Chapter 7
Formation Pathways in Vinification
7.1 Introduction
Flavour components in fruit and vegetables, and in their derived beverages, are produced biogenetically and/or by thermal processing. The developing flavours and aromas towards maturity result from biogenesis by the action of endogenous enzymes on non-volatile precursors in intact tissue. Biogenesis of new flavours in fruit and vegetables occurs by exogenous enzymes entering damaged tissues and their juices, as in fermentation to produce alcoholic beverages (notably wine) from the grape. These enzymes are mostly present in the micro-organisms (yeasts, moulds and bacteria) that can enter, some causing ‘decayed’ or ‘spoilage’ flavours. The original flavours from the fruit may or may not persist through a fermentation.
Other beverages, such as coffee brews, derive their flavour/aroma largely from roasting or thermal processes on the raw material, which make use of the well known Maillard reaction between amino acids (from proteins) and reducing sugars (like glucose and fructose). These non-enzymatic processes produce both new non-volatile substances, responsible for taste and new volatile substances that are responsible for aroma. The final beverage will also contain unchanged non-volatile and volatile substances from the original raw material, such as green coffee beans. Other chemical reactions may also occur.
The Maillard reaction is generally considered only to occur with any significant rate above 50°C. The contribution of this reaction to the flavour of most table wines, produced largely by fermentation, is therefore very small. In some wines (especially Madeira and thermo-vinified wine), there will be evidence of Maillard-type reaction products. Maturation (ageing) of wines taking substantial periods of time will also give additional flavour changes by chemical reactions, such as the production of furans and furanones from wooden barrels that can have been ‘charred’. Yeasts will then no longer play a rôle (except when matured sur lie), but airborne bacteria may be active and others introduced. Both Jackson (2008) and Ribéreau-Gayon et al. (2006) have provided very detailed accounts of all stages in vinification and should be consulted for further information.
In considering the formation pathways for flavour components in wine, it is convenient to examine first the process variables in the fermentation stage of vinification. These are (1) the raw materials, i.e. the grapes; (2) strain of yeast used; (3) temperature; (4) maceration; (5) clarification procedures; and (6) the nutrient medium. These descriptions will not cover all the flavour effects found in practice, so that it is also convenient to consider briefly the source of each group of flavours separately. The effects of various process variables have been closely studied over the decades and continue as a normal exercise in any craft but such studies have also been carried out in a rigorous academic manner in research institutes.
Over the last decade more studies have been done on the role of yeasts and their interaction with the nutrients in the must during fermentation and the effect on the aroma content and overall wine quality and adds to our current understanding (for reviews see Swiegers & Pretorius, 2005; Swiegers et al., 2005; Ugliano et al., 2007; Fleet, 2008; Ugliano & Henschke, 2009). Some of this information is discussed below. The reviews give detailed information regarding the formation biochemical pathways, enzymes involved and even the genetic information coding for the enzymes on the yeast, although this information is not yet available for all compounds.
The biochemical pathways of the flavour formation in grapes is also becoming a more prominent subject of study and the grapevine genome has now been sequenced (see Palaskova et al., 2008). So far only limited information has been published regarding grape terpene biosynthesis and carotenoid breakdown leading to the formation of β-ionone.
Once the volatile composition can be matched to sensory perception and it is known how grapes, yeast and fermentation conditions affect the formed aroma profile of the wine, wine makers may have the knowledge to plan in advance the properties they want their wine to have and make the wine accordingly!
7.2 Process variables in vinification
7.2.1 Grapes
As already described, a wide range of grape varieties/cultivars is available and used for wine-making. There are known differences of composition, especially of varietal aroma (see Chapter 4), although other differences in precursors for flavour are not generally well delineated. Considerable information is available on the chemical composition related to grape maturity and harvesting conditions. The content and proportions of free amino acids (present in grapes/musts, 1–4 g L−1), like proline and others, can differ markedly from one variety to another and will therefore be responsible for some differences found in volatile compound content, from their breakdown during fermentation. In a Doctoral thesis by Milley (1988) quoted by Ribéreau-Gayon et al. (2006), the content of free amino acids in three different grape varieties used for Champagne (Chardonnay, Pinot Noir and Pinot Meunier) is given at four different stages of ripening (September/October). Chardonnay is particularly characterized by a high proline content. Sulfur-containing amino acids, such as cysteine and methionine, are of general potential interest, since they are precursors of highly odoriferous sulfur compounds in wines. Numerous examples of amino acids as precursors will be noted in Section 7.4.
Figure 7.1 (Approximate) Location of extractable chemical compounds in wine grapes (red and white) during vinification.
Note: See Chapters 3, 4 and 5 for detailed information on contents, including trace elements and minerals.
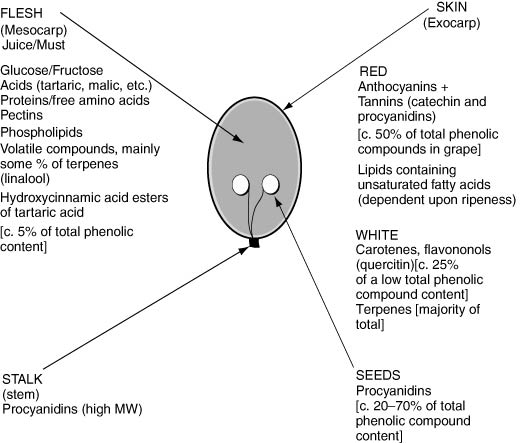
The location of the different chemical compounds in the actual wine grape (both red and white) is important in vinification, determining the type of many of the process stages used. A guide to their location is illustrated in Figure 7.1.
The maturity of the grape at harvest will determine the composition and as a result the potential aroma and quality of the wine. Traditionally the sugar–acid ratio has served as an indicator of ripeness. However, there is more interest in optimum maturity to gain the best wine quality of the grapes. A study in the volatile composition of Cabernet Sauvignon grapes during ripening showed the evolution of these compounds; terpenes characterized early berry development, whereas at later stages, aldehydes followed by alcohols tended to dominate (Kalua & Boss, 2009). The authors suggested that alcohol to aldehyde ratio may possibly be used to predict the picking date for optimum grape and wine aroma. Another study to predict the flavour potential of grapes used acid and enzymic hydrolysis, the former method showing some potential (Loscos et al., 2009).
7.2.2 Yeast strain
Yeast is the primary agent of change in grape ‘must’ to make wine, as already described briefly in Chapter 1. Several kinds of yeast may be involved in vinification but the most important is Saccharomyces (genus) cerevisiae (species). Some confusion on relevant species still arises, which is not entirely clarified by taxonomic evidence. The Saccharomyces genus has been divided according to cell shape, usually spherical to ellipsoidal but may be cylindrical or even apiculate, so that wine yeasts were once characterized as being Saccharomyces ellipisoideus.
Some oxygen is required for yeast to synthesize sterols and long-chain fatty acids, needed for the cell membranes of the yeast. Normal grape handling during de-stemming and crushing usually allows the must to absorb sufficient oxygen (see Chapter 3) for yeast growth. Fermentation by the yeasts does not require oxygen. Most yeasts grow, as is happening in a grape must, by vegetative reproduction; and wine yeasts grow fairly well under anaerobic conditions but with a lower yield. Saccharomyces ellipsoideus in vinification is now classified as Saccharomyces with cerevisiae as the correct species name; but there will be (not clearly delineated) varieties or strains of this species according to source/method of manufacture. There are also several other known species such as S. bayanus, S. chevalieri, S. italicus and S. heterogenicus but experimental data in a Doctoral thesis (Soufleros, 1978), quoted by Ribéreau-Gayon (1978), has indicated that an identical amount of the total higher alcohol and total fatty acid esters is formed under identical fermentation conditions from these species. However, other species such as S. baillii were shown in the same data to give substantially lower amounts. S. cerevisiae is also used in the brewing of beer, particularly ales, which are bottom fermented, but S. uranum is deliberately used in the manufacture of lagers, since it liberates significantly larger amounts of octanoic and decanoic acids during fermentation, providing a distinctive flavour. S. apiculatus (lemon-shaped) has been reported to give a higher quantity of esters/amyl alcohol, and S. oviformis to give aldehydes and ethyl propanoate in particular. The effects of yeast strain on important volatile compounds for flavour have been discussed by a number of workers, especially by Soles et al. (1982) in relation to the differences in ester production.
Much research has been focused on the effect of yeast on aroma production and its impact on wine quality, comprehensively reviewed by Bisson & Karpel (2010) and numerous reviews therein (Ugliano & Henschke, 2009; Swiegers & Pretorius, 2005). Of course, it has been known for some time that many volatile compounds are produced by yeasts during fermentation, one of the reasons wine has such a different aroma from grape juice. The flavours formed during the yeast fermentation depend on numerous factors (cultivar, ripeness, fermentation temperature and many processing parameters), including the yeast population. With the availability of more accurate methods of aroma analyses as well as techniques to determine the genetic differences between yeasts, this has become a fruitful area of research. Bisson & Karpel (2010) listed a total of eight alcohols, nine acids, 15 esters, three carbonyls, ten sulfur and four thiol compounds being formed during the fermentation (Table 7.1).
Table 7.1 Volatile compounds formed during Saccharomyces fermentation.
Compound class | Compounds |
Alcohol | ethanol, propanol, butanol, 2-methylpropanol, 2-methylbutanol, 3-methylbutanol, hexanol, phenylethanol |
Acid | acetate, propanoate, butyrate, 2-methylpropanoate, 2-methylbutanoate, 3- methylbutanoate, hexanoate, octanoate, decanoate |
Ethyl ester | ethylbutyrate, ethyl hydroxybutyrate, ethyl hexanoate, ethyl octanoate, ethyl decanoate, ethyl propanoate, ethyl 2-methylpropanoate, ethyl 2-methylbutyrate, ethyl 3-methylbutyrate, |
Acetate ester | ethyl acetate, hexyl acetate, 2-methylpropylacetate, 2-methylbutylacetate, 3-methylbutylacetate, phenethylacetate |
Carbonyl | acetaldehyde, diacetyl, acetoin |
Sulfur compounds | hydrogen sulfide, methanethiol, ethanethiol, dimethylsulfide, dimethyldisulfide, dimethyltrisulfide, diethylsulfide, diethyldisulfide, methionol, methional |
Thiol | 4-mercapto-4-methylpetan 2-one, 3-mercaptohexan-1-ol, 3-mercaptohexylacetate, furfurylthiol |
Information from Bisson & Karpel (2010).
Fusel alcohols, esters and sulfur-containing volatiles are commonly present above their threshold values and will influence wine flavour. The sulfur compounds can easily have a negative impact on wine flavour (see Table 4.28). In many cases the precursors as well as the genetic codes for the enzymes involved in the formation have been identified, although this work is by no means complete. For example a recent study on yeast involved in the release of 4-mercapto-4-methyl pentan-2-one (4-MMP) elucidated the four genes and enzymes involved in the release of this volatile thiol during the fermentation (Howell et al., 2005).
For the choice of yeast to have a dramatic impact on the resulting wine quality, impact aroma compounds would need to be affected, rather than the general fruity and floral compounds contributing generally to wine flavour. Also the precursors need to be present in sufficient concentration in order for the yeast to synthesize, liberate, or convert them into aroma compounds, so processing should be adapted to control the flavour formation. There appears to be a great diversity in the flavour impact genes of Saccharomyces, which may in future be used to select the yeast able to enhance the desired flavours in a particular wine. New recombinant yeast strains may well give more options to the wine maker than currently available.
Wine fermentation has been traditionally associated with ‘natural’ yeasts present on the surface of grapes and indeed present generally in winery premises, which in the case of red wine production are adequately present, though said to be often less so for white wines. Whilst Saccharomyces ellipsoideus will, however, be the predominant species, there will be others, including ‘wild’ strains and such yeasts as Kloeckera spp., Torulopsis and Hansenula present on the surface of grapes. However, as fermentation proceeds, S. cerevisiae will be the dominant active fermenting yeast. Kloeckera spp., for example, are inhibited at alcohol levels above 3–4%, and most other wild yeasts species are either slow growing or are inhibited by sulfur dioxide and by alcohol or the absence of oxygen. Other yeasts and parasitic fungi, such as Candida and Botrytis, can occur on diseased and damaged grapes, which again are overwhelmed by the growth of the fermenting yeasts. Acetic acid bacteria will also be found in damaged and diseased fruit. Hansenula and Pichia are associated with surfaces of wineries in addition to other yeasts, though hygienic operation in modern wineries lowers their significance.
There is a modern tendency associated with a definite emphasis on hygiene generally throughout a winery, for purposeful inoculation of the grape must with outside manufactured active dry S. cerevisiae based on laboratory strains (which, however, may not be totally pure), with white wines and to a lesser extent with red wines. It is certainly required after the vinification, such as for the production of sparkling wines, and is essential when pasteurized must is used. This subject causes considerable discussion and controversy, drawing parallels with the slightly different and often preferred taste of cheeses like Brie, when a choice can be made from non-pasteurized or compulsorily pasteurized milk (Barr, 1988). Potentially bland and uniform wines are thought to be produced when only cultured yeasts are used. Those in favour of using inoculum like the rapid onset of fermentation, reducing the risk of the growth of less desirable organisms, potentially giving off-flavours.
There are documented differences between wines depending whether cultured or wild yeasts were used for fermentation. A comparison of Chardonnay wines allowed to ferment with indigenous wild yeast and Chardonnay wines made by fermenting with an inoculated strain of S. cerevisiae showed a greater variability in flavour compounds in the wines fermented with wild yeasts; and higher concentrations of 2-methylpropanol, 2-methylbutanoic acid, ethyl 2-methylpropanoate, ethyl decanoate, and ethyl dodecanoate were considered the most significant differences contributing to the flavour of wines (Varela et al., 2009).
Different yeasts (species and strains) will contain the same enzymes but their relative proportions and catalytic activity may well vary, so providing some of the differences noted for the same environment. During fermentation on a sugar substrate, yeasts are growing and reproducing, where they can do so asexually by budding and sexually by the formation of spores, though the latter seldom occurs in must. The chemical composition of yeasts is of relevance. The yeast cell is surrounded by a strong and mechanically refractory cell wall, which may account for 20–30% of the cell solids, consisting of glucans and glycoproteins. The cell wall surrounds the plasma membrane, which regulates the transport of chemical substances into and out of the cell. S. cerevisiae contains some 45% estimated protein on a dry basis and a number of vitamin substances in small amounts.
Spoilage yeasts such as Brettanomyces, if not controlled, can lead to the undesirable flavours of ethyl phenols (Section 7.4.9).
7.2.3 Malo-lactic organisms
Another type of fermentation to be considered in any overall vinification is the malo-lactic acid conversion. The organism for this type of fermentation is Leucanostoc oenos, which grows after alcoholic fermentation, as also discussed in Chapter 1. Malo-lactic fermentation of model systems with grape flavour precursors using Oenococcus oeni, Lactobacillus brevis and Lactobacillus casei showed that these organisms released small amounts of terpenes, norisoprenoids, volatile phenols and vanillins into the model system (Hernandez-Orte et al., 2009). Quantitative differences were tentatively attributed to the nature of the enzymes of the malo-lactic organisms. The enzymes were able to affect volatile changes even when the organisms failed to carry out the malo-lactic fermentation.
7.2.4 Temperature
The temperature at which the fermentation should be conducted for optimal effect has been studied by numerous investigators, past and present in different locations, both in respect of alcohol production and for volatile compound formation (esters and higher alcohols, in particular) and also for the extraction of phenolic compounds from the grape skins.
Standard operating temperature
A controlled fermentation temperature is regarded as highly important for white wines and it is generally assumed to be kept on the cool side, i.e. 15–17°C. For red wines a higher temperature is generally selected, ca. 25°C. In red wine production, a shorter hotter fermentation extracts more phenolic compounds (from the skins), whilst a longer cooler one produces more aromatic substances (esters). Barr (1988) comments that a red wine intended to be drunk young, i.e. without containing too many tannin substances, is fermented at 25°C, whilst Peynaud (1986) reports that, for a fine wine, the ideal fermentation temperature should be no more than 30°C, irrespective of grape variety. Ribéreau-Gayon (1978), on the other hand, comments that in the production of red wines the fermentation should be at considerably higher than 30°C. At some Châteaux it is claimed higher temperatures are actually used, e.g. 32–34°C.
Ribéreau-Gayon (1978) quotes some interesting data from a Doctoral thesis of a pupil (Soufleros, 1978). For example, at 20°C, and a normal pH of 3.4, 10.8 mg L−1 of total fatty esters were generated compared with 7.8 mg L−1 at 30°C. With a pH of 2.9, even lower ester contents were recorded at the same temperature.
Robinson (1995) and Jackson (2008) comment that wine makers in the New World vinify white wine at the lower end of the 12–17°C range, so that the tropical fruit flavours (like pineapple) are especially generated. Conversely, Europeans tend to ferment Chardonnay at 18–20°C. The effect of temperature has also been studied by Ough et al. (1979, 1986), in particular in respect of the generation of isoamyl (pentyl) acetate (fruity/pears, bananas) and of 2-phenyl ethyl acetate (fruity/apricot). These authors show a higher content for isoamyl acetate at 13°C than at 30°C; the formation of 2-phenyl ethyl acetate peaks at 20°C. Other esters, such as ethyl octanoate and decanoate (the oily/soapy flavours) are produced optimally at 13–15°C. As described under maturation in Chapter 4, the final ester composition of a wine, particularly white, will be determined by the period and type of ageing practiced, due to changes in the ester composition during ageing. White wines drunk very young will have a dominant ester flavour resulting from low temperature fermentation.
Fermentation temperature has been shown also to influence the content of higher alcohols (fusel oils) in the work of Soufleros (1978) already quoted. Higher temperatures, in conjunction with lower pH values, gives a markedly lower fusel oil content.
Fermentation temperature is clearly important for red wines (and also white) since it influences the required extraction of phenolic substances, though this will also be largely determined by grape skin contact and variety of grape chosen. Most popular wine books (Clarke & Rand, 2001; Robinson, 1995) indicate fermentation temperatures for red wines to be 25–30°C; however, there is a trend to the use of ‘cool’ fermentation for red wines, in Australia, to gain a ‘fruity’ red wine to be drunk young.
Thermovinification
Thermovinification is a process that can be used for red wines to increase the contents of some phenolic compounds and of some volatile compounds during subsequent conventional fermentation. It consists of taking de-stemmed grapes and heating them up to temperatures in the range 60–87°C to disrupt the cell structure and facilitate subsequent extraction, especially of anthocyanins from the skins. The holding time of the heated pulp varies between two minutes at 87°C to several hours at lower temperatures; lower temperatures can only be used for sound, non-mouldy fruit, since the enzyme laccase remains active even at 60°C. The pulp is then pressed and once the juice has cooled to 25°C the yeasts are added and fermented without skins and seeds.
The process has been investigated in detail by Fischer et al. (2000) in Germany and the results statistically compared with those of standard tank vinification procedures conducted at the same time. The standard procedure had three sub-variants of operation in respect of handling/submerging the grape skins during fermentation; and three different red wine grape varieties, Pinot Noir, and two cultivars (Domfelder and Portugieser) specific to Germany. There were a number of interesting conclusions drawn; thus, the use of thermovinification very markedly increases the amount of certain esters, in particular 3-methyl-1-butyl (isoamyl) acetate (see above) and hexyl acetate, though the results and their interpretation were not always clear. There were important claimed differences in the extraction of certain phenolics between the different in-tank operations – thus quercetin (glycoside) content was some 2–8 times higher in wines vinified in ‘pump over’ tank than ‘punch down’. Differences in higher alcohol content and alkanoic acids were also shown. There were no sensory data, though based on the detailed chemical analysis the authors suggested that thermovinification would provide a more fruity style of red wine. The authors also said that thermovinification is mostly applied in cool climate viticulture to grape varieties of low anthocyanin concentration, yielding not only fruity red wine with strong colour but low tannin concentration. It is the method of choice for big wineries and co-operatives.
7.2.5 Clarification procedures
Ribéreau-Gayon (1978), quoting his own work of 1975, comments on the desirability of allowing the fermentation for white wines to take place in clarified juice or must. Clarification, for example, by racking, increased the production of total fatty acid esters significantly and lowered that of the higher alcohols. Must clarification by use of pectolytic enzymes can influence type of phenol content, as described in Section 7.4.9. Numerous clarification methods are available, all described in depth by Ribéreau Gayon et al. (2006).
7.2.6 Nutrient medium in fermentation
A correct composition of the nutrients in the medium for the growing yeast cells is important, especially in relation to nitrogenous components. A lack of nitrogen can lead to a ‘stuck’ fermentation. This may happen, for example, with clarified musts, where protein content has been lowered. An excess of nitrogen can, however, give much cell multiplication but a reduced conversion of sugar into alcohol.
The nitrogen in the must will depend on vineyard management, such as any nitrogen additions in the vineyard and of course the wine maker can make an addition of nitrogen to the must. It has become more apparent that the nitrogen available for yeast fermentation can significantly influence the non-volatile and volatile flavour components in wine, and for more detailed information the reader can refer to a number of reviews (Bell & Henschke, 2005; Ugliano et al., 2007; Ugliano & Henschke, 2009).
Nitrogen addition in the vineyard influenced berry composition and therefore may be expected to influence fermentation and the resulting wine. Generally, increases in esters are reported with use of nitrogen in the vineyard, however, there is insufficient information in literature to understand how wine composition and quality may be affected. Nitrogen application in the vineyard does give an increase in total nitrogen compounds in grapes, such as amino acids. The amino acid content may well have more influence on the aroma profile of a wine than perviously assumed but there is insufficient information available.
Yeasts assimilate nitrogen and the types and concentration of nitrogen available in must regulates the growth and metabolism of the yeast, including the sugar, nitrogen and sulfur pathway, all influencing the aroma formation and hence having a possible impact on the sensory properties of the resulting wine. In many wine regions, the available nitrogen in the must is low, possible limiting yeast growth, fermentation rate and increasing the risk of a stuck fermentation. A shortage of nitrogen can give high concentrations of fusel alcohols and there is an increased risk of sulfur off-flavour formation. However, a recent study threw doubt on the benefit of adding nitrogen to to reduce the formation of sulfur compounds, since the final Shiraz wines thus made all had higher levels of volatile sulfur compounds, irrespective of the fermenting yeast used (Ugliano et al., 2009). Shiraz is a variety particularly prone to form high levels of volatile sulfur compounds and a reductive aroma character. Wine makers oxygenate their fermentations to help to counter this. An excess of nitrogen can give an estery off-flavour, and is a limiting composition of must and the formation of aroma compounds, affecting the aroma and quality of wine. Other factors of importance are yeast strain and fermentation conditions.
Vilanova et al. (2007) studied aroma compound formation in chemically defined wine like media. There were differences in formation of aroma compounds, attributed to the differences in pathway of formation. They concluded that the addition of nitrogen in the form of diammonium phosphate shows a peak of higher alcohols formed at about 200–300 mg L−1 addition, after which the amounts formed tailed off considerably. The production of fatty acids ethyl esters and acetate esters, compounds responsible for the fruity characteristics of wine, generally increased with greater additions of nitrogen. The concentration of branched esters, now thought to contribute red berry flavour to wine, tended to decrease with increasing nitrogen additions. These preliminary trials suggest that nitrogen additions to white wine juice may influence the aroma and sensory quality of the resulting white wine. Since red wines are made differently from white wines (see Chapter 1), with more of the flavour generally believed to be derived from maturation, there is much less information on the effect of nitrogen on red wines.
The sulfur metabolism of yeasts give a number of sulfur containing compounds, many unpleasant and both yeast strain and nutrient composition determine their formation. Vitamins, such as biotin and pantothenic acid will also influence volatile formation in must but the influence of other factors, such as metal ions and phosphate, is not documented.
7.2.7 Maceration
An important process variable in vinification is the contact time and type of the grape skins with the grape juice and flesh either in the course of the fermentation or before, the so-called maceration.
Maceration primarily affects the extraction of phenolic substances, affecting bitterness, astringency and colour, as already described in Chapters 1 and 3, and in the section on thermovinification in this chapter. The extraction of volatile compounds can also be affected by skin contact, in particular that of terpenes. Different techniques will be used on account of the differential location of these compounds in the juice, flesh, skins, and indeed in the seeds and stems, though both the last mentioned are often avoided for use and often removed at crushing. There is the effect of crushing and draining and, particularly in red wine, vinification, the various ways of maintaining contact of the skins with the fermenting juice. Terpenes tend to reside more in the skin of grapes than in their flesh or juice but their distribution varies markedly between varieties, including Muscadet, and the particular terpenoid composition also varies.
7.3 Production of ethyl alcohol
The major function of the yeast, Saccharomyces cervisiae, in wine fermentation is, of course, the production of ethyl alcohol (ethanol, C2H5OH) from the sugars (glucose and fructose) present in the juice. This yeast will also ferment any added sucrose but not other sugars like arabinose, rhamnose and xylose, which may also be present in small quantity in the must.
The particular enzyme present in the yeast has the general name zymase but in fact, yeast contains several enzymes, including invertase, which is necessary to split the sucrose into its component sugars (glucose and fructose).
The mechanism of the metabolic pathway from glucose and fructose to ethyl alcohol has been well established; the conversion proceeds primarily via the so-called Embden–Meyerhof glycolytic pathway oxidation to pyruvate, then to acetaldehyde and ethyl alcohol. For growth and reproduction, yeast cells require a steady supply of ATP (adenosine triphosphate) together with the reducing power of NADH (nicotinamide adenine dinucleotide). There are metabolic intermediates, which result in the noted formation of succinates, glycerol, acetoin, diacetyl (buta-2,3-dione), acetic and succinic acids (Amerine & Joslyn, 1970). This complex pathway can be understood in detail by referring to Jackson (2008) and, of course, to numerous other texts on biochemistry.
Notably, the production of alcohol during fermentation assists the physical extraction of numerous compounds (e.g. terpenes) from grape cells, which appear in the fermented wine.
7.4 Production of individual groups of compounds
7.4.1 Esters
The majority of esters are formed during fermentation, with the important variables of temperature and clarification affecting content and type of compound in white wine, as already noted. These syntheses are controlled enzymatically. The synthesis of acetate esters is fairly well documented and is linked to the lipid and acetyl-CoA metabolism. The esters formed vary with different yeast strains and the esterase content, a diverse group of enzymes, able to cleave and form ester bonds. The ester concentration in the young wine depends on its rate of formation during the fermentation but also on the amount released from the yeast into the wine. Ethyl acetate is usually present in the highest concentration and is formed in small amounts by yeast during fermentation, but also by acetic acid bacteria during ageing in oak.
Ethyl esters occur in lower concentrations and the formation of ethyl fatty acid esters is not well understood. Ethyl esters are secreted slower and less efficiently from the yeast into the fermentation than acetate esters.
There are numerous influences on the amounts of esters formed. An increase in grape maturity tends to give a lower formation of acetate esters during fermentation and a higher formation of ethyl esters. Grape solids are a source of lipids and affect yeast growth and a small quantity of solids during fermentation stimulates ester production. Factors affecting the ethyl ester formation during fermentation are temperature, with higher fermentation temperature resulting in ethyl octanoate and ethyl decanoate (Saerens et al., 2008). These authors suggested that genetic expression of the genes and enzymes involved in the biosynthesis of ethyl esters was not a limiting factor in their formation, the limiting factor is the availability of precursors in the must. An addition of medium chain fatty acids to the fermentation resulted in an increased formation of ethyl esters.
The effect of fermentation temperature has long been exploited, especially in the fermentation of white wines, which have fruity characters produced during low temperature fermentation. Lower fermentation temperature (15°C) tends to favour ethyl esters.
The ester concentration tends to increase with an increase in available nitrogen, although the there is no clear established link between individual esters and amino acids. Addition of ammonium salt before the fermentation enhances the ester production.
However, certain subsequent reactions occur non-enzymatically; thus in young wines the esters of low molecular weight acids (e.g. ethyl acetate) are generally in excess of their equilibrium constant. Hydrolysis back to the constituent alcohol and acid can then occur, favoured by high temperature and low pH. Other esters of higher molecular weight acids are present at low equilibrium constant levels so that, as noted, they tend to increase in amount on ageing, as for example with succinic esters. Low SO2 levels are stated to favour ester synthesis and retention, whilst intercellular grape fermentation (e.g. carbonic maceration) and the absence of oxygen during yeast fermentation further enhance ester formation. Acetic acid bacteria can synthesize ethyl acetate but acetic acid can also react non-enzymatically with ethanol to form ethyl acetate.
Another example of ester formation during maturation are the four esters (ethyl 2-, 3- and 4-methylpentanoate and ethyl cyclohexanoate) recently reported in a range of wines by Campo et al. (2007). They are formed during maturation by esterification reactions with ethanol and the corresponding acids formed by micro-organisms. Their concentrations vary, and are especially high in aged wines.
7.4.2 Aldehydes
Acetaldehyde, the main aldehydic constitutent, is an early metabolic product of fermentation but can then fall in amount; another source is the oxidation of ethyl alcohol by o-diphenols present (Appendix I) as the must is fermented to ‘dryness’. During fermentation acetaldehyde is an intermediate in the conversion from pyruvate to acetaldehyde and then ethanol. The acetaldehyde level generally drops to a low concentration at the end of the fermentation. Fermentation conditions influence the concentration of acetaldehyde, such as a higher fermentation temperature leads to a higher concentration.
Certain C6 aldehydes have been described as important such as hexanal and the hexenals, which are thought to result from enzymatic cleavage of oxidized linoleic and linolenic acids, respectively, as first explained by Drawert (1974). This occurs during crushing, when auto-oxidation of the small quantity of lipids (oils) in the grapes can occur. The C6 aldehydes are formed by enzymic oxidation of C18 unsaturated fatty acids. The corresponding alcohols may be also formed at the same time but subsequent fermentation will also entirely convert these aldehydes into alcohols (Appendix I.1.3). Saturated higher aldehydes are derived from the biosynthesis of fatty acids from the acetyl-CoA, and usually produced in trace amounts.
The amount of lipid substances in grapes is not precisely known though some data on component linoleic and linolenic acids is available from Roufat et al. (1987), referenced by Jackson (2008), showing a decline in ripening. This would support the contention of a ‘grassy’ flavour arising only from unripe grapes. Though some 10 mg of oxygen per litre of grapes (0.31 millimole) are believed to be taken up in crushing, it would be difficult to translate this figure into an expected unsaturated aldehyde content through stoichiometric equations and, of course, the growing microflora on crushed grapes would also be expected to take up oxygen.
The formation of several other aldehydes during ageing in-cask has already been discussed in Chapter 4. Extracted compounds during fermentation can also be changed by yeast enzymes, also referred to in Chapter 4.
7.4.3 Ketones
Ketones are generally produced during fermentation, including diacetyl, though the latter may be more likely produced through the action of spoilage lactic acid bacteria, or by lactic acid bacteria carrying out the malo-lactic fermentation. Diacetyl formation in wine depends on a great many factors, reviewed by Bartowsky & Henschke (2004). At the completion of the yeast fermentation, the concentration is typically less than 1 mg L−1, and will have little impact on the wine aroma (see Chapter 4).
The malo-lactic fermentation forms the main quantity of diacetyl, depending on strain. Generally, Pediocuccus and Lactobacillus produce high concentrations of diacetyl, but Oenocuccus oeni produces lower concentrations and the range is wide (0.15–4 mg L−1). However, there are numerous facors influencing diacetyl formation during this process. A low inoculation concentration, some oxygen supply during malo-lactic fermentation, a higher citric acid concentration, a low temperature (18°C rather than 25°C) and a low pH value all seem to stimulate the concentration of diacetyl formed. Sulfur dioxide also plays a role in this process, inhibiting the growth of yeast and bacteria and stabilizing the diacetyl concentration.
The ketones (ketonic terpenes) β-damascenone, α-, and β-ionone with flavour significance are believed to exist in grapes or at least musts. These so-called isoprenoid ketones are postulated to arise from the enzymatic oxidation and cleavage of β-carotene (and other carotenoid substances) during the crushing of the grapes (Ribéreau-Gayon et al., 2006). There may also be increase in amount as the result of ‘in-bottle’ ageing. Oak ageing may also release some α- and β-ionone (Ribéreau-Gayon et al., 2006).
7.4.4 Acetals
Acetals (7.I) are found in wines, particularly in fortified wines such as Sherries, Port wines and Madeira, and are formed almost entirely by conventional chemical reaction between components subsequent to their production in the vinification process (fermentation). Oxidative storage conditions, such as for Madeira and Port wines, enhances the levels of aldehydes and subsequently the formation of acetals, see Chapter 6 for more information.
Acetalization is the reversible reaction between an alcohol (or polyol) and an aldehyde, as shown for (7.I):
Formation is enhanced by excess alcohol and low pH. When R is CH3, as in ethanal, and R1 is C2H5, as in ethanol, we may get the simplest acetal, 1,1-diethoxyethane, also known as diethyl acetal. There is an isomer 1,2-diethoxyethane.
Acetaldehyde will similarly react with other high alcohols present, e.g. propanol. Other aldehydes present, such as pentanal and 2-phenylacetal-dehyde, will form acetals that are known to be present.
Reaction with ethanal may also occur with butan-1,2,-diol (glycol) or glycerol present as shown below.
The glycerol acetal formed is cis-4 hydroxymethyl-2-methyl-1,3-dioxalane (7.II).
There has, however, to be sufficient free aldehyde present for reaction, which is less likely in table wines compared with Sherry, Port wines or Madeira.
7.4.5 Higher alcohols
As already mentioned, straight-chain higher alcohols or fusel oils are largely the products of fermentation and closely parallel the generation of ethyl alcohol. Sponholz (1988) quoted by Jackson (2008) says that the formation of higher alcohols is markedly influenced by winery practices, so that synthesis is favoured by the presence of oxygen, high fermentation temperatures and the presence of suspended material into fermenting mash. However, Ribéreau-Gayon et al. (2006) suggest the opposite to be true in Section 7.2.4. Overall, factors stimulating the fermentation generally stimulate the formation of higher alcohols.
Chaptalization and pressure tank fermentation also tend to enhance synthesis. Chen (1978), also quoted by Jackson (2008), says that certain higher alcohols may originate from grape-derived aldehydes (as we have seen through crushing in Section 7.4.2) and by the reductive denitrification of amino acids (see Section 7.4.1) or via synthesis from sugars. It seems that a higher amino acid content in the must leads to a greater higher alcohol content, since amino acids are the precursor for a number of higher alcohols. Additional higher alcohols are formed from metabolic action of spoilage yeasts and bacteria. Pleasant smelling lighter alcohols may be produced by particular micro-organisms, e.g. oct-1-en-3-ol as in grapes with ‘Noble Rot’ (see Section 7.5).
According to the early twentieth century work of Ehrlich, the production of fusel oils is due to the need for the living yeast cell to provide itself with protein by disruption of amino acids in the fermenting mash. Many higher alcohols are formed by decarboxylation followed by reduction of α-keto acids, produced as intermediate compounds in the amino acid synthesis and catabolism. Leucines give rise to isoamyl alcohol (3-ethyl-butan-1-ol), isoleucine to rotatory amyl alcohol (2-methyl-butan-1-ol), and valine to isobutyl alcohol (2-methylpropan-1-ol). These branched chain alcohols are formed via the so-called Ehrlich pathway, involving amino transferases, decarboxylases and dehydrogenases. The addition of leucine, etc. will increase the overall content of fusel oil but by the addition of other nitrogen compounds, which are more easily assimilable by the yeast, the formation of higher alcohols during fermentation may be avoided. Early on, it was also found that the active amyl alcohol content in fusel oils prepared from potatoes, corn and molasses, differ markedly with higher amounts of the latter. Subsequent scientific opinion, however, suggests that the protein requirements of the yeast are largely met by synthesis of inorganic nitrogen present. The aromatic alcohols 2-phenyl-ethanol, tyrosol and tryptophol have phenyl alanine, tyrosine and tryptophan as their precursors, whilst methionol is formed from methionine.
Glycerol is is formed as part of the conversion of sugar to alcohol (see Ugliano & Henschke, 2009) and its content in wine ranges from 5–14 g L−1, with red wines tending to have higher levels than white wines. Higher levels are found in wines made from grapes affected by Botrytis (see Section 7.5). The formation of glycerol during fermentation can be affected by the available nitrogen for yeast fermentation, as reviewed by Ugliano et al. (2007). Glycerol itself is sweet, however, its contribution to the sweetness or mouthfeel of a wine is still not clear. The threshold concentration for sweetness is high (5.2 g L−1, Noble & Bursick, 1984, quoted by Ugliano & Henschke, 2009) and the high acidity in wines is expected to interact with residual sweetness, so the extent to which glycerol contributes to sweetness or mouthfeel aspects is still unknown.
7.4.6 Furanones and lactones
These compounds can be present in wines via a number of pathways. The simpler lactones like γ-butyrolactone (dihydro-3(H)-furan-2-one) can arise in the fermentation, by the lactonization of γ-hydroxybutanoic acid, a somewhat unstable substance, itself being formed by the deamination and decarboxylation of free glutamic acid or from protein present. Lactones can be present in the grapes, thus in Riesling and Merlot (see Chapter 4) and Jura wine, Port wines and Madeira (sotolon). Sotolon, however, is more characteristic of botrytized wines (see Section 7.5), but can be produced by a condensation reaction between γ-ketobutyric acid and ethanol. They can be formed from amino or organic acids, such as glutamic and succinic acid.
These compounds can be formed from saccharide fragmentation and the Maillard reaction, as discussed by Grosch (2001). Camara et al. (2004) determined up to 1 mg L−1 sotolon being formed in sweet Madeira wines and they observed that its formation was very highly correlated with the formation of furfural, 5-methylfurfural and ethyl 5-hydroxymethylfurfural. Therefore they concluded that sotolon synthesis in Madeira is probably related to sugar degradation as a result of Maillard reactions.
Other lactones present arise during the ageing processes, particularly of course the oak lactones (see Chapter 4) during ‘in-cask’ ageing in oak barrels. These substances appear to be already present in the untreated oak, and the favourable cis-variant increases in amount after ‘toasting’ of the oak barrels. Solarone is reputed to develop during ‘in-bottle’ ageing, although its concentrations remain generally low.
7.4.7 Acids
Of the few volatile acids found in wines, some acetic acid is produced by the yeast fermentation but any excess is most likely to have been produced by the acetobacter micro-organism in unhygienic conditions (see Chapter 1). Anaerobic acetic acid bacteria metabolize ethanol into acetic acid, causing spoilage, often as a consequence of excess oxygenation. The Saccharomyces strain in wine-making is also known to produce acetic acid, the amount produced depending on the strain. Aetic acid is formed also by oxidation of acetaldehyde. Similarly, the presence of propanoic and butanoic acids is due also to micro-organisms. The higher aliphatic acids C6 (hexanoic), C8 (octanoic) and C10 (decanoic), barely volatile, but reportedly present in wines (together with their esters) result from the yeast fermentation itself, formed as part of the saturated fatty acid metabolism. Branched chain fatty acids are formed by oxidation of aldehydes formed from α-keto acids during the amino acid metabolism.
The main non-volatile acids (tartaric, malic and citric) are already present in the grape must. Succinic acid will be present either/both from the lactic acid bacteria (undesirable) or the malo-lactic fermentation process. The sugar acids, gluconic, glucuronic and galacturonic, formed by oxidation of glucose, are associated primarily with botrytized grapes.
7.4.8 Amines
As free amino acids are present in wine musts, not surprisingly, by decarboxylation, small amounts of amines can be formed, or otherwise by deamination of aldehydes, though bacteria will be needed for this purpose. Small amounts of amines can be formed by yeasts, especially during the early part of the fermentation. Yeast autolysis can also release amines in wine. Their contribution to flavour development is uncertain and only some amines are volatile themselves, usually at higher pH values.
However, certain of these amines are also reportedly converted into amides by Saccharomyces cerevisiae, whilst yeasts generally convert amines into alcohols. Amides do not appear to affect wine flavour.
7.4.9 Phenols (volatile)
Phenols not originally present in the must are formed either in the fermentation or generally released in ageing. Two types of phenols are regarded as important to ‘phenol’ taint (see Chapter 4), vinyl- and ethylphenols. Vinylphenols are formed by enzymatic decarboxylation by the yeast during fermentation, selectively, from two cinnamic acids (p-coumaric and ferulic) present. The effect can be noted in white wines but is lower in red wines, where inhibition by other phenols is occurring. Phenolic acid decarboxylases, usually derived from spoilage organisms, decarboxylate phenolic acids into volatile phenols. Saccharomyces brewing strains can also produce phenolic acid off-flavours in beer but in most strains of S. cerevisiae the phenolic acid decarboxylase activity is very low, hence little or no volatile phenols are formed during fermentation.
There are differences in content of cinnamic acids for red and white wines, depending upon grape variety and ripening conditions, thus concentrations are higher in ripe grapes and those grown in hot climates. An example of varietal difference is that Sémillon or French Colombard often have a higher p-coumaric acid and ferulic acid content than Sauvignon Blanc. The use of certain pectolytic enzymes in must clarification, with contaminant cinnamic esters and the yeast strains used are also influential in determining content of cinnamic acid. It is desirable to keep the cinnamic acid content as low as possible. This subject is very fully reported by Ribéreau-Gayon et al. (2006) and comprehensively reviewed by Suarez et al. (2007).
The presence of ethylphenols, relatively infrequent, arises not during fermentation but rather during ageing, due to the effect of a particular yeast, Brettanomyces/Dekkera (Chatonnet et al., 1992/1993; quoted by Ribéreau-Gayon et al., 2006, and reviewed by Suarez et al., 2007). They claimed that the occurrence of ethyl phenols has become more prominent in aged red wines, in particular due to the current trend requiring ageing in wooden barrels, as it appears Brettanomyces species can thrive in old wood. Wines are aged in wood without any physical clarification processes, which is thought to enhance the quality of the wine leaving the aroma more intact, but this also poses an increased risk of spoilage by slow growing yeasts such as Brettanomyces bruxellensis, Brettanomyces anomalus and Saccharomyces baiili, as well as some lactic acid bacteria, all capable of producing undesirable off-flavours. These organisms can grow in the presence of ethanol and require minimal nutrients. In particular, ageing wines at higher pH values in oak barrels increases the risk of spoilage. With the trend in picking grapes very ripe, at which stage the acidity is lower, this may increasingly be the case.
Numerous factors influence the formation of undesirable volatile phenols, in particular the concentration of precursors and the size of the Brettanomyces/Dekkera population but also alcohol concentration of wine, see Suarez et al. (2007). Prevention seems to be difficult, although good housekeeping helps and detailed information is given regarding the methods for cleaning wooden barrels in order to reduce the problem. It is crucial that barrels are cleaned well, pH of the wine is low, adjusted if necessary, storage is at low temperature and sulfur dioxide is added to protect the wine, since these yeasts are controlled by the sulfur dioxide used. Fining of wine with various fining agents or membrane filtration prior to maturation also reduces the risk.
Interestingly, Suarez et al. (2007) discussed that the formation of highly stable pigments based on malvidin 3-glucoside are reported to play a role in the prevention of ethylphenol formation. Benito et al. (2009) tested Saccharomyces strains with different hydroxycinnamate decarboxylase activities in fermenting red wine musts to enhance the formation of stable anthocyanins and minimize the production of 4-ethylphenol. They were able to increase the formation of vinylphenolic pyroanthocyanins (see Chapter 3) and reduce the concentration of 4-ethylphenol and 4-ethylguiacol, generated by vinylreductase of Dekkera bruxellensis. They concluded that generating vinyl phenols early in the wine-making process allowed them to bind with anthocyanins forming stable coloured compounds and may reduce the risk of off-flavour formation at later stages during barrel ageing.
The use of oak barrels after toasting, during ageing is the main factor in determining the presence of the other phenols identified in the wine; in particular, (iso-)eugenol in large amounts and the cresols in very small amounts. Data has been presented (by Ribéreau-Gayon et al., 2006) relating the degree of toasting (none, light, heavy) to the extractability of the various phenols. Syringol, for example, is only really available until after heavy toasting of the barrels before use for wine maturation.
7.4.10 Terpenes
Terpenes in plants are secondary constituents and its biosynthesis starts with acetyl CoA, as described by Maicas & Mateo (2005). Although micro-organisms can synthesize terpenes, their formation by yeasts has not been observed. Synthesis of terpenes is thought to take place in grape berries. Part of the genome of Pinot Noir has been shown to have the capability to produce terpenes, although this variety does not normally produce significant levels (see Ebeler & Thorngate, 2009).
It is now established that many terpenes (the alcohols and polyols) are present in either free or bound form. The latter are glycosides based on glucose, arabinose, rhamnose and apiose, with diglycosides most frequent. The bound forms are, of course, non-volatile and do not contribute to wine aroma, unless they lose their sugar attachment. The proportion in the free and bound form, in ripe grapes, vary with variety, both within the Muscat aromatic group where the content of both is high and other neutral varieties where the content is low. Data from Günata (1984) quoted by Ribéreau-Gayon et al. (2006) shows higher amounts of monoterpenes, both in the free and bound form in the skins of white Muscat grapes, followed by that in the juice and pulp. Such grape varieties are often given some maceration on the skins during wine-making to extract the terpenes.
The free terpenes pass substantially unchanged during fermentation. Though grapes do contain β-glycosidases that can release more free volatile, odoriferous-free terpenes, they are unable to do so under normal vinification conditions. Various fungal β-glycosidases have been used in experiments to enhance the terpene flavour, although precursers for potential off-flavours can also be released, so care has to be taken in selecting an enzyme. For detailed information on the hydrolysis of terpenes refer to the review by Maicas & Mateo (2005). Fleet (2008) also mentions the potential of non-Saccharomyces yeast species being possible producers of more effective glycosidases to remove the sugars of glycosilated terpenes.
The final terpene composition of a wine will also depend upon the type and time of maturation subsequently prescribed, as already described in Chapter 4. The odoriferous compound, called Ho-trienol for simplicity, is derived from a terpen-diol, by acid hydrolysis during the fermentation. This terpen-diol and other terpene polyols present in grapes are not themselves odoriferous.
7.4.11 Pyrazines
Methoxypyrazines are nitrogen-containing heterocyclic compounds biogenically produced in many fruits and vegetables, including grapes, by the metabolism of amino acids. A large part of the 2-methoxy-3-isobutylpyrazine in Cabernet Sauvignon grapes is located in their skins from which they are extracted in red wines.
The content depends strongly upon climatic and soil conditions, so that unripe grapes have the highest amounts, declining with maturity. Direct sunlight on the grape bunches on the vine reduces its 2-methoxy-3-isobutylpyrazine content. However, one study seems to come to the opposite conclusion (Sala et al., 2004), suggesting the lack of photodegradation of 2-methoxy-3-isobutylpyrazine in sunlight did not occur in shaded grapes. They concluded that during maceration 2-methoxy-3-isobutylpyrazine was also released from the solid parts of the grapes, whilst malo-lactic fermentation did not influence the concentration.
A recent model study on the formation of possible flavour compounds in wine via Maillard and Strecker degradation using temperatures and pH values akin to wine storage conditions and carbonyl compounds present in wines (glyoxal, methylglyoxal, diacetyl, pentan-2,3-dione, acetoin and acetol) showed the possibility of some of these compounds forming under storage conditions (Pripis-Nicolau et al., 2000). In the presence of sulfur amino acids, in particular cysteine, the authors reported the formation of hetrocyclic compounds, in particular pyrazines, methyl pyrazines, methyl thiazoles, acetylthiazolines, acetylthiazolidines, trimethyloxazole and dimethylethyloxazoles. These model observations will need to be confirmed in further studies.
7.4.12 Sulfur compounds
There are five different groups of sulfur compounds in wine: thiols, sulfides, polysulfides, thioesters and heterocyclic compounds. Only a few contribute positively to the aroma of wine, as discussed in Chapter 4, most cause off-flavours, often referred to as reduction defects and are produced during alcoholic fermentation. There is a range of origins of sulfur compounds in wine, but wine makers would like to be able to avoid their formation. Juice settling, for white wines, use of sulfur dioxide and selected yeast strain helps to prevent their formation.
Table 7.2 Concentrations of sulfur compounds formed during wine-making.
Compound category | Concentration range detected (μg L −1 ) |
Sulfides | 0–480 |
Mercaptans | 0–16 |
Thioacetates | 0–56 |
Thioalcohols | 0–4500 |
Tiazoles | 0–14 |
Others | 0–5 |
Data adapted from Ugliano & Henschke (2009).
During normal wine-making low concentrations of hydrogen sulfide (3–4 μg L−1) and methanthiol (<1 μg L−1) are produced by yeasts. The latter is formed from methionine by deamination, then decarboxylation to methional, followed by reduction to methionol. Hydrogen sulfide is formed by the sulfate reduction sequence pathway. A shortage of nitrogen during the fermentation is one of the main causes of hydrogen sulfide accumulation. Autolysis at the end of fermentation can also release hydrogen sulfide into the wine. Numerous other sulfur-containing (off) odours are formed as a result of reactions of the reactive hydrogen sulfide, for example reaction with ethanol gives ethanethiol, a mercaptan. Some sulfide compounds can be eliminated from wine by aeration. Ugliano & Henschke (2009) reviewed the sulfur metabolism in Saccharomyces cerevisiae, although not all the genetic information is available. Only very small concentrations are formed, but because these compounds have generally very low odour threshold values, their sensory impact can be significant. Table 7.2 shows typical concentrations in wine.
Recently, five somewhat complex volatile thiols positively contributing to the wine aroma have been identified, especially in Sauvignon Blanc wines and are listed in Chapter 4. Three of these (e.g. 4-mercapto-4-methylpentan-2-one or 4MMP, 4-mercapto-4-methylpentan-2-ol or 4MMPOH and 3-mercaptohexanol or 3MH) have also been found in the must of Sauvignon Blanc as their non-odorous conjugate form with S-cysteine, which releases the free volatile form during fermentation by the presence of a specific β-lyase in the yeast. Only a small concentration of thiols is released, proportional to the concentration of precursors. Most of the 4MMP and 4-MMPOH precursors are found in the flesh. The strain of Saccharomyces cerevisiae used is important, to ensure optimum activity of this enzyme to release the volatile thiol compounds from cysteinylated precursors.
Furfurylthiol is formed by yeasts, using furfural extracted from oak during fermentation as a precursor but the mechanism is not yet known.
A model study on the formation of possible flavour compounds in wine via Maillaird and Strecker degradation by Pripis-Nicolau et al. (2000) showed that in the presence of sulfur amino acids, in particular cysteine, some sulfur compounds were formed and they suggested that just after the fermentation sufficient carbonyl compounds, such as diacetyl, may be present for reactions with cysteine to occur. Numerous off-flavours, such as hydrogen sulfide, methanthiol, dimethyldisulfide and carbon disulfide, were also formed. It will be interesting to see whether these reactions observed in models can also occur during wine storage, this may give future opportunities to allow formation of selected flavour compounds by ensuring the correct storage conditions and fermenting with yeasts that give the precursors required for such reactions.
7.5 Noble Rot
Following infection, which may take place early in grape growth and which requires release of nutrients through the grape skin, the micro-organism Botrytis cinerea (a mould) then injects active enzymes and cause changes in the infected grapes. These changes are in addition to a drying effect by removal of water from within the grape and cause concentration of the sugars. This drying effect limits the extent of secondary invasion by other bacteria and fungi. The chemical changes that occur within the grape are considered (Jackson, 2008; Ribéreau-Gayon et al., 2006) to be:
(1) Pectolytic enzyme action. The pectins of the cell wall are degraded, causing collapse and death of tissues, leading to moisture loss.
(2) Formation of gluconic acid. This is more likely, however, to result from invasion by acetic acid bacteria, which will also produce acetic acid and ethyl acetate.
(3) Increase of citric acid content. The increase will be roughly proportional to the weight loss by drying.
(4) Terpene conversion. Linalool, geraniol and nerol are metabolized to the less volatile compounds, β-pinene, α-terpineol and various pyran and furan oxides, so that the Muscat variety of grape will be particularly affected. Over 20 terpene derivatives have been isolated from infected grapes.
(5) Ester degradation. Non-specific.
(6) New syntheses. In particular, sotolon, 4,5-dimethyl-3-hydroxy-2(5H)-furanone, which is reported to have a ‘toasty/spicy’ fragrance, and 1-octen-3-ol (‘the mushroom’ alcohol), are formed.
(7) Thiamine deficiency. This factor increases the synthesis of sulfur-binding compounds during fermentation, so that the free SO2 content in wine may be markedly reduced unless much higher additions of total SO2 are used to achieve the required free SO2 content. Acetic acid bacteria also produce sulfur-binding compounds, like 2- and 5-oxoglutaric acids (2-oxo-pentan-1,3-dioic acid).
(8) Chemical changes by the activity of laccases. These enzymes, present in Botrytis cinerea, are most likely to be influential in the must of the grape during fermentation. They will oxidize 1,2-, 1,3- and 1,4-diphenols and the anthocyanins, and are particularly active at the pH of wine, so that they need to be inhibited by higher concentrations of total SO2 (∼50 mg L−1) at pH 3.4 in wine. In must, much higher levels of SO2 are needed to inhibit laccase.
Botrytis cinerea has several other effects, significant in the vinification process:
(1) Synthesis of high molecular weight polysaccharides, polymers of mannose and galactose, increasing the acetic and glycerol content in fermentation, high polymers of glucose (glucans), which form strand-like colloids in the presence of alcohol, which can clog filters in filtration.
(2) Calcium salt instability. B. cinerea produces an enzyme, which oxidizes galacturonic acid, a breakdown product of pectin, into galactaric acid, the calcium salt of which becomes insoluble, leading sometimes to sediments in bottles of botrytized wine.
A number of sulfur compounds have been identified in wines made from Botrytis infected grapes, determined in Sauternes wines after fermentation and are thought to contribute to the aroma (Sarrazin et al., 2007; Bailly et al., 2006). The most significant compounds are listed in Table 7.3.
Sensory evaluations showed the additive effects between these sulfur compounds. Most of the polyfunctional thiol compounds disappeared after two years of bottle ageing (Bailly et al., 2009), only 3-sulfanylhexan-1-ol remained present above threshold concentration. However, other key odorants determined in young Noble Rot wines could still be found after about six years bottle ageing, such as α-terpineol, sotolon, fermentation alcohols, esters, and oak related maturation compounds (guiacol, vanillin, eugenol, β-damascenone, trans-non-2-enal, β-methyl-γ-lactone, γ-nonalactone and furaneol). A new compound, abnexon, was isolated and identified. It is formed at about threshold level in these aged wines (about 7 μg L−1), and contributes a honey, spicy aroma to aged Sauternes wines made from Botrytis affected grapes.
Table 7.3 Sulfur compounds and their sensory property in wines made from Botrytis infected grapes.
Compound | Sensory property | Ref. |
3-Sulfanylpentan-1-ol | citrus | a |
3-Sulfanylheptan-1-ol | citrus | a |
2-Methyl-3-sulfanylbutanol | raw onion | a |
3-Sulfanylhexan-1-ol | fruity | a |
3-Sulfanyl-3-methylbutanal | bacon, petroleum | b |
2-Methylfuran-3-thiol | bacon, petroleum | b |
aSarrazin et al. (2007). aBailly et al. (2006).
Bibliography
General texts
Jackson, R.S. (2008) Wine Science, Principles, Practice, Perception, 3nd edn. Academic Press, San Diego.
Ribéreau-Gayon, P., Glories, Y., Maujean, A. & Dubordieu, D. (2006) Handbook of Enology, Vol. 2 The Chemistry of Wine, Stabilization and Treatments. John Wiley & Sons, Ltd, Chichester. loc. cit.
References
Amerine, M.A. & Joslyn, M.A. (1970) Table Wines: The Technology of their Production, quoted by Jackson, R.S. Wine Science (1994) loc. cit. pp. 236–241.
Bailly, S., Jerkovic, V., Marchand-Brynaert, J. & Collin, S. (2006) Aroma extraction dilution analysis of Sauternes wines. Key role of polyfunctional thiols. Journal of Agriculture and Food Chemistry, 54, 7227–7234.
Bailly, S., Jerkovic, V., Meuree, A., Timmermans, A. & Collin, S. (2009) Fate of key odourants in Sauternes wines through aging. Journal of Agriculture and Food Chemistry, 57, 8557–8563.
Barr, A. (1988) Wine Snobbery, p. 247. Faber and Faber, London.
Bartowsky, E.J. & Henschke, P.A. (2004) The ‘buttery’ attribute of wine -diacetyl- desirability, spoilage and beyond. International Journal of Food Microbiology, 96, 235–252.
Bell, S.J. & Henschke, P.A. (2005) Implication of nitrogen nutrition for grapes, fermentation and wine. Australian Journal of Grape and Wine Research, 11, 242–295.
Benito, S., Palomero, F., Morata, A., Uthurry, C. & Suarez-Lepe, J.A. (2009) Minimisation of ethylphenol precursors in red wines via the formation of pyranoanthocyanins by selected yeasts. International Journal of Food Microbiology, 132, 145–152.
Bisson, L.F. & Karpel, J.E. (2010) Genetics of yeasts impacting on wine quality. Annual Review of Food Science and Technology, 1, 139–162.
Camara, J.S., Marques, J.C., Alves, M.A. & Ferreira, A.C.S. (2004) 3-Hydroxy-4,5-dimethyl-2(5H)-furanone levels in fortified Madeira wines: relationship to sugar content. Journal of Agricultural Food Chemistry, 52, 6765–6769.
Campo, E., Cacho, J. & Ferreira, V. (2007) Solid phase extraction, multidimensional gas chromatography determination of four novel aroma powerful ethyl esters. Assessment of their occurrence and importance in wine and other alcoholic beverages. Journal of Chromatography A, 1140, 180–188.
Chatonnet, P., Boidron, J.N., Dubordieu, D. & Pons, M. (1992) quoted by Ribéreau-Gayon et al. (2000) Handbook of Enology, loc. cit. pp. 218–225.
Chen, E.C-H (1978). The relative importance of Ehrlich and biosynthetic pathways to the fermentation of fusel alcohols, quoted by Jackson, R.S. (1994) loc. cit. p. 185.
Drawert, F. (1974) Wine making as a biotechnological sequence. In: Chemistry of Wine Making. Advances in Chemistry Series, 157, pp. 1–10. American Chemical Society, Washington.
Ebeler, S.E. & Thorngate, J.H. (2009) Wine chemistry and flavour: Looking into the crystal glass. Journal of Agricultural and Food Chemistry, 57, 8090–8108.
Fischer, U., Strasser, M. & Gutzler, K. (2000) Impact of fermentation technology on the phenolic and volatile composition of German red wines. International Journal of Food Science and Technology, 35(1), 81–94.
Fleet, G. (2008) Wine yeasts for the future. Federation of European Microbiological Sciences Yeast Research, 8, 978–995.
Grosch, W. (2001) Volatile Compounds in Coffee: Recent Developments (eds. R.J. Clarke & O.G. Vilzthum), pp. 68–89. Blackwell Publishing Ltd., Oxford.
Günate, Z. (1984) Terpenes, quoted by Ribéreau-Gayon, P. et al. (2006) Handbook of Enology II, loc. cit. pp. 206–211.
Hernandez-Orte, P., Cersosimo, M., Loscos, N., Cacho, J., Garcia-Moruno, E. & Ferreira, V. (2009) Aroma development from non-floral grape precursors by wine lactic acid bacteria. Food Research International, 42, 773–781.
Howell, K.S., Klein, M., Swiegers, J.H., Hayasaka, Y., Elsey, G.M., Fleet, G.H., Hoy, P.B., Pretorius, I.S. & de Barros Lopes, A. (2005) Genetic determinants of volatile-thiol release by Saccharomyces cerevisiae during wine fermentation. Applied and Environmental Microbiology, 71, 5420–5426.
Kalua, C.M. & Boss, P.K. (2009) Evolution of volatile compounds during the development of Cabernet Sauvignon grapes (Vitis vinifera L.) Journal of Agriculture and Food Chemistry, 57, 3818–3830.
Loscos, N., Hernandez-Orte, P., Cacho, J. & Ferreira, V. (2009) Comparison of suitability of different hydrolytic strategies to predict aroma potential of different grape varieties. Journal of Agriculture and Food Chemistry, 57, 2468–2480.
Pripis-Nicolau, L., de Revel, G., Bertand, A. & Maujean, A. (2000) Formation of flavor components by the reaction of amino acid and carbonyl compounds in mild conditions. Journal of Agriculture and Food Chemistry, 48, 3761–3766.
Ribéreau-Gayon, P. (1978) Wine flavour. In: Flavour of Food and Beverages (eds. G. Charalambous & G.E. Inglett), p. 370. Academic Press, New York.
Ribéreau-Gayon, P., Glories, Y., Maujean, A. & Dubordieu, D. (2000) Handbook of Enology, Vol. I, loc. cit. pp. 193–161 (carotenoid breakdown), pp. 383–389 (barrel toasting).
Robinson, J. (1995) Jancis Robinson’s Wine Course, pp. 81–82. BBC Books, London.
Saerens, S.M.G., Delvauz, F., Verstrepen, K.J., Van Dijck, P., Thevelein, J.M. & Delvaux, F.R. (2008) parameters affecting ethyl ester production by Saccharomyces cerevisiae during fermentation. Applied and Environmental Microbiology, 74, 454–461.
Sala, C., Busto, O., Guasch, J. & Zamora, F. (2004) Influence of vine training and sunlight exposure on the 3-alkyl-2-methoxypyrazines content in musts and wines from the Vitis vinifera variety Cabernet sauvignon. Journal of Agriculture and Food Chemistry, 52, 3492–3497.
Sarrazin, E., Shinkaruk, S., Tominaga, T., Bennetau, B., Frerot, E. & Dubourdieu, D. (2007) Odourous impact of volatile thiols on the aroma of young botrytized sweet wines: Identification and quantification of new sulphanyl alcohols. Journal of Agriculture and Food Chemistry, 55, 1437–1444.
Soles, R.M., Ough, C.S. & Kunkee, A. (1982) Ester concentration differences in wine fermented by various species and strains of yeast. American Journal of Enology and Viticulture, 33, 94–98.
Soufleros, A. (1978) University thesis quoted by Ribéreau-Gayon, P. (1978). Wine Flavour. In: Flavour of Foods and Beverages (eds. G. Charalambous & G. Inglett), p. 369 (different yeasts), p. 370 (ester formation). Academic Press, London.
Sponholz, W.R. (1988) Alcohols derived from sugars and other sources, quoted by Jackson, R.S. (2000), loc. cit. p. 185.
Suarez, R., Suarez-Lepe, J.A., Morata, A. & Calderon, F. (2007) The production of ethylphenols in wine by yeasts of the genera Brettanomyces and Dekkera: A review. Food Chemistry, 102, 10–21.
Swiegers, J.H., Bartowsky, E.J., Henschke, P.A. & Pretorius, I.S. (2005) Yeast and bacterial modulation of wine aroma and flavour. Australian Journal of Grape and Wine Research, 11, 139–173.
Swiegers, J.H. & Pretorius, I.S. (2005) Yeast modulation of wine flavour. Advances in Applied Microbiology, 37, 131–175.
Ugliano, M., Henschke, P.A., Herderich, M.J. & Pretorius, I.S. (2007) Nitrogen management is critical for wine flavour and style. Wine Industry Journal, 22(6), 24–30.
Ugliano, M. & Henschke, P.A. (2009) Yeasts and Wine Flavour. In: Wine Chemistry and Biochemistry (eds. M.V. Moreno-Arribas & M.C. Polo), pp. 313–392. Springer ScienceBusiness Media, LLC.
Ugliano, M., Fedrizzi, B., Siebert, T., Travis, B., Magno, F., Versini, G. & Henschke, P.A. (2009) Effect of nitrogen supplementaion and Saccharomyces cerevisiae on hydrogen sulfide and other volatile sulphur compounds in Shiraz fermentaion and wine. Journal of Agriculture and Food Chemistry, 57, 4948–4955.
Varela, C., Siebert, T., Cozzolino, D., Rose, L., McClean, H. & Henschke, P.A. (2009) Discovering a chemical basis for differentiating wines made by fermentation with ‘wild’ indigenous and inoculated yeasts: role of yeast volatile compounds. Australian Journal of Grape and Wine Research, 15, 238–248.
Vilanova, M., Ugliano, M., Varela, C., Siebert, T., Pretorius, I.S. & Henschke, P.A. (2007) Assimilable nitrogen utilisation and production of volatile and non-volatile compounds in chemically defined medium by Saccharomyces cerevisiae wine yeast. Applied Microbial Biotechnology, 77, 145–157.