17.1 Introduction
The importance of nutrition in critical illness has been increasingly recognized, and this is no different with neurological critical illness. Critical illness is associated with a catabolic state which is frequently associated with secondary complications such as increased infection rates, multiple organ dysfunction, prolonged intubation, further morbidity, and worsened mortality. Moreover, patients with neurological injury often have an associated dysphagia, poor mental status, and prolonged immobilization, putting them at further risk of malnutrition.
Nutritional support in neurological patients is a complex issue, dependent on such factors as a patient’s baseline metabolic status, severity and temporal progression of illness, use of mechanical ventilation, and use of sedatives. The appropriate dose of nutrition should be administered early in the hospital course once hemodynamic stability is established. Improper administration can lead to overfeeding, underfeeding, or poor glycemic control. However, traditional methods of assessing baseline energy expenditure may not apply to patients with neurological injury.
17.2 Metabolic Response to Injury (Fig. 17.1)
17.2.1 Inflammatory Response
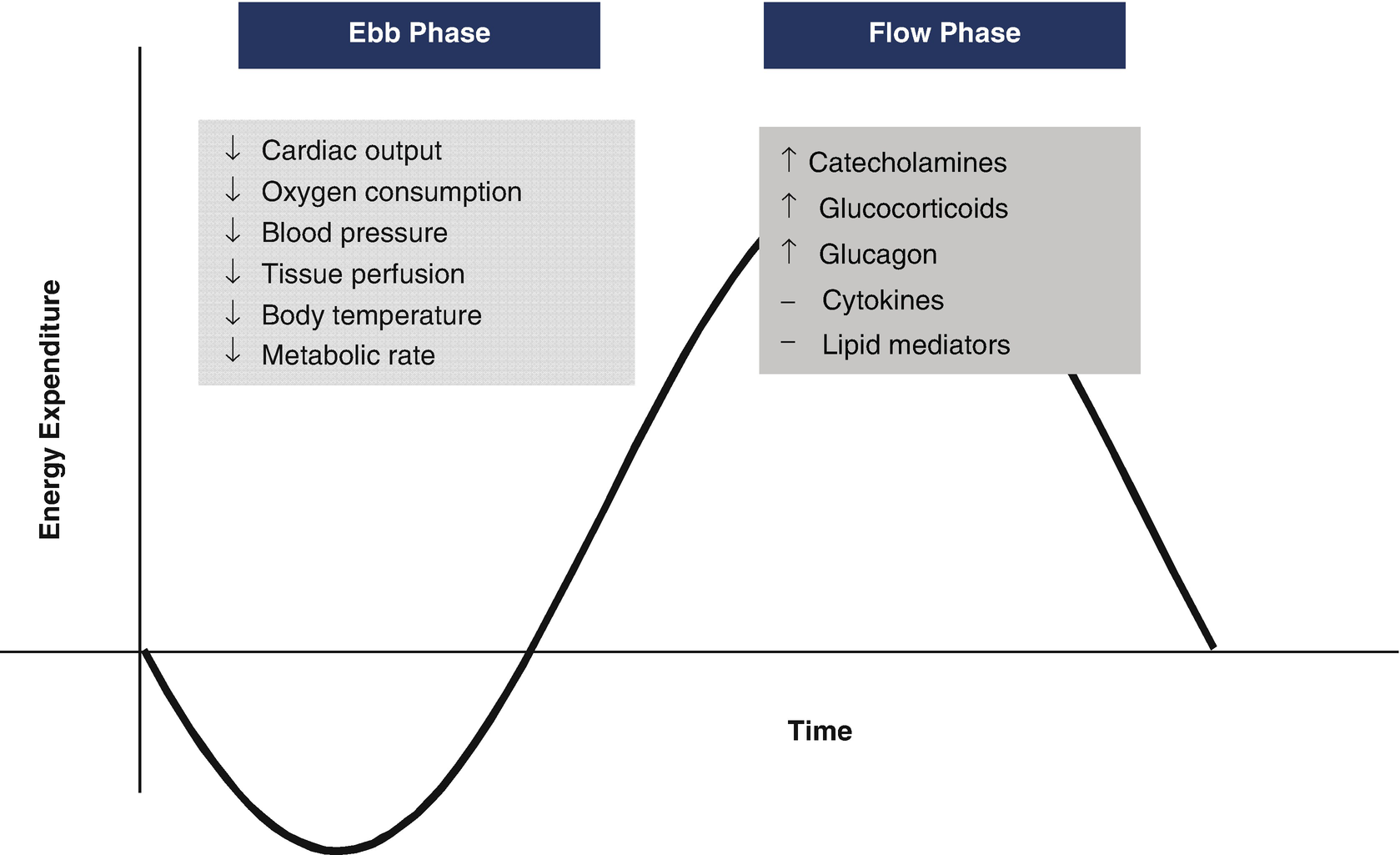
Metabolic response to injury. Characterization of the Ebb and Flow phases of metabolic response to injury. The primary response involves a decrease in metabolic rate, reflected by a decrease in cardiac output, oxygen consumption, and tissue perfusion. The next phase involves a ramp up in catecholamine activity and subsequent increase in metabolic rate. Adapted from: Cutherbertson DP, et al. Adv Clin Chem 1969;12:1-55
The inflammatory response implicated in the pathogenesis of cerebral ischemia and traumatic brain injury has been well studied [2, 3]. Cytokines and chemokines produced by immune cells from the periphery along with microglia, astrocytes, and neurons from the CNS mediate the inflammatory response. Interleukin-1β is a pro-inflammatory cytokine thought to be produced primarily in the brain within the first 24 h after injury. Studies have correlated increases in CSF IL-1β with increases in ICP and poorer outcome in TBI. In human studies, IL-6 has had more of a neuroprotective role and peaks after IL-1β, Although systemically it is associated with hyperpyrexia and anorexia [1]. Elevations in complement have been associated with blood-brain barrier dysfunction. Together, tumor necrosis factor alpha and IL-1 stimulate the release of interleukin 8 (IL8) from astrocytes. IL8 is chemokine that promotes neutrophil infiltration and is associated with increase blood-brain barrier permeability.
17.2.2 Substrate Utilization (Fig. 17.2)
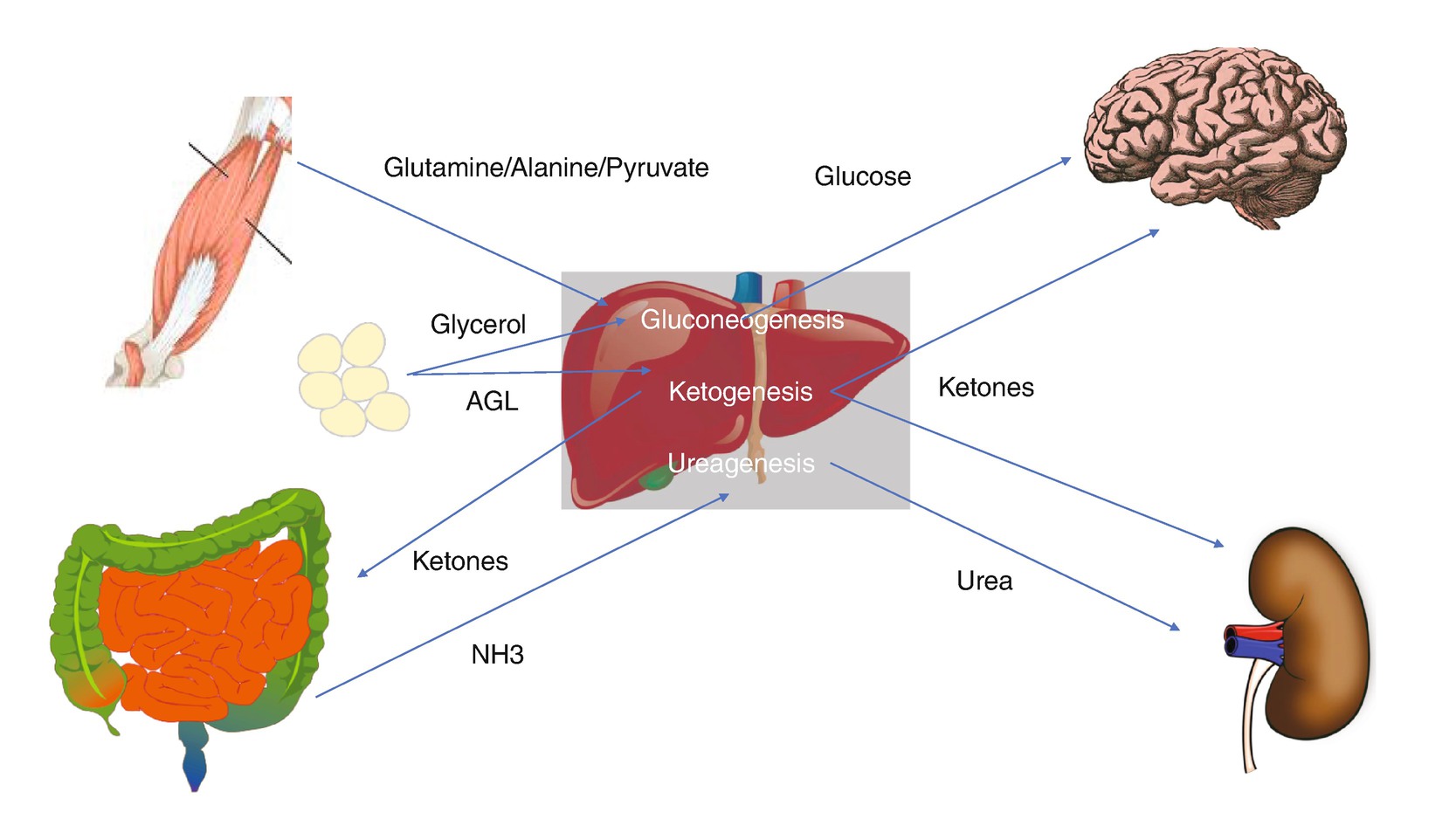
Substrate utilization in critical illness. Depiction of pathways and substrates involved in metabolism during injury
During relative hypoglycemia, the brain utilizes other substrates for energy such as ketones, lactate glycerol, and amino acids [6]. Ketone synthesis occurs in the liver through ketogenesis and is regulated by insulin, glucagon, and catecholamines. Ketones are then transported by monocarboxylate transporters (MCTs) for utilization. In the setting of brain injury, studies have shown increases in both vessel and neuronal monocarboxylate transporters, favoring transport of ketones to the brain. As previously mentioned, glutamate toxicity leads to a sequence of events that ultimately lead to neuronal death, and experiments in animals have shown reduction in neuronal damage with the use of ketone supplementation in the setting of glutamate-induced excitotoxicity indicating a potential neuroprotective effect [4].
Protein catabolism exceeds protein synthesis during the inflammatory response as indicated by a negative nitrogen balance. Amino acids, primarily alanine and glutamine, are then utilized in gluconeogenesis or oxidized and excreted in the form of urea and ammonia. As a result, critically ill patient will experience skeletal muscle depletion and lean body mass [1]. Currently ASPEN guidelines do not recommend glutamine supplementation in the general ICU population, but it is recommended in patient with TBI based on a clinical trial where patient with brain injuries was provided supplementation with glutamine and probiotics. This control group in the study that did not receive supplementation had longer lengths of stay in the ICU, more days of mechanical ventilation, and greater infection rate [7].
The role of lipolysis during critical illness is to produce glycerol from free fatty acids. Glycerol is then for both glucose and ketone production. These free fatty acids are susceptible to peroxidation by reactive oxygen species produced in the setting of mitochondrial dysfunction. This oxidative stress, defined as a state of imbalance between reactive oxygen species and nitric oxide synthetase, results in consumption of antioxidants and their stores and is associated with increased morbidity and mortality in the critically ill [8]. Studies have demonstrated low serum levels of antioxidants and altered levels of zinc, copper, and iron in brain injury and ischemic stroke [8, 9]. Despite an abundance of clinical and preclinical data suggesting micronutrients as potential therapeutic targets, no studies have demonstrated a clear benefit in their use as supplementation.
17.2.3 Brain-Gut Microbiome
There has been increasing interest in the brain-gut axis, a bidirectional physiologic model, as both a mechanism of insult in the CNS and potential target for therapy. Neuronal components include the autonomic nervous system, and the gastrointestinal component consists of the intestinal flora and wall [10]. Based on preclinical and clinical data, Sundman et al. hypothesized how disruption of the brain-gut axis created a positive feedback, causing secondary injury in traumatic brain injury. They propose that brain trauma creates a focal inflammatory response, producing microglial hypersensitivity. Additionally, dysautonomia from the head injury creates dysbiosis of the gut flora and compromise of the intestinal wall, allowing pathogens and harmful substance to enter the bloodstream; as a result, the hypersensitive microglial cells become neurotoxic, producing this state of perpetual neuroinflammation. Studies focusing on the influence of intestinal flora on ischemic stroke suggest that the intestinal flora may function as a modulator of the inflammatory response, like the hypothesis proposed in TBI. Additionally, the microbiota may contribute to the pathogenesis of ischemic stroke with the promotion as atherosclerotic plaque formation [11].
17.3 General Clinical Approach
17.3.1 Measurement of Caloric Requirements
Resting energy expenditure equations
Predictive equation | Population cohort |
---|---|
Harris and Benedict equation | Healthy volunteers (men) |
Mifflin equation | Healthy volunteers (men and women) |
Ireton-Jones equations | Burn patients |
Owen equation | Hospitalized patients |
Penn State equations (1998 and 2003) | Critically ill patients |
Swinamer equation | Critically ill patients |
When IC is not readily available, the REE can be estimated using various equations. The first calculations formulated are the Harris-Benedict equations. There have subsequently been numerous equations attempting to estimate the REE, with accuracies ranging from 40 to 75% when compared to the gold standard of indirect calorimetry [13]. The calculation of REE is more difficult in the ICU setting, as patients have wide fluctuations in temperature, use of sedatives, and varying levels of engagement with physical therapy. Even the use of the gold standard indirect calorimetry can be limited based on many ICU-specific patient factors, such as the use of chest tubes, supplemental oxygen sources, positive end-expiratory pressure, use of anesthesia, and continuous renal replacement therapy [14].
17.3.2 Assessment of Nutritional Risk (Fig. 17.3)


Examples of nutrition risk scores. (a) NUTRIC score. (b) NRS-2002
When IC is not readily available, there are recommendations for the use of published predicted equation, such as the Penn State or Harris-Benedict, or a weight-based equation of 25–30 kcal/kg/day can be used. However, these are inadequate given dynamic variables in critically ill patient like fevers and metabolic changes due to medication and have not been validated in the neurocritical care population [13, 16]. Other traditionally used methods such as serum biomarkers like albumin, prealbumin, transferrin, and retinol-binding protein are not adequately studied for monitoring of nutrition due to changes in acute-phase response [6].
17.3.3 Nitrogen Balance and Protein Provision
Aside from assessing general nutritional risk, measuring the adequacy of protein intake is important in the critically ill patient. This can be assessed by the nitrogen balance. Since critical illness is a catabolic process involving the breakdown of proteins into amino acids, this increases overall nitrogen expenditure and results in a negative nitrogen balance. Most of the nitrogen excreted by patients is in the form of urea nitrogen in the urine. Protein requirements in a critically ill patient range from 1 to 1.2 g/kg/day and may be higher in burn and multi-trauma patients.
Ultrasound presents an inexpensive and easily accessible modality to assess qualitative changes in skeletal muscle. Rapid skeletal muscle wasting is a common result from critical illness, which leads to poor functional outcome. Puthucheary et al. compared sequential histologic specimens and rectus femoris echogenicity and found that fasciitis and muscle necrosis can be readily detectable using ultrasound. These qualitative changes in critically ill patient may have implication in mobilization and rehabilitation [17]. A more widely accepted method to monitor protein energy metabolism is the measurement of nitrogen balance, and although not an optimal marker of total nutritional therapy, negative nitrogen balance has been associated with infectious complications and injury severity in several neurocritical care subgroups [16].
17.3.4 Enteral Nutrition
Starting enteral nutrition (EN) early during the neurocritically ill patient’s admission is important for combating the hypermetabolic status and preventing secondary complications. The 2016 SCCM/ASPEN guidelines recommend initiating EN within 24–48 h in patients who cannot maintain a proper volitional intake. This recommendation is based on a meta-analysis of multiple randomized trials comparing early to delayed EN that found improvements in mortality and infectious morbidity in the early EN group [13]. This adds on data that indicate that early EN is associated with reduced mortality [18], length of stay, and hospital-acquired infections [19].
There remains concern in initiating early EN in patients who are being treated with high-dose vasopressors, as these medications increase splanchnic vasoconstriction and decrease gastric motility [20]. For these patients, careful attention should be paid for signs of mesenteric ischemia or rising gastric residual volumes as an indicator of intolerance.
17.3.5 Parenteral Nutrition
Enteral nutrition is preferred over parenteral nutrition (PN) whenever possible due to the beneficial effects on normal gut flora growth, bowel motility, and normal balance of nutrient uptake. PN is only indicated in patients who cannot receive at least 60% of their energy and protein requirements via EN [13]. Recommendations on timing of when to initiate PN depend on the patient’s nutritional risk. In patients at low nutritional risk, PN should be withheld 7 days after admission and started only if EN remains unfeasible [13]. However, in patients with high nutritional risk as indicated by an NRS-2002 greater than five or NUTRIC greater than six, PN should be initiated as early as possible to reduce mortality and infection rates [21].
17.4 Nutrition for Specific Neurocritical Care Diagnoses
17.4.1 Traumatic Brain Injury
Traumatic brain injury (TBI) induces a significant hypermetabolic response that can last for up to 4 weeks after the initial injury [22]. The increase in metabolic rate is on par with that of burn patients with 20–40% of body surface area affected [23]. This response is likely due to the release of cytokines and counterregulatory hormones activated in the acute-phase response [24, 25]. An increased resting energy expenditure and protein catabolism make it difficult to maintain a positive nitrogen balance, which can last for up to 4 weeks after the initial injury [26].
Due to these physiological responses, the lack of appropriate feeding can lead to malnutrition and secondary complications. In addition, patients with sustained TBI commonly have feeding intolerance due to damage to neurologic pathways responsible for swallowing. Patients with moderate to severe TBI frequently require mechanical ventilation, necessitating enteral feeding. Early nutrition, once a patient is hemodynamically stable, is an important goal for these patients, with data showing that early nutrition within the first 5 days is associated with a lower rate of mortality, poor outcome, and infectious complications. There is inadequate data to support total parenteral nutrition versus enteral nutrition [27, 28].
Sparse data exists to recommend a specific formulation of nutrition support. Small studies have shown a potential benefit of a carbohydrate-free diet [29] as well as zinc supplementation, but there is a lack of confirmatory data, and larger trials are needed to determine the proper formulation and supplementation for patients with TBI. Currently, best practices are adopted from the general critical care literature.
17.4.2 Spinal Cord Injury
Unlike most neurological conditions, spinal cord injury (SCI) results in a decrease in resting energy expenditure [30]. The extent of this decrease is related to the level of the injury, with larger decreases observed in patients with upper spinal injuries. Failure to account for lower REE can lead to overfeeding and prolonged mechanical ventilation [31]. Other metabolic abnormalities include increased calcium excretion that peaks 3 weeks after injury resulting from immobilization and increased bone resorption. Oral intake can become a significant problem, with dysphagia being a common issue following SCI, particularly with cervical spine-injured patients. Percutaneous endoscopic gastrostomy (PEG) insertion may provide a safe alternative for these patients, although no data from controlled trials exists in this patient population to inform optimal timing for PEG insertion.
17.4.3 Acute Ischemic Stroke
Malnutrition is a common issue in the stroke population which associated with poor functional outcomes and increased length of stay in the hospital and rehabilitation setting. A large percentage of stroke patients present with dysphagia, which is not only a risk factor for malnutrition but also increases risk of complications such as aspiration pneumonia [32].
Feed or Ordinary Diet (FOOD) trial collaboration consisted of three separate studies addressing factors implicated in undernutrition within the stroke population [33, 34]. Two of the three trials involved dysphagic patients. The first trial compared early enteral feeding versus delayed feeding for at least 7 days. Results from this trial suggested that earlier feeding was better. In the second trial, the use of tube feeding via nasogastric versus percutaneous endoscopic gastrostomy which demonstrated that PEG feeding to be associated with an increased risk of death or poor outcome. The third study compared standard nutrition with oral nutrition supplementation with protein. No significant difference was noted between the groups in terms of poor outcome or death. Also, increased development of pneumonia, infection, pressure sores, and gastrointestinal hemorrhage was noted within population that was classified as malnourished [35].
17.4.4 Intracerebral and Subarachnoid Hemorrhage
Subarachnoid hemorrhage (SAH) results in a hypermetabolic state, with an increase in REE comparable to that seen in TBI. This catabolic state results in a net negative nitrogen balance that is associated with worse neurological outcome and increased hospital-acquired infections [36]. The degree of hypermetabolism is proportional to the clinical severity of SAH [37]. Additionally, injury severity and hypermetabolism are associated with higher n-free fatty acid (FFA) levels and an increase in the n6:n-3 FFA ratio, providing a potential therapeutic target to mediate the inflammatory response in SAH [38]. Another factor exacerbating poor nutritional status in SAH patients is the use of nimodipine for prevention of delayed cerebral ischemia, as this medication commonly causes diarrhea. The use of banana flakes or loperamide can help prevent this issue.
Despite the known risks of malnutrition, there are sparse data to recommend specific interventions to improve the nutritional status in SAH. A small, prospective randomized trial demonstrated that supplementation with 2700 mg/day of eicosapentaenoic to SAH patients who underwent for up to 30 days post clipping was shown to decrease both symptomatic vasospasm and cerebral infarction caused by cerebral vasospasm [39]. More studies are needed for specific recommendations to optimize the nutritional status of these patients.
17.4.5 Fulminant Hepatic Failure
Fulminant hepatic failure results in the well-recognized metabolic abnormalities of hypoglycemia, due to impaired gluconeogenesis, and hyperammonemia, due to reduced hepatic synthesis of urea and glutamate. The degree of hyperammonemia is proportional to the severity of the condition as well as the risk of cerebral edema and herniation [40].
Despite substantial loss of functioning hepatic cells, studies have shown increase in energy expenditure in fulminant hepatic failure. One series demonstrated an increase in energy expenditure in patients with acetaminophen-induced fulminant hepatic failure as compared with both healthy controls and patients actively undergoing liver transplantation during the anhepatic phase [41]. Another series confirmed this finding in a cohort of patients with more heterogenous causes of fulminant hepatic failure [42]. Malnutrition can have an especially detrimental impact on the prognosis after liver transplantation, as hypermetabolism and inadequate nutrition can lead to an increased lactate: pyruvate ratio, inducing a pro-inflammatory cytokine response rendering the patient at increased risk of systemic inflammatory response syndrome and multiorgan failure [43].
Despite the known risks of malnutrition, there are insufficient data from clinical trials to guide nutritional therapy. Most suggestions are extrapolated from animal and physiological data. ESPEN guidelines recommend the measuring energy expenditure for individual patients via indirect calorimetry [44]. Glucose provision is regarded as mandatory, with the administration of lipid preferred in the case of insulin resistance. Amino acid administration is not considered mandatory in the hyperacute phase but should be used in the acute and subacute phase.
17.4.6 Coma After Cardiac Arrest
While there is sparse data on the metabolic response to cardiac arrest, most studies have focused on the metabolic implications during therapeutic hypothermia. While active cooling reduces cerebral metabolic demand, a small series of patients receiving hypothermia demonstrated a higher than expected resting energy expenditure. This expenditure rose proportionally with body temperature during active rewarming. There was also a significant association between energy deficit and ICU length of stay in patients with a good neurological outcome, indicating that these patients may have been malnourished [45]. Despite concern for decreased gastric emptying and high gastric residuals, enteral feedings appear to be safe. A small observational study of patients undergoing therapeutic hypothermia for cardiac arrest showed that 72% of enteral feeds are tolerated during cooling [46]. Another small cohort of ICH patients undergoing active hypothermia showed no GI-related adverse effects to enteral feeds [47].
17.5 Conclusion
The nutritional considerations in the neurocritical care population are complex. Despite the various pathophysiological mechanisms of neurocritical care conditions, early and adequate nutrition remains an imperative component of care. Adequate nutrition appears to blunt the secondary complications that can occur with under- or overfeeding, and accurate measurement of patients’ metabolic demands remains important. Overall, acute neurological injury creates a distinctive challenge for nutritional therapy and compels a careful assessment of the patient’s underlying mechanism of injury and comorbidity.
Key Points
Critical illness is associated with a catabolic state which is frequently associated with secondary complications such as increased infection rates, multiple organ dysfunction, prolonged intubation, further morbidity, and worsened mortality.
The appropriate dose of nutrition should be administered early in the hospital course once hemodynamic stability is established.
Improper administration can lead to overfeeding, underfeeding, or poor glycemic control.
Adequate nutrition appears to blunt the secondary complications that can occur with under- or overfeeding, and accurate measurement of patients’ metabolic demands remains important.