, Colorado, Utah, and Oklahoma—Late Jurassic dinosaur fossils are now found in a sequence of rocks called the Morrison Formation. In Portugal, the Lourinhã and Alcobaça formations form a rock sequence that contains some of the same kinds of dinosaur fossils. In Tanzania, similar dinosaurs have been collected from a different sequence of rocks geologists call the Tendaguru Series. It is mostly because of the similarity of the dinosaur fossils found in the Morrison Formation, the Portuguese rock formations, and the Tendaguru Series that paleontologists believe all three rock sequences are of approximately the same age. The dinosaur fossils provide an important biostratigraphic correlation of three rock sequences widely separated geographically, then and now (
box figure 3.2).
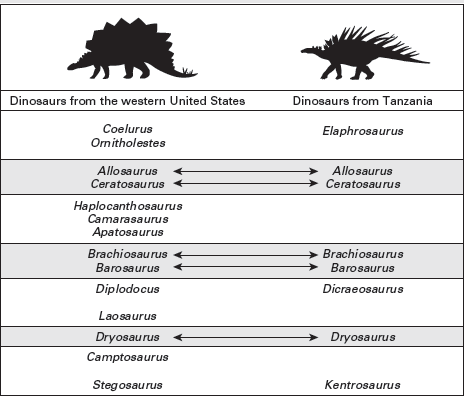
BOX FIGURE 3.2
Dinosaur fossils from rocks as far apart as the western United States and Tanzania are similar and support the correlation of these rocks. (Drawing by Network Graphics)
Dinosaur fossils from the Morrison Formation are among the most familiar to the American public: “Brontosaurus” (properly called Apatosaurus), Diplodocus, Brachiosaurus, Allosaurus, and Stegosaurus. These and other Morrison Formation fossils were first collected by American paleontologists during the late nineteenth century. Imagine the surprise of many paleontologists when some of the same types of dinosaurs, such as Brachiosaurus and Allosaurus, were discovered in the Tendaguru Series in eastern Africa by German paleontological expeditions just before the First World War. More recently, four of the dinosaur genera known from the Morrison Formation have been identified in Portugal: Allosaurus, Apatosaurus, Ceratosaurus, and Torvosaurus. According to the principle of biostratigraphic correlation, this means that the dinosaur-bearing sequences from the western United States, Portugal, and Tanzania are of the same relative age.
The Tendagaru Series, however, has additional importance to the correlation of these dinosaur faunas. This is because the dinosaur-bearing rocks in Tanzania were deposited along the shore of a Late Jurassic sea, and some of the dinosaur bones from the Tendaguru Series have the fossils of marine clams attached to them. Apparently, the clams grew on these bones in the shoreline lagoons and estuaries after the dinosaurs died. These clams are considered Late Jurassic in age because they are the same types as clams found in other marine rocks of well-accepted Late Jurassic age. So, we can now say that the same types of dinosaurs lived in the western United States, Portugal, and eastern Africa during the Late Jurassic when certain types of clams lived in the sea. These discoveries have given paleontologists a more complete picture of the Late Jurassic world, on the land and in the sea.
Today, rocks of Triassic age are recognized worldwide. The Triassic Period lasted 50 million years, from 201 to 251 million years ago (see
figure 3.11). Dinosaurs did not appear until the Late Triassic, about 225 to 230 million years ago. They appeared at almost exactly the same time as the first turtles, crocodiles, pterosaurs (flying reptiles), plesiosaurs (long-necked marine reptiles), and mammals.
Many of us know Alexander von Humboldt (1769–1859) as a famous explorer and geographer of the late eighteenth century. But von Humboldt was also a trained geologist who, in 1799, first used the name “Jura” for a distinctive limestone found in the Jura Mountains of Switzerland. This became the basis for the word “Jurassic,” used by geologists to refer to the time period between the Triassic and the Cretaceous, 145 to 201 million years ago (see
figure 3.11).
Like the Triassic, the Jurassic is divided into Early, Middle, and Late intervals of time and Lower, Middle, and Upper rock intervals. Dinosaurs flourished everywhere on Earth during the Jurassic and, judging by their abundance and diversity, were extremely successful.
In parts of western Europe, especially in Great Britain, France, and Belgium, rocks that are younger than Jurassic and older than Tertiary are mostly chalk. For this reason, a Belgian geologist, Jean-Baptiste-Julien D’Omalius d’Halloy (1783–1875) used the French term Terrain Crétacé (Cretaceous System) in 1822 to refer to these rocks (creta being Latin for “chalk”).
The Cretaceous Period is the interval of geologic time between the Jurassic and Tertiary, 66 to 145 million years ago (see
figure 3.11). It has traditionally been divided only into Early and Late time intervals, but, during the past 30 years, a Middle Cretaceous time interval has also been distinguished by some geologists. Dinosaurs are known from rocks of Cretaceous age on all the world’s continents. They became extinct at the end of the Cretaceous.
The geologic time scale used here (see
figure 3.11) includes not just the names of the eras, periods, and epochs, but also assigns numerical ages, in millions of years, to their boundaries. How are these numerical ages calculated?
Before the discovery of radioactivity, calculating numerical ages for events in geologic history was little more than guesswork. But radioactivity, the spontaneous decay or falling apart of some types of atoms, provides a natural clock for estimating numerical geologic ages, because the rate of decay is constant for a given type of atom (
figure 3.12). So, geologists can use this
radioactive clock to determine the numerical age of rocks, provided that the rate of decay is known for the radioactive atoms and that the rock contains a known quantity of such atoms.
In laboratories, geochemists have calculated with great precision the rates at which radioactive atoms decay. Some of these rates are so slow that the decay products can be measured to estimate ages as old as billions of years. However, the rate of decay of some radioactive atoms—carbon-14 is a good example—is too fast to be used to estimate ages older than a few tens of thousands of years. In contrast, the rate of decay of uranium-238 is so slow that it takes 4.5 billion years for half the uranium atoms in a sample of uranium to decay. Calculating the amount of decay of uranium atoms by measuring the product of the decay, which is lead-206, in a rock from the age of dinosaurs involves measuring a very small amount. Avoiding errors in measurement is difficult; sometimes mistakes are made, and incorrect numerical ages are calculated.
These problems, however, do not prevent the best laboratories from calculating accurate numerical ages. Instead, what poses the largest problem for a numerical time scale is the fact that most rocks, indeed nearly all sedimentary rocks, do not contain enough decaying radioactive atoms to accurately determine age. Sufficient quantities of these types of atoms are found almost exclusively in igneous rocks. However, because igneous rocks typically do not contain fossils, the usual way to calculate a numerical age for a fossil is to find an igneous rock layer close to the sedimentary rock that does contain fossils. In ideal circumstances, there are sheets of lava above and below a layer of sedimentary rock containing dinosaur fossils, which yield numerical ages bracketing the age of the fossils (
figure 3.13). But, this does not happen often, and paleontologists can only estimate the numerical age of a dinosaur fossil by evaluating its proximity to the nearest rocks yielding a numerical age.
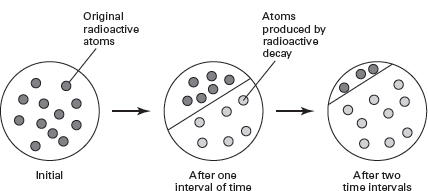
FIGURE 3.12
The slow decay of radioactive atoms provides a radioactive clock for measuring geologic time in millions of years. (Drawing by Network Graphics)
Such evaluation relies on several lines of evidence used in stratigraphic correlation. But, the fact remains that we cannot assign precise numerical ages to all dinosaur fossils, and we may never be able to do so. This is why we continue to use the divisions of the relative geologic time scale, supplemented by the best numerical-age estimates available, when discussing the “when” and “how fast” of the age of dinosaurs and their evolution.
FIGURE 3.13
A numerical age from a volcanic rock only approximates the age of dinosaur fossils found in nearby sediments. In this example, the volcanic rock yields a numerical age of 100 million years. Because the volcanic rock is above the layer with the dinosaur bone, the principle of superposition indicates the bones must be older than 100 million years. (© Scott Hartman)
Every year, thousands of dinosaur fossils are discovered and collected worldwide. Here’s how to find a dinosaur fossil:
1. Dinosaurs lived only during the Mesozoic Era, from the Late Triassic to the end of the Cretaceous. Limit your search to rocks that formed during that geologic time interval.
2. Dinosaurs lived on land, so almost all of their fossils are found in sedimentary rocks deposited in fluvial, lacustrine, or deltaic sedimentary environments. Focus on such rocks.
3. Dinosaur fossils can be discovered only once they begin to erode from rock on the land surface. So, find places where rock is actively eroding, not places where rock is covered by soil and vegetation. Badlands are ideal.
4. You need a search image, which means you need to know what a dinosaur fossil—bone, tooth, or footprint, for example—looks like. Look at photographs or go to a museum and familiarize yourself with dinosaur fossils before you begin your search.
5. Hike and search. At this point, luck may also be needed to help you find that rare place where a dinosaur fossil is eroding from the rock.
If you reach that place, then you will discover a dinosaur fossil (
box figure 3.3). You then become the first human to see this dinosaur since it became entombed in rock, many millions of years ago!
BOX FIGURE 3.3
You may find a dinosaur fossil like this one—the ulna of a Late Cretaceous ceratopsian.
Dinosaur fossils are present in Mesozoic sedimentary rocks formed in nonmarine environments. So, the search for dinosaur fossils focuses on these rocks (
box 3.3). Paleontologists will examine such rocks, relying in part on the locations of previously discovered dinosaur fossils and in part on sheer luck, to find dinosaur fossils.
Once a dinosaur fossil is found, if it is small enough and sufficiently solid it may be collected by simply picking it up (if it is on the surface) or digging it right out of the rock. But most dinosaur fossils are so large and/or fragile that they must be encased in a plaster jacket before being collected. This is done by carefully digging around the fossil (
figure 3.14), covering it with paper (so the plaster does not adhere directly to the fossil), and then wrapping the fossil with strips of burlap soaked in wet plaster. When the wet plaster hardens, it forms a solid case that protects the fossil from damage during transport to the laboratory.
FIGURE 3.14
Excavating a dinosaur takes much care, patience, and hard work.
In the laboratory, technicians remove the plaster jacket and surrounding rock from the dinosaur fossil. This process of preparation has to be undertaken with great care and precision so as not to damage the fossil. Often the cleaned dinosaur fossil needs further stabilization and is coated with shellac-like hardeners. Complete preparation of a dinosaur fossil may take months or years!
After preparation, the dinosaur fossil is ready for scientific study and display. Paleontologists and technicians at museums and universities worldwide undertake the collecting, preparation, and study of dinosaur fossils.
1. Fossils are evidence of past life. Dinosaur fossils are not only bones, but also footprints, eggs, skin impressions, tooth marks, stomach stones, and feces.
2. Dinosaur bones were almost always mineralized as they were fossilized, a process believed to take at least 10,000 years.
3. Taphonomy is the study of the processes that intervened between the life of a dinosaur and the fossils of the dinosaur we collect and study. Taphonomy particularly concerns the information lost via these processes.
4. Dinosaur fossils are preserved almost exclusively in sedimentary rocks formed on the continents by rivers, lakes, and deltas.
5. Geologic time is measured by two time scales: a relative one and a numerical one.
6. On the relative time scale, dinosaurs lived during the Mesozoic Era, from the Late Triassic Period through the entire Jurassic and Cretaceous Periods, until their extinction at the end of the Cretaceous.
7. On the numerical time scale, dinosaurs lived about 66 to 225 or 230 million years ago.
8. The numerical time scale is based primarily on the decay of radioactive atoms found in sufficient quantity, with few exceptions, in igneous rocks.
9. Because dinosaur fossils are not found in igneous rocks, numerical ages for dinosaurs are estimates with varying degrees of accuracy. This is why most statements about the ages of dinosaurs employ the relative time scale.
10. Collecting and preparing dinosaur fossils is a complex process that can take months or years.
biostratigraphy
body fossil
calcium phosphate
Cenozoic Era
coprolite
Cretaceous Period
deltaic
eolian
floodplain
fluvial
fossil
gastrolith
igneous rock
Jurassic Period
lacustrine
marine
Mesozoic Era
metamorphic rock
mineralization
numerical time scale
Paleozoic Era
principle of biostratigraphic correlation
principle of superposition
relative time scale
sedimentary (depositional) environment
sedimentary rock
sedimentologist
sedimentology
strata
stratigraphy
taphonomy
trace fossil
Triassic Period
1. What types of dinosaur fossils can paleontologists collect and study?
2. How does bone fossilize? How long might fossilization take?
3. What taphonomic processes intervene between the time a dinosaur is alive and when its remains have fossilized? What effect do these processes have on the information available to paleontologists?
4. Which types of rock and which types of sedimentary environment contain most dinosaur fossils? Why are few fossils found in igneous and metamorphic rocks?
5. Compare and contrast the relative and the numerical geologic time scales.
6. Why can’t we assign precise numerical ages to all dinosaur fossils?
7. When, in terms of the relative and the numerical time scales, did dinosaurs live?
Berry, W. B. N. 1987. Growth of a Prehistoric Time Scale. Palo Alto, Calif.: Blackwell Scientific. (Reviews the history of the relative geologic time scale and the principles behind it)
Gradstein, F. M., J. G. Ogg, M. D. Schmitz, and G. M. Ogg. 2012. The Geologic Time Scale 2012. 2 vols. Amsterdam: Elsevier. (Recent grand synthesis of the geologic time scale)
Martin, R. E. 1999. Taphonomy: A Process Approach. Cambridge: Cambridge University Press. (A comprehensive review of the theory and practice of taphonomy)
Prothero, D. R., and F. Schwab. 1990. Sedimentary Geology. New York: Freeman. (Textbook on stratigraphic principles and sedimentary environments)
Stanley, S. M., and J. A. Luczaj. 1989. Earth System History. New York: Freeman. (Textbook that provides an overview of fossils and the history of life)