Chapter 2
Types of Polymers
The large number of natural and synthetic polymers has been classified in several ways: thermal behavior, route of chemical synthesis, and structural organization. These will be outlined below, and in the process many terms important in polymer science and technology will be introduced.
2.1 Reaction to Temperature
The earliest distinction between types of polymers was made long before any concrete knowledge of their molecular structure. It was a purely phenomenological distinction based on their reaction to heating and cooling.
2.1.1 Thermoplastics
It was noted that certain polymers would soften upon heating and could then be made to flow when a stress was applied. When cooled again, they would reversibly regain their solid or rubbery nature. These polymers are known as thermoplastics. By analogy, ice and solder, though not polymers, behave as thermoplastics.
Some of the most commercially important thermoplastics include polyethylene (PE), polypropylene (PP), poly(vinyl chloride) (PVC), and polystyrene (PS). PE is used in products ranging from plastic bags to detergent bottles and has the simplest possible repeat structure of any polymer, since all the pendant groups are hydrogens. PP is also found in a wide range of products, such as plastic storage containers, and competes with other polymers in making plastic bags and pipes. PVC is commonly found in materials as diverse as rigid drain pipes, shower curtains, and raincoats, while PS has been used in foam coffee cups and disposable cutlery. Because these materials are thermoplastics, they are typically made into pellets after polymerization, and then can be melted and extruded or shaped into final products.
2.1.2 Thermosets
Other polymers, although they might be heated to the point where they would soften and could be made to flow under stress once, would not do so reversibly; that is, heating caused them to undergo a “curing” reaction. Sometimes these materials emerge from the synthesis reaction in a cured state. Further heating of these thermosetting polymers ultimately leads only to degradation (as is sometimes attested to by the smell of a short-circuited electrical appliance) and not softening and flow. Again by analogy, eggs and concrete behave as thermosets. Continued heating of thermoplastics will also ultimately lead to degradation, but they will generally soften at temperatures below their degradation point.
Commercially important thermosets include epoxies, polyesters, and phenolic resins. Each of these materials starts out as (often viscous) liquids that set by curing into a final shape. Because these materials set the first time they are made, they cannot be reheated after the polymer is formed without degrading the structure.
Natural rubber is a classic example of the difference between a thermoplastic and a thermoset. Introduced to Europe by Columbus, natural rubber did not achieve commercial significance for centuries; because it was a thermoplastic, articles made of it would become soft and sticky on hot days. In 1839, Charles Goodyear discovered the curing reaction with sulfur (which he called vulcanization in honor of the Roman god of fire) that converted the polymer to a thermoset. This allowed the rubber to maintain its useful properties to much higher temperatures, which ultimately led to its great commercial importance.
2.2 Chemistry of Synthesis
Pioneering workers in the field of polymer chemistry soon observed that they could produce polymers by two familiar types of organic reactions: condensation and addition. As monomers react, new structures are created during the polymerization:
An oligomer is simply a small polymer, but it has unique properties since it has a small number of repeat units. Eventually, enough reactions take place to form a large polymer, where it is not uncommon to have thousands or tens of thousands of repeat units connected along a single polymer backbone. In the formation from monomer to polymer, the basic repeat unit remains the same, but the material changes from a monomer that is liquid (or even a gas) to oligomers that may be highly viscous liquids to solid polymers.
Several elementary organic functional groups are worth reviewing, as they play important roles in the synthesis of polymers (Figure 1.3). Because the chemical structures resulting from condensation and addition polymerizations are a bit different, the method of polymerization is one of the major distinctions used in describing polymers. Note that certain functional groups are reactive for forming polymers (common ones include alkenes, alcohols, carboxylic acids, and amines), while others are more commonly found in the resulting structures (alkanes, amides, esters, imides, and urethanes). Other functional groups may not participate in reactions, but be present as side groups or within the polymer backbone (e.g., aryl groups, and ethers).
2.2.1 Condensation Polymerization
Polymers formed from a typical organic condensation reaction, in which a small molecule (most often water) is split out, are known, logically enough, as condensation polymers. The common esterification reaction of an organic acid and an organic base (an alcohol in this case) illustrates the simple “lasso chemistry” involved:
The –OH group on the alcohol and the on the acid are known as functional groups, those parts of a molecule that participate in a reaction, while R and R′ are abbreviations that represent the remainder of the molecule––they are generic organic groups and will commonly be used in organic chemistry. Of course, the ester formed in the preceding reaction is not a polymer because we have hooked up only two small molecules, and the reaction is finished far short of anything that might be considered “many membered.”
At this point, it is useful to introduce the concept of monomer functionality. Functionality is the number of bonds a mer can form with the other mers in a reaction. In condensation polymerization, it is equal to the number of (organic) functional groups on the mer. These groups must be reactive (such as carboxylic acids, alcohols, aldehydes, or amines).
In the above example, the reactants are monofunctional (there is one alcohol group on one reactant and one carboxylic acid group on the other); thus, if there are no other functional groups present in the rest of the molecule (the R group), the reaction will form only one new bond (an ester). Consider, though, what happens if both reactants are difunctional and the reaction progresses at each end.
Here, the resulting product molecule is still difunctional (an alcohol on the left end and an acid on the right; the ester bonds, , are not reactive for polymerization). So, the left end of the molecule can react with another diacid molecule and its right end with another diol molecule. At each step, the growing molecule remains difunctional, so the reaction can continue until all alcohol and acid groups in the reaction mixture are added together (this includes the possibility that two larger molecules can react). Once enough of these molecules (hundreds to even millions of “mers”) have condensed together, a polymer is created.
2.2.1.1 Polyesters
In general, the polycondensation of x molecules of a diol with x molecules of a diacid to give a polyester molecule is written as in the reaction above.
In the polyester (many ester “mers”) molecule, the structure in brackets is the repeating unit, and this is what distinguishes one polymer from another. The linkage characterizes all polyesters. The generalized organic groups R and R′ may vary widely (with a consequent variation in the properties of the polymer), but as long as the repeating unit contains the
ester linkage, the polymer is a polyester. Of course, there are other functional groups that can react, and thus there are many different types of condensation polymers (polyamides, polyimides, polyethers, etc.).
The quantity x is the degree of polymerization, the number of repeating units strung together as identical beads in the polymer chain. It is sometimes also called the chain length, but it is a pure number, not a measurable length.
The above nomenclature was introduced by Wallace Hume Carothers, who, with his group at Du Pont, invented two important polymers, neoprene (an addition polymer) and nylon, in the 1930s and was one of the founders of modern polymer science 1.
2.2.1.2 Polyamides (Nylons)
Another functional group that is capable of taking part in a condensation polymerization is the amine (–NH2) group, where one hydrogen from this group reacts with a carboxylic acid in a manner similar to the alcoholic hydrogen to form a polyamide (commonly known as nylon):
The linkage is characteristic of all nylons. This bond is also commonly referred to as a peptide bond when it connects two amino acids. Proteins are made from polypeptides, which are one type of natural polymers, and are discussed later.
2.2.1.3 Polyimides
Polyimides are actually a subset of polyamides, but forms when both carboxylic acid groups in diacid monomer react with an amine. As shown, a benzene ring with two acid groups on adjacent carbons (in the ortho position) condenses with an amine to form a single bond. The result is an imide linkage.
Compare the functionality of the diacid in this reaction versus the polyamides or polyesters. Here, the diacid acts as a monofunctional group, while when forming polyamides or polyesters, the diacid reacts separately on both ends. For the formation of a polyimide, the carboxylic acid groups must be close together. Of course, this forms only a single bond. To form a polyimide, there needs to be another diacid as part of the R1 functional group and another amine in the R2 group.
2.2.1.4 Polyurethanes
Polyurethanes are made by the reaction of a molecule with two isocyanate groups (a diisocyanate) and two alcohols (a diol). In this reaction, the hydrogen from the alcohol is transferred to the nitrogen atom of the isocyanate group, and a new bond is formed between the isocyanate carbon and the alcohol oxygen.
As observed for the polyesters, polyamides, and polyimides, polyurethanes also have a characteristic bond, –(O–C–N)–, in their backbone (with the carbon having a =O) that makes these polymers easily identifiable. Note that after the reaction to form a urethane bond, the end groups (an alcohol and an isocyanate) are still available to grow the polymer chain further.
2.2.1.5 Polycarbonates
One additional group of condensation polymers highly important in industry is polycarbonates. Polycarbonates are a special subcase of polyesters, in that one monomer has only a single carbon, resulting in the characteristic linkage:
A common reaction to produce polycarbonates involves a diol (bisphenol-A) and phosgene, which is a diacid chloride (with functionality similar to a dicarboxylic acid) (see part N in Example 2.4).
2.2.1.6 Monomers with Tri- (or Higher) Functionality
The above examples are for polymers formed from difunctional reactants. If either reactant has only one functional group, no polymer can result. However, monomers with functionalities higher than two are also used to make polymers. For example, glycerin is a trifunctional molecule (three alcohol groups) that can be used in polyesterification. Molecules with three or more functional groups tend to make network (or cross-linked) polymers, as the reaction can proceed to form a three-dimensional network instead of just a linear polymer as difunctional monomers do.
2.2.1.7 Monomers with Two Different Functional Groups
It is possible that the starting molecule for a condensation polymerization has two (or more) different functional groups and does not require the presence of two different monomers. Two examples of such monomers are amino acids and hydroxy acids:
Of course, most of us have heard of amino acids in biology classes, as these are the building blocks for polypeptides, which are given the “poly” prefix because they are polymers of amino acids. Multiple polypeptides make up the structure of proteins. An amino acid can react with another amino acid to form an amide bond (or peptide bond if it is an α amino acid) because they have both an amine functional group and a carboxylic acid functional group. Similarly, hydroxy acids condense to form polyesters. However, these reactions may not always proceed in a straightforward fashion. If the R group is large enough, it is possible that the molecule becomes configured so that it can “bite its own tail,” condensing to form a cyclic structure rather than a polymer.
This cyclic compound can then undergo a ring-scission polymerization, in which the polymer is formed without splitting out a small molecule (e.g., water), because the small molecule had been eliminated previously in the cyclization step.
Despite the lack of elimination of a small molecule in the actual polymerization step, the products can be thought of as having been formed by a direct condensation from the monomer and are usually considered condensation polymers. Also of note is that not all condensation polymerizations yield water as the by-product, sometimes carboxylic acids are formed into acid chlorides (with the functional –OH group replaced by a chlorine atom), so instead of an –OH leaving group, a –Cl group leaves, resulting in HCl as the “condensation” by-product.
Note that in this case, the characteristic nylon linkage, , is split up in the above reaction, and not immediately obvious in the repeating unit as written. If you place a second repeating unit next to the one shown, it becomes evident. This illustrates the somewhat arbitrary location of the brackets, which should not obscure the fact that the polymer is a nylon (though the structure inside the brackets is the repeating “mer”). One important difference between typical synthetic polymers and biological polypeptides is that in most synthetic polymers, the R group is the same throughout the polymer, whereas polypeptides are made up of a sequence of 20 different amino acids (as coded by DNA), each with a unique R group.
Though not formed from a monomer with two different functional groups, another important class of natural polymers is polysaccharides (literally poly sugars). The most common monomer here is glucose (C6H12O6), and when bonded together by glycosidic linkages (a condensation reaction), it forms starch, cellulose, and other polymers that are important for structural rigidity (particularly in plants) and energy storage in living organisms, as most starches can be broken down (depolymerized) easily.
2.2.2 Addition Polymerization
The second polymer formation reaction is known as addition polymerization and its products as addition (or chain or free radical) polymers. Addition polymerizations have two distinct characteristics:
Monomers of the general type undergo addition polymerization as
The double bond “opens up,” forming bonds to other monomers at each end, so a carbon–carbon double bond is difunctional according to our general definition. (Note that a monomer with two double bonds has a functionality of four; this type of monomer is often referred to as a cross-linking agent as it results in the formation of network structures.) The question of what happens at the ends of addition polymers will be deferred until we reach Chapter 9 on polymerization mechanisms. Also, note that carbon–oxygen double bonds, while common in monomers and polymers, are stable and unreactive for addition polymerization.
An important subclass of the double bond containing monomers is the vinyl monomer:
Addition polymerization is occasionally referred to as vinyl polymerization. Table 2.1 lists some commercially important vinyl monomers, with different substituents replacing X.
Table 2.1 Some Commercially Important Vinyl Monomers.
Monomer | −X |
Ethylene | −H |
Styrene | ![]() |
Vinyl chloride | −Cl |
Propylene | −CH3 |
Acrylonitrile | ![]() |
Acrylic acid is a vinyl monomer with a double bond (since it has only one carboxylic acid, it would not be useful in a condensation polymerization). It undergoes addition polymerization to form
Interestingly, the ester linkage in poly(lactic acid), PLA, is easily hydrolyzed, breaking down the polymer. Thus, this polymer has found applications in biodegradable plastics for products ranging from food packaging to tissue engineering scaffolds for medical applications. Poly(acrylic acid), or PAA, is not degradable, but the pendant carboxylic acid group makes this polymer extremely hydrophilic and thus it is used as a superabsorbent (particularly important in diapers).
Although most molecules with two C=C bonds (dialkenes or simply dienes) have a functionality of 4 for addition polymerization, in conjugated dienes, the two double bonds are separated by only one single C–C bond, having a functionality of 2, so they form linear polymers. A conjugated diene is a unique monomer for polymerization because (unlike molecules where the double bonds that act as cross-linking agents are further separated) the double bonds here act in the same way as a single double bond in vinyl polymerizations.
The addition polymerization of a conjugated diene results in an unsaturated polymer, or a polymer that contains double bonds, either in the backbone of the polymer or in the pendant group. Furthermore, conjugated diene polymerizations can result in several structural isomers in the polymer. If the monomer is symmetrical with regard to substituent groups on carbons one through four, it can undergo 1,2-addition and 1,4-addition. If the monomer is asymmetrical, the 3,4 addition will result in yet another structural isomer. (For symmetrical dienes, the 1,2 and 3,4 reactions result in the same structure.) This is illustrated below for the addition polymerization of isoprene (2-methyl-1,3-butadiene), an asymmetrical conjugated diene:
Polyisoprene is an important flexible rubbery material, one of the first rubbers to be used as it is found in nature (thus the alternate name “natural rubber”). It is used in belts and hoses in automobiles, in laboratory gloves, and in rubber bands. The 1,2 and 3,4 reactions are sometimes known as vinyl addition, because part of the diene monomer simply acts as an X group in a vinyl monomer.
2.3 Structure
As an appreciation for the molecular structure of polymers was gained, three major structural categories emerged. These are illustrated schematically in Figure 2.1.
Figure 2.1 Schematic representation of polymer structures.
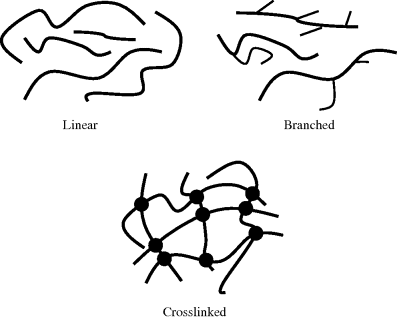
2.3.1 Linear
If a polymer is built from strictly difunctional monomers, the result is a linear polymer chain. A scale model of a typical linear polymer molecule made from 0.5 cm diameter clothesline rope would be about 3 m long. This is not a bad analogy: the chains are long, flexible, and essentially one-dimensional structures. The term linear can be somewhat misleading, however, because the molecules do not necessarily assume a geometrically linear conformation as shown in the figure. Some of the better analogies for what these macromolecules look like is a bowl of spaghetti or tangled strands of yarn. The nomenclature for homopolymers usually simply puts “poly” before the name of the repeat unit, which is particularly simple for most addition polymers, such as in polystyrene. If the repeat unit has more than one word, parentheses are used to indicate what is repeated, such as in poly(vinyl chloride). Many condensation polymers would have fairly complex names using this rule, so most are simply referred to by their class (e.g., a polyester) or by their trade names (Dacron®, Nylon®, Lexan®, etc.).
2.3.1.1 Random Copolymers
Polymers consisting of chains that contain a single repeating unit are known as homopolymers (this includes many polymers made by addition and condensation polymerization). If, however, the chains contain a random arrangement of two separate and distinct repeating units, the polymer is known as a random or statistical copolymer, or simply copolymer. A random copolymer might be formed by the addition polymerization of a mixture of two different vinyl monomers A and B (the degree of “randomness” depends on the relative amounts and reactivities of A and B, as we shall see later) and can be represented as
and called poly(A-co-B), where the first repeating unit listed is the one present in the greater amount. For example, a random synthetic rubber copolymer of 75% butadiene and 25% styrene would be termed poly(butadiene-co-styrene). Of course, ter- (3-) and higher multipolymers are possible: nature makes a regular tetrapolymer (RNA and DNA, which are complex polymers with four bases arranged according to genetic codes) and polypeptides that are copolymers with 20 different repeat units (amino acids).
It must be emphasized that the products of condensation polymerizations that require two different monomers to provide the necessary functional groups, for example, a diacid and a diamine, are not copolymers because they contain a single repeating unit. If, however, two different diamines were used in the polymerization, there would be two distinct repeating units and this could be correctly termed a copolymer.
2.3.1.2 Block Copolymers
Under certain conditions, linear chains can be formed that contain long contiguous blocks of two (or more) repeating units combined in the chains termed a block copolymer.
These structures are called a diblock copolymer, poly(A-b-B), and a triblock copolymer, poly(A-b-B-b-A), respectively. Here, the b (meaning “block”) replaces “co” to indicate the organized structure. These structures are important for nanotechnology as several block copolymers can self-assemble into nanospherical micelles, with the A and B blocks arranged to form the core and shell (Figure 2.2). This works especially well if the A and B groups have opposite polarities, so they prefer to segregate rather than intermingle.
Figure 2.2 Block copolymers self-assembling into a micelle with core of B groups and corona of A groups.
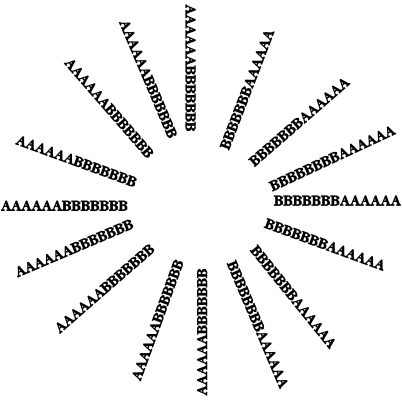
2.3.2 Branched
If a few molecules of tri (or higher) functionality are introduced (intentionally or through side reactions) to the reaction, the resulting polymer will have a branched structure. One such example is the grafting of branches made from repeating unit “B” to a linear backbone of A repeating units. Here, B is said to be grafted onto A:
This graft copolymer would be termed poly(A-g-B), as the backbone repeat unit is listed first. Note that “few molecules” is key here, since if even as little as 0.1% of the reaction mixture contains monomers with functionalities of 3 or higher, a network or cross-linked structure is likely to form (particularly at higher reaction conversions).
2.3.3 Cross Linked or Network
As the length and frequency of the branches on polymer chains increase, the probability that the branches will ultimately reach from one backbone chain to another increases. When the backbone chains are connected in this way, the molecular structure becomes a network, with all the chains linked through covalent bonds. This creates a three-dimensional cross-linked polymer and the entire mass of the polymer becomes one single tremendously large molecule! One impressive example is a bowling ball, which is a cross-linked polymer, so the entire mass is one molecule; it has a molecular weight on the order of 1027 g/mol. Remember this the next time someone suggests that individual molecules are too small to be seen with the naked eye.
Cross-linked or network polymers may be formed in two ways: (1) by starting with reaction masses containing sufficient amounts of tri- or higher functional monomer, or (2) by chemically creating cross-links between previously formed linear or branched molecules (“curing”). The latter is precisely what vulcanization does to natural rubber, and this fact serves to introduce the connection between the phenomenological “reaction to temperature” classification and the more fundamental concept of molecular structure. This important connection will be clarified through a discussion of bonding in polymers.
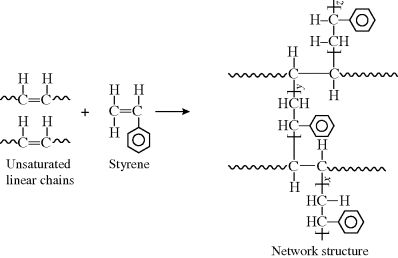
Part Polymer Name | Monomer Structure(s) | |
A Polystyrene | ![]() |
|
B Polyethylene | ![]() |
|
C Poly(butyleneterephthalate) (PBT) | ![]() |
![]() |
D Poly(ethylene terephthalate) (PET) (Dacron®, Mylar®) | ![]() |
![]() |
E Nylon 6/6 (the numbers designate carbon atoms in the diamine and diacid, respectively) | ![]() |
![]() |
F Nylon 6 (made from a single monomer) | ![]() |
|
G Glyptal (glycerol + phthalic anhydride) | ![]() |
![]() |
H Poly(diallyl phthalate) | ![]() |
|
I Melamine-formaldehyde (Melmac®, Formica®) | ![]() |
![]() |
J Polytetrafluoroethylene (Teflon TFE®) | ![]() |
|
K Poly(phenylene oxide) (PPO) (Hint: polymerized in presence of O2) | ![]() |
|
L Polypropylene | ![]() |
|
M Acetal (polyformaldehyde or polyoxymethylene) (Celcon®, Delrin®) | ![]() |
![]() |
N Polycarbonate (Calibre®, Lexan, Makrolon®) | ![]() |
![]() |
O Epoxy or phenoxy | ![]() |
![]() |
P Poly(dimethyl siloxane) (silicone rubber) (Hint: polymerized in presence of H2O) | ![]() |
|
Q Polyurethane | ![]() |
![]() |
R Polyimide | ![]() |
![]() |
S Polysulfone | ![]() |
![]() |
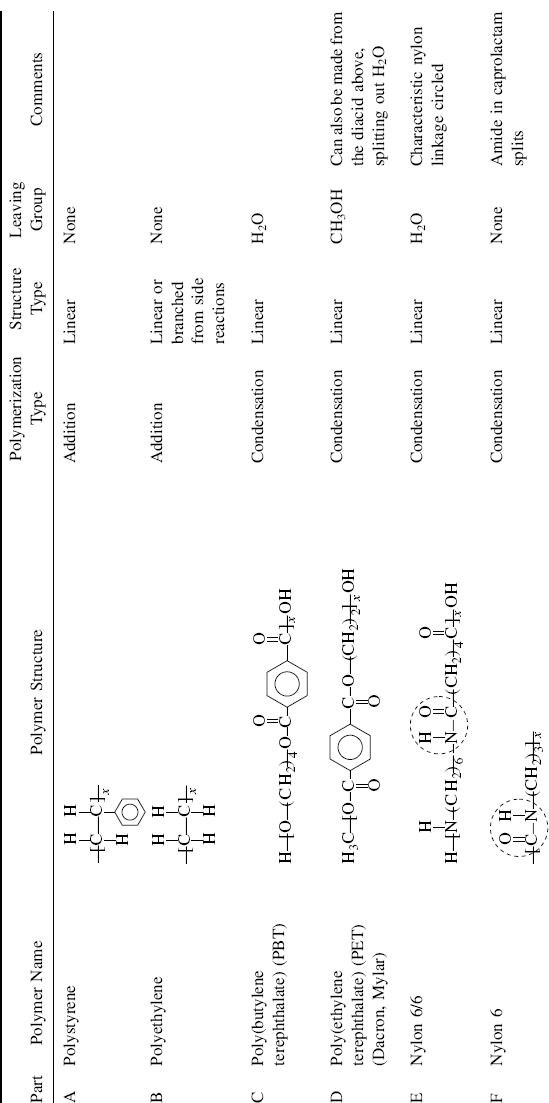
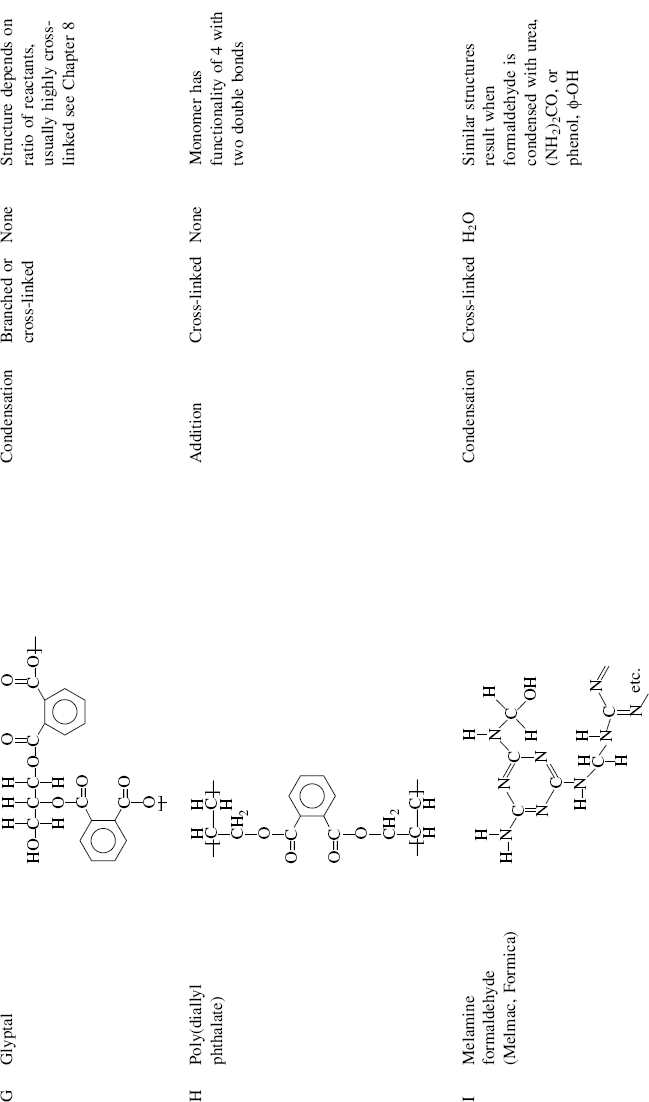
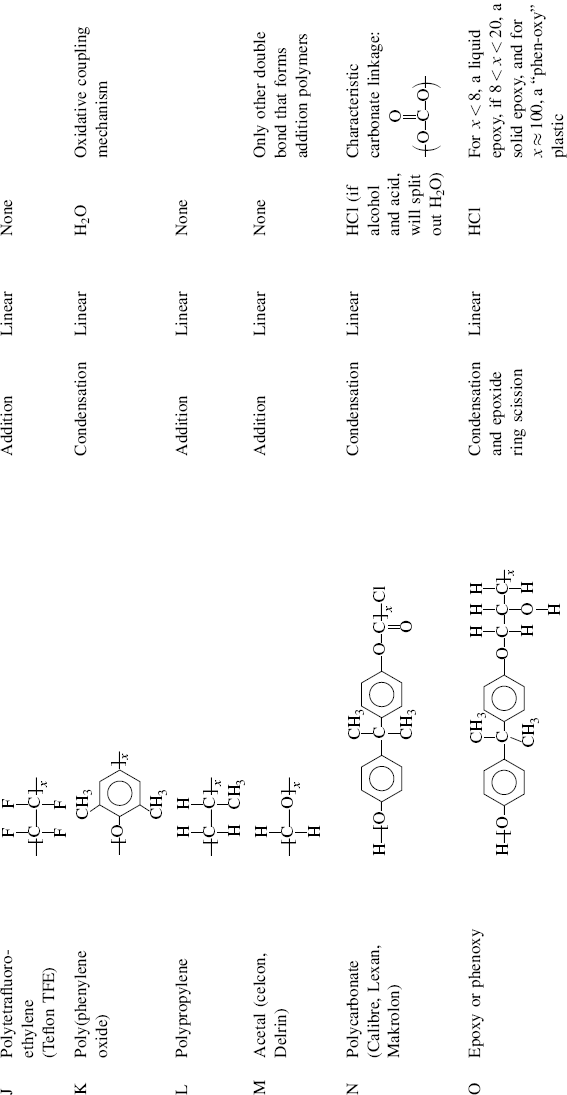
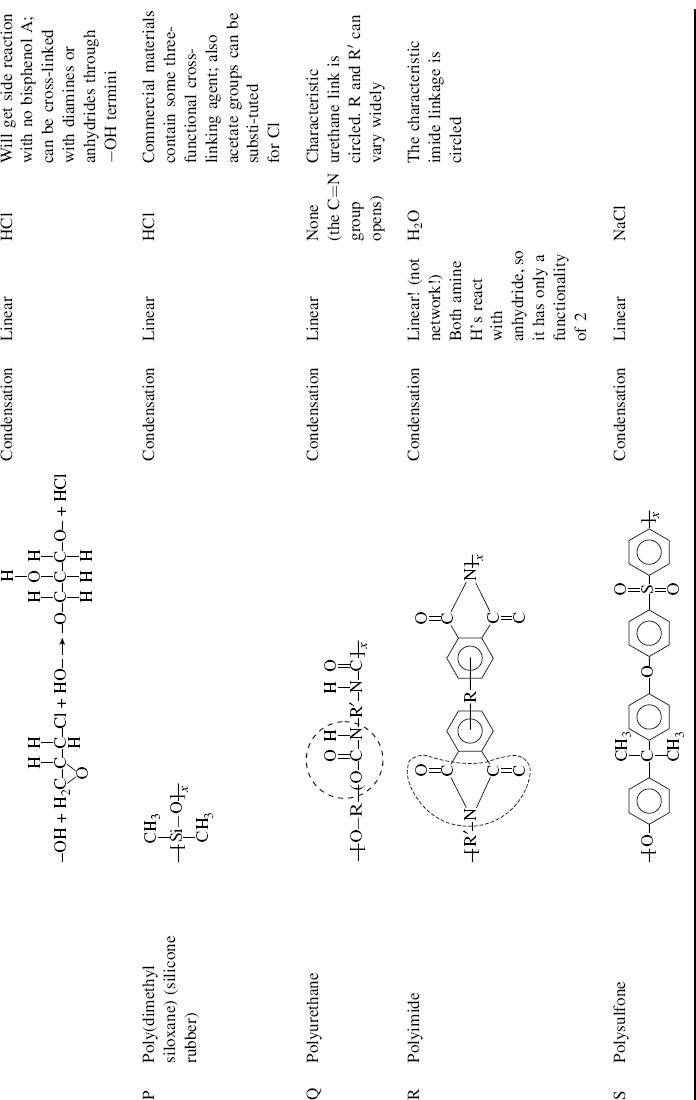
Table 2.3 Examples of the Versatile Applications of Polymers.
Bulletproof vests |
Food containers and bottles |
Furniture cushions and upholstery |
Carpeting and vinyl flooring |
Pharmaceutical coatings (e.g., gelcaps) |
Paints (latex and oil-based) |
Clothing (nearly all types) |
Chewing gum |
Sports equipment |
Wetsuits |
Shatterproof windshields |
Casing for electronics |
2.4 Conclusions
The division of polymers along the three categories discussed in this chapter included response to temperature (thermosets versus thermoplastics), polymerization mechanism (condensation versus addition), and structural organization (linear, branched, or network). Although these are very handy distinctions used to describe a polymer, we will see in further chapters that in some cases many polymers behave in a similar way despite having a variety of compositions and structures, there are many variables (molecular weight, additives, thermal processing history, etc.) that yield a wide range of properties for any one polymer. This wide range of properties allows polymers to be used in numerous applications (Table 2.3), as we will discover. The applications for the specific polymers in Example 2.4 are left as a homework exercise.
Problems


A visit to the website for the Macrogalleria may be helpful for most (if not all) of these polymers.
Notes
1. Although aromatic rings are often symbolized by , this is a poor representation of resonance-stabilized structures, which are completely inert to addition polymerization. They are more properly symbolized by
to avoid confusion with the ordinary double bond. To save space in this book, we will sometimes also use ϕ to represent the aromatic (or phenyl) ring.
1. Hounshell, D.A. and J.K. Smith, Jr., Invention and Technology, Fall 1988, p. 40.