Introduction
The convergence of political, financial, medical, and basic science interests is transforming the field of marijuana science. In three decades from 1930 to 1960, a scant 109 marijuana-related reports were published in the biomedical literature. Fifty years later, in a single decade from 2011 to fall of 2019, a staggering 15,269 marijuana manuscripts were published. This 140-fold increase in the scientific literature was catalyzed by changes in our perception of marijuana and by the discovery of an entirely new signaling system, the endocannabinoid system, in living organisms. Shortly after this discovery, revelations appeared on how plant-based cannabinoids (phytocannabinoids) and de novo synthetically produced cannabinoids target and modulate this system in the brain and other organs. The current phase of this near-exponential growth in scientific inquisitiveness focuses on the biological mechanisms by which cannabinoids contribute to pathophysiology or the potential of cannabinoids for treating diseases. Endocannabinoids and their receptors are now recognized as significant modulators of human physiology and pathophysiology. This signaling system is among the most dense, widely distributed, and versatile in animals and humans.
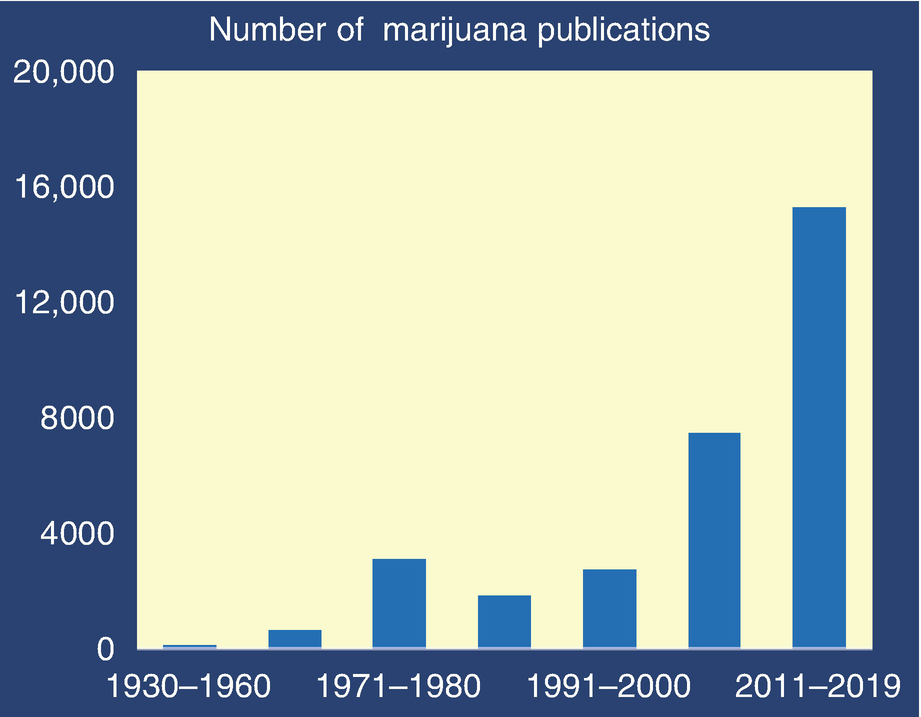
The review summarizes the endocannabinoid system and actions of exogenous cannabinoids on this system, but with a caveat: The composition of marijuana has changed dramatically with medicalization, legalization, and commercialization [1]. Marijuana is a complex plant [2] with over 700 identified constituents, including more than 100 cannabinoids. The most prominent of these are THC (Δ9-tetrahydrocannabinol) and CBD (cannabidiol). For most of the 20th century, research on marijuana biology was based on low-potency (low THC) botanical marijuana converted into crude smokable preparations of crushed plant parts and stems containing ~1–3% THC. Findings from this period require tempering or are obsolete, with the advent of high-potency strains of the plant (~20% THC), extreme potency of marijuana concentrates (as high as 90% THC), high THC:CBD ratios (>100), an expanding repertoire of synthetic cannabinoids [3], new routes of administration (e.g., vaping, edibles), and increased daily use. These parameters can influence the pharmacological actions of cannabinoids.
Discovery, Evolutionary Origins, and Function of the Endocannabinoid System
Among the many insights provided by the human genome project was the discovery of an abundance of genes implicated in cell-cell communication. Signaling between cells is a critical mechanism to coordinate the activities of multicellular organisms, enabling development, repair, immunity, homeostasis, and response to environmental cues. In general, communication systems are complex and comprise at least five components: a mechanism to synthesize, store, and release a signaling molecule (neurotransmitter or hormone) by the signaling cell; transport or diffusion of the signaling molecule to its target; binding and activation of a specific receptor (target); initiation of a signal transduction pathway to amplify or diminish the signal; and a mechanism to inactivate the signal. Signaling molecules can belong to different chemical classes, to include lipids, amino acids, monoamines, proteins, or gases. Receptors are proteins embedded in cell surface membranes, or function intracellularly, or mobilize and circulate between the two environments. At least 4% of our genome encodes G protein-coupled receptors, which are crucial for vision, smell, taste, immune response, water balance, blood pressure, and higher brain functions. Spanning the full thickness of cell membranes, G protein-coupled receptors are activated by a signaling molecule (e.g., dopamine) to undergo a conformational change. The change transfers the signal to the cell interior by enabling the receptor to bind to G proteins (guanine nucleotide-binding proteins), molecular switches inside cells that transmit signals generated by cell surface receptors. They activate effectors (e.g., enzymes such as adenylate cyclase or phospholipase C or RhoGEFs) to produce transducing molecules, e.g., cAMP, that amplify or depress signals to trigger a cascade of signaling events that change cell function.
Communication is the core activity of the brain. It has the most highly developed and complex system of cell-cell communication dynamics: ~80 billion nerve cells (neurons), each of which can make a few or 10,000+ connections to other neurons for signaling, with neurons supported by ~1 trillion glia (non-neuronal cells). G protein-coupled receptors are abundant in the brain (over 100 types) and include adrenergic, dopaminergic, muscarinic cholinergic, metabotropic glutamate, opioid, and other peptide receptors, olfactory, and light-sensitive photoreceptors. It is now established that the endocannabinoid signaling system is comprised of G protein-coupled receptors, the principal targets of THC in brain and peripheral organs, endocannabinoids, and enzymes that synthesize and degrade endocannabinoids.
Discovery of an endocannabinoid system was driven by scientific curiosity: how do marijuana, THC, and other exogenous cannabinoids so profoundly modulate behavioral, cognitive, motor, and physiological functions of living organisms? It was evident early on that THC, the principal and psychoactive constituent of marijuana, did not target any known receptor system in the brain, such as dopamine, serotonin, glutamate, cholinergic, adrenergic, or GABAergic receptors. Did it target a novel, as yet undiscovered signaling system? Isolation of THC and CBD from marijuana [4], [5], [6] and synthesis of an array of high-potency cannabinoids preceded and were essential for the discovery of the endocannabinoid system.
The search began by probing for a receptor. The first breakthrough came indirectly, by finding that cannabinoids inhibited cyclic AMP accumulation in neuronal cells [7]. As described above, cAMP translates receptor activity into a cascade of intracellular events, by amplifying or depressing G protein receptor signals. cAMP modulation was followed by the discovery that THC effects were stereoselective, another incentive to seek a receptor. Stereoselective or three-point (three-dimensional) binding of signaling molecules (ligands) is a common feature of receptors [8]. These leads drove the next stage of discovery. Using a radiolabeled, potent analog of THC as a ligand, Howlett identified a receptor in brain tissue that displayed appropriate pharmacological specificity for cannabinoids [9]. A radiolabeled probe was also used to map this newly discovered cannabinoid receptor in the brain [10]. Intriguingly, the brain distribution coincided with the brain map of an unknown, newly cloned receptor gene from a cDNA library [11, 12]. Confirmation was swift, as the gene encoded a protein with the same pharmacological properties as the receptor characterized in brain tissues. The existence of a brain cannabinoid receptor was indisputable. Intriguingly, the brain distribution of this receptor also corresponded to the known pharmacological actions of THC, with high to moderate expression levels in regions implicated in coordination, learning, memory, cognition, executive function, reward, and emotional state. With one receptor (CB1) now confirmed and a second cloned receptor (CB2) discovered shortly afterwards by structural homology [13], the field was ripe for discovering endocannabinoids, signaling molecules in the brain that would activate CB1 and CB2 receptors. As THC is a lipophilic molecule, it was assumed that an endogenous cannabinoid would probably be lipid-like. Indeed, using methods to isolate lipids, the discovery of the first endogenous cannabinoid signaling molecule, anandamide (AEA or N-arachidonoylethanolamine), followed shortly thereafter [14]. AEA is derived from arachidonic acid, a precursor of prostaglandins, leukotrienes, and others. Another arachidonic acid derivative which activates cannabinoid receptors, 2-AG (2-arachidonoylglycerol), was subsequently isolated [15]. 2-AG is now recognized as a primary cannabinoid transmitter in the brain. Other receptors implicated in cannabinoid signaling, but not proven definitively are GPR18 and GPR55. They exhibit limited sequence homology with CB1 and CB2 cannabinoid receptors, but their pharmacology overlaps [16]. The enzymes N-acyl phosphatidylethanolamine phospholipase D (NAPE-PLD), fatty acid amide hydrolase (FAAH), diacylglycerol lipase (DAGL), monoacylglycerol lipase (MAGL), and α/ß-hydrolase domain 6 (ABHD6) were shown to be critical for synthesizing, degrading, and regulating availability of endocannabinoids [17].
In conformity with other signaling systems, it is now established that the endocannabinoid system is composed of at least two receptors, CB1 and CB2, which are activated by endocannabinoid neurotransmitters 2-AG and AEA, to elicit physiological responses. Endocannabinoid availability is regulated by synthetic (e.g., diacylglycerol lipase alpha) and degradative enzymes (fatty acid amide hydrolase or FAAH).
From an evolutionary perspective, the cannabinoid signaling system is ancient [18]. Comprehensive reviews of cannabinoid signaling show that invertebrates and advanced vertebrate organisms express cannabinoid signaling proteins. Although CB1 and CB2 receptors are unique to chordates, enzymatic synthesis and inactivation of endocannabinoids occur throughout the animal kingdom, even in the absence of CB1 or CB2 receptors. Accordingly, other receptors, e.g., transient receptor potential vanilloid-type ion channels, may also be used by endocannabinoids for signaling, with endocannabinoids binding to other proteins to elicit signaling responses. 2-AG is generated by diacylglycerol lipase alpha and acts via presynaptic CB1 cannabinoid receptors to inhibit neurotransmitter release. This retrograde signaling mechanism occurs throughout the vertebrate animal tree, with endocannabinoid signaling implicated in learning, locomotion, and feeding. Even though the endocannabinoid system is not unique to humans, certain human responses to cannabinoids (e.g., marijuana drug-seeking) are challenging to replicate in preclinical animal studies. For example, Western hemisphere squirrel monkeys, but not old-world monkeys, can be trained to self-administer THC [19, 20]. Squirrel monkeys will even self-administer an inhibitor of anandamide transport [21]. Conditioned place preference (animals prefer to spend time in a chamber associated with liking an administered drug) is another measure of drug liking/seeking. THC does not elicit conditioned place preference in rodents, except in those primed with prior exposure to cigarettes via smoke or vaping [22, 23]. Other drugs with abuse liability (cocaine, heroin) are readily self-administered by various species and engender robust conditioned place preference. Conceivably, the endocannabinoid system in humans is unique and elicits interoceptive effects (subjective responses) that may not be replicable in other mammalian species.
Endocannabinoid System in the Brain
The endocannabinoid system has four main components: (1) G protein-coupled cannabinoid CB1 and CB2 receptors, (2) endocannabinoids that target cannabinoid receptors and possibly other receptors, (3) enzymes that catalyze endocannabinoid biosynthesis and metabolism, and (4) mechanisms involved in cell modulation of specific endocannabinoids. Within the endocannabinoid system, cannabinoid receptors, enzymes, and genes represent putative therapeutic targets for addressing metabolic and behavioral dysfunctions.
The endocannabinoid system is widespread throughout the brain. Endocannabinoids have a major role as retrograde transmitters in many brain regions, although often with region-specific specialization. A synapse is a small aqueous gap between neuronal extensions (axons, dendrites) across which transmitters diffuse. Most transmitters are released from presynaptic nerve terminals when the nerve is excited and activate receptors postsynaptically. In contrast to this general principle, the highest levels of CB1 receptors are found presynaptically in most brain regions, while endocannabinoid synthesizing enzymes are postsynaptic. AEA and 2-AG are made and released postsynaptically, diffuse to presynaptic neurons expressing cannabinoid receptors, and activate the receptors. Receptor activation modulates release of other neurotransmitters in the same cell.
The CB1 receptor is expressed in the brain and peripheral tissues, producing multiple physiological responses. In the brain, it is among the most abundant of the G protein-coupled receptors and mediates most if not all the psychoactive effects of THC in marijuana. Its brain distribution is strikingly consistent with the pharmacology of marijuana: CB1 receptors are enriched in the cerebellum (cognition, coordination), hippocampus (learning and memory), cortex (cognitive function, executive function and control, integration of sensory input), basal ganglia (motor control, planning), ventral striatum (prediction and feeling of reward), amygdala (anxiety, emotion, fear), hypothalamus (appetite, hormone levels, sexual behavior), brain stem, and spinal cord (vomiting, pain). CB1 is also found on vasculature and in some astrocytes and microglia. In peripheral tissues, CB1 receptors have been localized to the adrenal gland, heart, lung, prostate, liver, uterus, ovary, testes, vas deferens, bone marrow, thymus and tonsils myocardium, adipocytes, smooth muscle, and preganglionic sympathetic neurons. The CB2 receptor is expressed in peripheral blood mononuclear cells (macrophages, B cells, and natural killer cells), myocardium, vascular endothelium, smooth muscle, spleen, tonsils, thymus, and leukocytes, as well as the lung, testes, and sparsely in brain neurons. In brain microglia, it may regulate neuroinflammatory responses. CB2 receptors modulate the release of chemical signals primarily engaged in immune system functions (cytokines, immune cell migration) and may play a role in depression and substance abuse [24]. In contrast to CB1 receptors, the relevance of CB2 to problem and compulsive marijuana use is unknown. Anandamide and 2-arachidonoylglycerol function as full agonists at CB1 and CB2 receptors. CB2 receptors are of considerable interest because THC activation of CB2 does not produce psychoactive effects, as does THC on the CB1 receptor. Accordingly, it is a promising target for therapeutics that can circumvent the adverse effects promulgated by marijuana/THC via CB1 receptors. Accumulating evidence suggests that other receptors may be activated by cannabinoids to engender a broader and more divergent range of physiological and pharmacological effects. Several orphan G protein-coupled receptors, GPR18, GPR55, and GPR119, interact with cannabinoids. There may be others.
Five structurally distinct classes of cannabinoid compounds have been identified that bind CB1 receptors: the classical cannabinoids (e.g., THC); bicyclic cannabinoids (e.g., CP-55,940); indole-derived cannabinoids (e.g., WIN 55,212); eicosanoids (e.g., endogenous ligands AEA, 2-AG); indazole agonists (e.g AB-PINACA) and antagonist/inverse agonists (e.g., SR141716A for CB1, SR145528 for CB2) [25]. While few agonists show selectivity for the CB1 or CB2 receptors, antagonist compounds have been synthetized that are highly selective. Structural differences between CB1 and CB2 receptors are reflected in the actions of THC: THC binds CB1 and CB2 receptors with similar affinities, but THC is a partial agonist for CB1 receptors and a weak partial agonist or antagonist at CB2 receptors.
CB receptor molecular genetics and CB regulation and functional consequences are complex [26]. Numerous polymorphic forms of the CB1 receptor have been identified, with sequences comprised of either single nucleotide polymorphisms (SNPs) or large changes in the number of repeat sequences. Polymorphisms in the untranslated region of the genes can affect splicing and promoter activity. Some of the SNPs are silent, while others lead to amino acid substitutions in the receptor protein. Many of the known genetic polymorphisms of the endocannabinoid system have been linked to pathophysiology of substance abuse, mental disorders, and energy metabolism. Specifically, CB1 receptor gene variants have been associated with osteoporosis, ADHD, post-traumatic stress disorder, drug dependency, obesity, and depression. Functional variants of cannabinoid receptor genotypes may be associated with disturbances of the brain involving emotional and social stimuli, such as autism and depression. Polymorphisms in the CB2 gene are frequent and manifest as reduced function of the CB2. CB2 receptors reportedly are associated with disease phenotypes, including osteoporosis, schizophrenia, bipolar disease, depression, and immune-related and eating disorders [23].
Endocannabinoids AEA and 2-AG play a fundamental role in regulating pleasure, memory, thinking, concentration, body movement, awareness of time, appetite, pain, sensory processing (taste, touch, smell, hearing, and sight), and immune response in the brain. They are produced “on demand” from membrane lipid precursors by biosynthetic pathways and are metabolized through distinct routes (see below). AEA and 2-AG can also be oxidized by cyclooxygenase-2, distinct lipoxygenases, or cytochromes P450, and these products likely have different biological activities arising from activation of other non CB1 or CB2 receptors. Endocannabinoids act at CB1 and CB2 receptors with different affinities [27] and may have other targets such as orphan receptors (e.g., GPR55), the transient receptor potential vanilloid 1 (TRPV1) ion channel, and the peroxisome proliferator-activated receptors (PPAR). AEA and 2-AG modulate and “fine-tune” signaling in most brain regions, to enable the brain to adapt to signals generated by multiple sources. Fundamental differences between endocannabinoid with phytocannabinoid signaling have been reported. Isoforms of sn-1-diacylglycerol lipases and N-acyl-phosphatidylethanolamine selective phospholipase D generate 2-AG and AEA, respectively. Endocannabinoids are released on demand in small quantal amounts in discrete brain regions. The process is tightly regulated; it is short-lasting and terminated rapidly by enzymatic degradation, with monoacylglycerol lipase and fatty acid amide hydrolase inactivating 2-AG and AEA, respectively [28]. THC and CBD are not substrates for these enzymes, and therefore signals cannot be rapidly cleared. If metabolic degradation of 2-AG is blocked artificially by genetic disruption of its degradative enzyme, CB1 receptors desensitize and downregulate. In a system designed to respond rapidly and reversibly to stimuli, phytocannabinoid levels and signaling are uncontrolled. Repeated dosing of THC causes the brain to adapt by altering endocannabinoid function, receptor response, promoting CB1 receptor downregulation and engendering a range of other molecular changes.
Endocannabinoid System Function in Early and Adolescent Brain Development
The brain is particularly susceptible to disruptions during the maturational process. A few clues indicate the wisdom of avoiding marijuan use during pregnancy, to enable brain development in utero to proceed undisturbed during this critical phase [29]. Endocannabinoids play a critical role in neuronal and glial development in the brain, guiding neural stem cell survival, fate, proliferation, migration, and differentiation of neuronal and glial cells [39]. The endocannabinoid 2-AG also enhances proliferation and differentiation of oligodendrocyte progenitor cells (a subtype of glia), regulating essential steps in oligodendrocyte development [30]. Developmental endocannabinoid signaling, from fetus to young adult, may be susceptible to marijuana use during pregnancy and adolescence, possibly affecting brain structure and function. Exposure to marijuana in utero can influence neuropsychiatric outcomes in offspring. As a component of the ABCD study [31], a small sample of children exposed to marijuana in utero displayed higher scores on a 21-point rating scale for psychosis proneness [32]. The data suggest that prenatal marijuana exposure may be associated with later psychosis proneness in offspring only when fetal CB1 receptor expression is adequate, which may not occur until after mothers learn they are pregnant. CB1 receptors have been identified in the midgestational fetal human forebrain, in the amygdala, and in the hippocampus [33]. Decreased D2 dopamine receptor mRNA expression in the basal amygdala correlated with the amount of maternal marijuana intake and was specific to this brain region and not to the hippocampus or striatum [34]. Since these brain regions are involved in behavioral and mood disorders, altered dopamine receptor expression could contribute to depressive symptoms and impaired social behaviors, as reported in children upon longitudinal follow-up [35, 36]. These and other findings suggest that use of marijuana during pregnancy should be discouraged.
Initially functioning to determine cell fate prenatally, the endocannabinoid system switches to a different role, homeostatic regulation of synaptic neurotransmission, and bioenergetics in the mature nervous system [37]. In adolescents, marijuana use has been repeatedly shown to adversely impact brain structure and function [38], but with some potential for recovery. Notwithstanding the need for improved measures of use and longitudinal data, deleterious effects are more likely to occur with early age of use and continued use for decades. Adolescence is a vulnerable period because of rapid brain development [39]. The poorly understood mechanisms conceivably are shaped by age of onset, potency, frequency, duration of use, and other factors including polypharmacy and genetics. Dynamic changes in the development of corticolimbic structures occur during adolescence. Structures contributing to fear, stress responsivity, and anxiety-related behavioral regulation are regulated in part by the endocannabinoid system. During adolescence, endocannabinoid signaling modulates the maturation of local and corticolimbic circuit neurons, enhancing communication within and between brain regions and promoting brain development and plasticity. Not surprisingly, this period is sensitive to perturbations of endocannabinoid signaling as related to stress [40], potentially leading to altered developmental trajectories of neural circuits governing emotional behaviors. Perturbations of endocannabinoid signaling during adolescence conceivably hinder structural maturation of the prefrontal cortex and its circuitry [41]. Excess CB1 receptor stimulation alters dendritic arborization of specific cortical neurons. Conversely, blocking endocannabinoid activity during adolescence prevents normal developmental changes, as well [42].
Adolescents are vulnerable to environmental sources of stress and anxiety. Disturbances in endocannabinoid signaling disrupt discrete developmental changes in cortical-limbic circuit regions as manifest by altered emotional and stress responding [43]. Early cannabinoid, especially THC exposure, may increase vulnerability to adverse outcomes. Although CBD is considered safer for young patients, there are no long-term studies investigating CBD effects on brain development [44]. Endocannabinoids and marijuana-altered endocannabinoid signaling may contribute to neuropsychiatric diseases that are of developmental origins and in which modifications to signaling have been observed.
Overall Function of the Endocannabinoid System in the Brain [45]
Understanding the multiple functions of endocannabinoid signaling in the brain offers insight into the pharmacological effects of marijuana and other exogenous cannabinoids, their therapeutic potential, and undesirable adverse effects. An overview by Kalant [46] describes in depth “on-demand” endocannabinoid modulation of excitatory and inhibitory synaptic transmission and regulatory functions in the brain [47].
Endocannabinoid signaling is crucial for brain development. It modulates generation and survival of neurons from embryonic to adult periods [48], guides neural stem cell survival and proliferation, cell fate decisions, motility and differentiation of ensuing neuronal and glial cells (section “Endocannabinoid System in Brain” above) [37]. Developmental endocannabinoid signaling, from fetus to young adult, may be susceptible to marijuana use during pregnancy and adolescence, possibly affecting brain structure and function. Endocannabinoids and marijuana-altered endocannabinoid signaling may contribute to neuropsychiatric diseases that are of developmental origins and in which modifications to signaling have been observed: autism [49], schizophrenia [50], bipolar disorder [51], and depression [52]. The central role of the cannabinoid system in promoting adult neurogenesis in the hippocampus and the lateral ventricles provides insight into the processes underlying post-developmental neurogenesis in the mammalian brain. Abnormal activity of cannabinoid receptors may alter neurogenesis in embryonic or adult nervous systems, possibly influencing the emergence of psychiatric and neurological disorders such as anxiety, depression, and schizophrenia. An understanding of the mechanisms by which cannabinoid signaling influences developmental and adult neurogenesis may accelerate the development of new therapeutic strategies for neurodevelopmental, psychiatric, and neurological disorders. Both THC [53] and CBD [54] inhibit neurogenesis in adolescent or adult rodent brain, a process of potential relevance to a wide range of marijuana-induced adverse events [55]. Systematic review of the current literature indicates that the evidence is scant for therapeutic benefit of cannabinoids in improving depressive disorders and symptoms, anxiety disorders, attention-deficit hyperactivity disorder, Tourette syndrome, post-traumatic stress disorder, or psychosis. The evidence is of low quality that pharmaceutical THC (with or without CBD) leads to a small improvement in symptoms of anxiety among individuals with other medical conditions [56]. Conversely, marijuana use is implicated in psychosis and schizophrenia [57] [58], and its use is associated with poorer recovery from a psychotic disorder, increasing the risk of relapse, rehospitalization, and lower social functioning [59].
Cannabinoids and CB1 and CB2 receptors display neuroprotective effects in the brain by preventing or decreasing the severity of damage resulting from mechanical, blood flow, or other forms of injury. Genetic ablation of the CB1 receptor exacerbates ischemic stroke [60], with CB2 agonists providing anti-inflammatory properties and CB1 activation promoting hypothermia. The use of marijuana for this purpose is compromised by the psychoactive effects and other adverse events of THC, and the development of tolerance to its neuroprotective effects.
The endocannabinoid system contributes to olfactory, auditory, and pain sensations. A review of these functions is beyond the scope of this summary, but readers are referred to an excellent overview [46]. There is extensive anatomical overlap of the opioid and cannabinoid receptor systems, and it appears probable that functional interactions between them occur in the production of analgesia.
A number of nuclei in the medulla are involved in the regulation of appetite and nausea. These nuclei coordinate sensory input from the brain stem, vagal complex, vestibular organs, and peripheral organs. Endocannabinoids and CB1 agonists inhibit vagal fibers to promote eating and CB1 antagonists decrease or inhibit food intake [61]. Even though marijuana has been promoted as an antiemetic for chemotherapy-induced nausea, cannabis hyperemesis syndrome (intractable vomiting) has emerged in heavy marijuana users [62].
Endogenous and exogenous cannabinoids, including marijuana and THC, affect sleep patterns [63]. There is poor quality evidence that marijuana or cannabinoids have therapeutic benefit in sleep disorders [64].
The endocannabinoid system has mood-elevating, antidepressant, and anxiolytic effects. The anxiolytic response to marijuana is biphasic, implying that marijuana dosing is a critical factor in minimizing risk of anxiety and depression and maximizing benefit [65, 66]. Marijuana or THC at high doses increases the risk for depression or anxiety possibly by downregulating CB1 receptors [67–70].
The endogenous cannabinoid system inhibits seizure susceptibility. Accordingly, it is not surprising that exogenous marijuana has antiseizure activity. However, if THC levels are high or if marijuana is consumed by susceptible individuals, THC may promote seizures [71]. CBD has therapeutic antiseizure benefits for rare childhood forms of epilepsy: Dravet and Lennox-Gastaut syndromes [72]. CBD is effective without the psychoactive effects or potential for pro-seizure activity of whole-plant marijuana [71, 73]. However, CBD is not without risk. The adverse events of CBD in animal studies range from developmental toxicity, embryo-fetal mortality, central nervous system inhibition and neurotoxicity, hepatocellular injuries, spermatogenesis reduction, organ weight alterations, male reproductive system alterations, and hypotension, although at doses higher than recommended for human pharmacotherapies. In humans, the therapeutic benfit of CBD for epilepsy and psychiatric disorders reportedly may be compromised by drug-drug interactions, hepatic abnormalities, diarrhea, fatigue, vomiting, and somnolence [74].
The endocannabinoid system plays a complex role in regulating motor pathways, which conceivably are relevant to symptomatic relief, or to addressing the underlying pathology in a wide range of neurological diseases characterized by motor impairment [75]. CB1 receptors are abundant in brain regions that regulate motor function and coordination, including the basal ganglia and cerebellum. CB1 receptors are downregulated in several neurological conditions [76].
Cannabinoids can both facilitate and degrade learning processes dependent upon the process involved. Endocannabinoids apparently facilitate various forms of learning and memory processes in a number of brain regions. The endogenous cannabinoid system is also implicated in extinguishing learning of aversive situations. On the other hand, THC and marijuana decrease working memory, apparently by actions in the hippocampus, a brain region critical for learning and memory. The memory decrements induced by THC or marijuana resemble hippocampal lesions. These impairments may result from suppression of glutamate release in the hippocampus, which is responsible for the establishment of synaptic plasticity [77–79]
The endocannabinoid system modulates pain sensations. There is anatomical overlap of the opioid and cannabinoid receptor systems, and it appears probable that functional interactions between them occur in the production of analgesia. The endocannabinoid system is localized along the pain pathways in the spinal cord and brain pain centers including the thalamus, the dorsal raphe nucleus, and the periaqueductal grey. Both CB1 and CB2 agonists reduce pain responses. CB2-selective agonists have produced analgesia or reduction of hyperalgesia in models of neuropathic and inflammatory pain. There also is extensive overlap between endocannabinoid systems and prostanoid systems. Accordingly, modulation of endocannabinoid metabolism will affect the prostanoid system and conversely. Arachidonic acid is produced with endocannabinoid hydrolysis and anandamide, and 2-arachidonoylglycerol can be converted into prostaglandin-ethanolamides and prostaglandin-glycerol esters. The therapeutic benefit of marijuana or THC for pain management is controversial, with meta-analyses concluding the therapeutic benefit outweighed by adverse events.
Endocannabinoid System in Peripheral Tissues
Endocannabinoid signaling systems are found nearly ubiquitously in the peripheral tissues, with their distribution possibly accounting for the myriad of effects and potential medical applications of individual cannabinoids [80]. Differences in CB1 and CB2 receptor function in the body are a focus of this segment, because THC in the marijuana plant activates both CB1 and CB2 receptors and could have detrimental effects in tissues in which CB1 receptor activity may contribute to pathophysiological states [81].
Accumulating evidence supports endocannabinoid signaling as critical to increased consumption and storage of energy [82]. Endocannabinoids are mobilized during exercise and conceivably replenish energy stores and contribute to analgesic and mood-elevating effects of exercise.
Virtually all gut functions are regulated by endocannabinoids and coordinate CNS control of its metabolic and homeostatic functions. CB1 and CB2 receptors are highly expressed on enteric nerves and on enteroendocrine cells (CB2) throughout the intestinal mucosa, on immune cells (CB1 and CB2), and enterocytes (CB1 and CB2). This discovery was guided by marijuana effects in the GI tract. Endocannabinoid actions in the GI tract are largely mediated by CB2 receptors [81].
CB1, CB2, endocannabinoids, and their anabolic/catabolic enzymes are present in cardiovascular tissues, and CB1 may contribute to the development of common cardiovascular disorders [83]. In the heart, there is strong evidence supporting a pathologically overactive endocannabinoid system (mainly CB1) in cardiometabolic disease [84], with endocannabinoids and exogenous cannabinoids exerting opposing effects on cardiovascular injury and inflammation. A consistently reliable acute action of marijuana is mild tachycardia, with increases cardiac output and correspondingly increases myocardial oxygen requirement. The increased myocardial load is problematic, if there is underlying coronary insufficiency [85]. Peripherally restricted CB2 agonists and CB1 antagonists are promising targets in cardiovascular disease [86].
Liver differentiation (but not heart, pancreas, or kidney differentiation) requires functional CB1 and CB2 receptor signaling. Cannabinoid receptor expression is normally low in the liver, with CB1 and CB2 receptors acting in opposite directions: CB2 receptors mediate several biological functions in various types of liver cells, and CB1 blockade contributes to beneficial metabolic effects [40]. CB1 expression increases in pathological states, and the receptor plays a critical role in liver disease. It promotes fibrogenesis, steatosis, and the cardiovascular complications of liver disease, whereas CB2 is protective, reducing these indices of liver dysfunction. Clinical studies of marijuana reveal its detrimental effects on the liver, presumably by activating the CB1. Peripherally restricted CB1 antagonists and CB2 agonists have therapeutic potential in the liver: activation of CB2 reduces pro-inflammatory cytokines, attenuates reperfusion injury, reduces fat accumulation, and is antifibrotic; CB2 agonists and peripherally restricted CB1 antagonists may benefit (non)alcoholic fatty liver diseases.
Endocannabinoids modulate the functional activities of immune cells, largely through CB2 receptors. Immune functions may be modulated through the interaction of ligands with the CB2 cannabinoid receptor, providing novel targets for therapeutic manipulation.
The endocannabinoid 2-AG controls skeletal muscle cell differentiation via CB1 receptor-dependent inhibition of Kv7 channels [87]. In adults, endocannabinoid signaling (largely through CB2 receptors) contributes to regulating energy metabolism in muscle and the formation of new muscle fibers.
Endocannabinoid signaling, primarily mediated by the CB2 receptor, regulates all critical stages of pregnancy and affects pregnancy events. Signaling is also involved in the preservation of normal sperm function and thus male fertility.
Endocannabinoid signaling, through both CB1 and CB2, is involved in regulating skin functions such as proliferation, differentiation, cell survival, immune responses, and suppressing cutaneous inflammation. Exogenous modulators of the receptors would clarify the role of the endocannabinoid system in hyperproliferative skin conditions, allergic, and inflammatory skin diseases.
Endocannabinoid signaling regulates bone elongation and bone remodeling by modulating bone cell proliferation, by communicating between bone cells, and by neuronal control of bone remodeling. THC has profound effects on murine bone growth.
The role of endocannabinoid signaling in respiratory tract and urinary system remains unclear. The recent vaping crisis in the United States has implicated THC in > 80% of the new cases of EVALI (e-vaping associated lung injury). It is well documented that pulmonary function is compromised by marijuana smoking. Whether THC alone or a different substance used in preparing vaping cartiges or pods is responsbile for the vaping crisis is unknown [88]. Preliminary evidence indicates that CB1 and CB2 receptors may contribute to kidney disease. Endocannabinoid signaling is also implicated in pancreatic development, with fetal endocannabinoids orchestrating the organization of pancreatic islet microarchitecture [89].
Circulating endocannabinoids respond to or reflect stressful conditions, and their concentrations are may be modulated in stress disorders, e.g., PTSD. At present, physiological regulation that contributes to circulating concentrations is too complex for using blood levels as a biomarker for a specific disorder. Circulating concentrations of 2-arachidonoylglycerol are circadian and dysregulated when sleep is disrupted. Other conditions under which circulating endocannabinoids are altered include inflammation, pain, and psychosis.
Disturbances of the Endocannabinoid System in the Brain
Every analysis of endocannabinoid tone, signaling, and relevance to disease states should consider marijuana use as a critical, confounding variable.
Rare genetic variants in the endocannabinoid system genes encoding the CB1 and CB2 receptors, as well as synthetic and metabolic enzymes (DAGLA, MGLL, FAAH), have been identified in genetic testing of a small number of patients (6032) with neurological disorders. Heterozygous rare coding variants in CNR1 gene (CB1 receptor) were significantly associated with pain sensitivity (especially migraine), sleep, and memory disorders – alone or in combination with anxiety – compared to controls without variants. These phenotypes are similar to those implicated in a “clinical endocannabinoid deficiency syndrome” theory. Heterozygous rare variants in DAGLA, which encodes diacylglycerol lipase alpha (synthesizes 2-AG), were significantly associated with seizures and neurodevelopmental disorders (autism, brain morphology abnormalities), compared to controls. The severe phenotypes associated with rare DAGLA variants underscore the critical role of rapid 2-AG synthesis and the endocannabinoid system in regulating neurological function and development [90].
A variant of FAAH (A385), which metabolizes and inactivates endocannabinoids, leads to reduced FAAH activity, resulting in increased AEA. This FAAH polymorphism in humans has been linked to threat and stress responses, manifest as reduced amygdala reactivity to threat possibly due to enhanced signaling. Stress exposure rapidly mobilizes FAAH to deplete anandamide and increase neuronal excitability in the amygdala. The FAAH variant A385 may sustain higher levels of AEA under stressful conditions.
The relevance of the endocannabinoid system to schizophrenia is through: 1. the abnormal levels of endogenous cannabinoids or receptors, 2. the association between marijuana use and the onset, course of illness, dose-dependent symptom severity, and 3. therapeutic potential of cannabidiol, a potential antipsychotic that elevates endocannabinoids. This segment summarizes the evidence for 1. In 5 studies (226 patients, 385 controls), significantly higher concentrations of anandamide were found in the CSF of patients than controls. In 9 studies (344 patients, 411 controls), anandamide levels were higher in blood of patients compared with controls. In 3 studies (88 patients, 179 controls), higher expression of CB1 was found on peripheral immune cells in patients compared with controls. Higher endocannabinoid tone was found at an early stage of illness in individuals who were antipsychotic naïve or free and an inverse association with symptom severity and was normalized after successful treatment. Testing clinically relevant markers of the endocannabinoid signaling elements in blood and CSF of people with psychotic illness may eventually provide useful biomarkers for the psychotic disorder and even insight into pathophysiological processes. However, as not all studies accounted for important variables, such as marijuana use, it is premature to assume that endocannabinoid dysregulation is a state or trait of the disease [91]. Nonetheless, higher endocannabinoid tone at an early stage of illness showed an inverse association with symptom severity, but normalized after treatments. Children with autism spectrum disorder had lower serum levels of anandamide, 2-arachidonoylglycerol, and their related endogenous compounds, N-palmitoylethanolamine and N-oleoylethanolamine, which were not associated with or correlated with age, gender, BMI, and medications.
Marijuana and the Brain: Receptors and Changes in Endocannabinoid Signaling
A comprehensive review of marijuana effects in the brain and behavior is beyond the scope of this chapter [92–105]. Marijuana can affect the brain at multiple levels, by physically altering molecular, cellular, function, circuitry, and morphology; by compromising cognition, motivation, learning, memory, coordination, sleep, and affect; and by engendering addiction and psychosis. Unless laboratory based, or constructed as a randomized controlled trial, current human marijuana research is challenging: THC doses consumed are rarely known unless chemical composition of each consumed product is tested, including the ratio of THC to CBD which varies widely, resulting in additive, neutral, or antagonistic pharmacological effects [106, 107]. Conclusions drawn from consumption of retail “marijuana” and self-reporting patterns of use are valid but lack the rigor needed to quantify the consequences of marijuana based on potency, purity, composition of matter, frequency of use and route of delivery. Also, polypharmacy is common among marijuana users, representing another potential confounding variable [108, 109]. At present, molecular observations are insufficient to develop a comprehensive model of the mechanisms underlying the behavioral, psychiatric, cognitive and psychological sequelae engendered by marijuana.
Cannabinoid synaptic adaptability is core to understanding the primary effects of marijuana in the brain and how these effects can trigger a cascade of biological changes that compromise learning, motor function, tolerance, and other brain functions. CB receptors are primary targets of THC, the principal cannabinoid in marijuana. Cannabinoid tolerance leading to diminished biological responses in isolated tissue develops over time and is attributable to loss of receptor function and CB1 receptor downregulation following chronic exposure to THC. CB1 receptor down-regulation has also been observed in a brain region-specific manner. In humans, non-invasive PET imaging has revealed CB1 receptor downregulation after chronic marijuana exposure [110]. Specificity of regions more responsive to downregulation was observed in marijuana-dependent subjects, with reductions largely in cortical areas. Receptor downregulation correlated with number of years marijuana was smoked but was reversible upon cessation. One possible interpretation of these findings is that receptor downregulation may function as a driver of compulsive marijuana use to restore equilibrium levels of CB1 activity. Adaptation is variable and can be as transient as modulation of endocannabinoid signaling or longer term, involving CB1 receptor internalization and downregulation or altered activity of enzymes involved in synthesis and metabolism of endocannabinoids. Longer time frame adaptive responses include the generation of new neurons from neural stem cells in the hippocampus of the adult brain, crucial functions attenuated by THC. CB2 downregulation is not as reproducible as CB1 and may be modulated similarly by both agonists and antagonists. At a more granular level not feasible for scrutiny in living human brain, THC induces expression of immediate early genes (zif268, pCREB, c-Fos) differentially in brain regions, primarily in the dopamine-rich striatum, hippocampus, and cortex [111]. Repeated THC exposure produces less induction of these factors in certain regions, possibly reflecting the development of tolerance. In contrast, CB1 receptors in the basal ganglia exhibit less plasticity than in other brain regions, possibly accounting for why humans show less tolerance to the subjective and motor effects of marijuana.
In rodents, acute marijuana exposure increases dopamine and opioid peptide release and attenuates GABA and glutamate release in the nucleus accumbens, leading to a rewarding effect. Cognitive function likely is compromised by reduced acetylcholine release in the hippocampus and prefrontal cortex. Other brain regions are also affected by introduction of THC. After repeated THC administration, three key changes conceivably drive impaired cognition, dysphoria/stress, and marijuana-seeking behavior: (1) CB1 receptors and function decline in many brain regions, especially in human and rodent cortex, even though declinations are not parallel in the two species. Memory and cognitive impairment is likely associated with the decline in CB1 receptor and impaired signaling in regions critical for learning and memory; (2) repeated exposure to THC also affects the magnitude of reward, as it downregulates dopamine signaling; (3) stress-related signaling (e.g., dynorphin, corticotropin-releasing factor) rises in the “emotional” amygdala, conceivably elevating dysphoria and increasing the compulsion to use the drug [94, 112]. Conventional approaches to investigating the biological targets of marijuana have traditionally been focused on “known zones” of commonly accepted critical brain regions and biological targets. Future preclinical research should incorporate more advanced approaches, using RNA-Seq and corresponding proteomics in multiple brain regions and within single neurons to clarify pathways most affected by a range of THC doses, THC:CBD ratios of marijuana, used acutely or repeatedly, by various routes (smoking, vaping, edibles) for various periods of time and at different age ranges. Ultimately, a comprehensive view of the full spectrum of marijuana’s biological consequences will be generated.
Conclusion
Marijuana is the third most commonly used drug in the United States among adults (after alcohol and tobacco) and the second most widely used substance by adolescents. The principal target of marijuana is the vastly influential endocannabinoid system, a signaling system that modulates a wide range of behavioral, cognitive, psychiatric, endocrine, and motor functions in the brain and peripheral tissues. Consumption of novel, high potency marijuana products has launched, a vast human experiment without informed consent.