1 Introduction
Around 30% of the world’s freshwater resources are stored underground in the form of groundwater (shallow and deep groundwater basins up to 2,000 m, soil moisture, swamp water, and permafrost). This constitutes about 97% of all the freshwater that is potentially available for human use [1].
Groundwater is the lifeline for many rural populations around the globe and a cornerstone of global food production. Groundwater constitutes nearly half the world’s drinking water and much of the world’s irrigation water supply. Population growth, overexploitation, salinization, nonpoint source pollution from agricultural activities, degradation of surface water, and groundwater quality and quantity conflicts at the urban–rural interface have reached global dimensions and threaten water availability and food security [3].
Groundwater irrigation worldwide [4]
Region | Area (Mha) | Volume used | |
---|---|---|---|
km3/a | % | ||
Global Total | 112.9 | 545 | 43 |
South Asia | 48.3 | 262 | 57 |
East Asia | 19.3 | 57 | 34 |
South East Asia | 1.0 | 3 | 5 |
Middle East and Central Asia | 11.9 | 76 | 38 |
Europe | 7.3 | 18 | 38 |
North Africa | 2.5 | 16 | 24 |
Sub-Saharan Africa | 0.4 | 2 | 7 |
North America | 19.1 | 100 | 54 |
Latin America | 2.2 | 88 | 19 |
Australia | 0.9 | 3 | 21 |
Groundwater provides drinking water to at least 50% of the global population and accounts for 43% of all water used for irrigation [4]. Groundwater also sustains the base flows of rivers and important aquatic ecosystems. Uncertainty over the availability of groundwater resources and their replenishment rates pose a serious challenge to their management and in particular to their ability to serve as a buffer to offset periods of surface water scarcity [5]. Groundwater supplies are diminishing, with an estimated 20% of the world’s aquifers being over-exploited [6, 7] leading to serious consequences such as land subsidence and saltwater intrusion in coastal areas [8]. Groundwater levels are declining in several of the world’s intensely used agricultural areas and around numerous mega-cities.

Competing water uses in the different sectors [2]. Source: World Water Development Report, www.unesco.org/water/wwap/facts_figures/water_industry.shtml

Total renewable water per capita (2013) and water scarcity [11]. Source: WWAP, with data from the FAO AQUASTAT database (http://www.fao.org/nr/water/aquastat/main/index.stm) (aggregate data for all countries except Andorra and Serbia, external data), and using UN-Water category thresholds. Note: The figures indicate total renewable water resources per capita in m3

Renewable water in the Arab Countries (2011) shows the water scarcity in Egypt [12]. Source: UNESCWA, prepared with data from FAO AQUASTAT. Note: The statistics for the year 2011 cover Sudan and South Sudan, as South Sudan did not become a country since July 2011

Siwa oasis and Wadi El-Natrun depression (Google Earth)
2 Key Messages of Using Groundwater in Agriculture
Food production requires the largest quantities of water, with groundwater resources providing more than 40% of all water used globally for irrigated agriculture [1, 11].
Groundwater has proved to be a critical input for securing improved crop yields and enabling the 250% increase in food production achieved during the “green revolution” of 1970–2000 [1, 11].
During the past 30–40 years there has been a remarkable level of investment in private water well construction for agricultural irrigation, because groundwater availability allows higher crop yields and high farmer returns per unit of water.
Groundwater storage is very large but current withdrawal rates for irrigated agriculture in more arid areas are not physically sustainable, resulting in long-term (semi-permanent) depletion of aquifer reserves at rates in excess of 120 km3/a [1].
Land-use practices affect ground in the form of water recharge rates and quality; with intensification of cropping diffuse pollution of groundwater by plant nutrients, salinity and some residual pesticides is common.
There is a pressing need to mobilize groundwater professionals, together with water-resource managers and irrigation engineers, to identify trans-sectoral governance and management responses for improving resource sustainability.
3 Priority Actions
Elaboration of sustainable groundwater management plans for aquifers under pressure from irrigated agriculture, including the identification of improved irrigation-water management measures [1].
Integrated evaluation and conjunctive management of groundwater and surface-water in major alluvial areas to enhance agricultural productivity and to avoid land drainage problems.
Careful evaluation and monitoring to ensure that crop irrigation practices and new irrigation developments reduce groundwater salinization problems.
Promotion of land management measures by farmers to enhance groundwater recharge rates and to reduce nutrient, salinity, and pesticide leaching to groundwater.
Re-aligning government finances (such as crop guarantee-prices, pumping-energy subsidies, water well, and irrigation hardware grants) so as to reflect limited groundwater availability and the value of lost ecological services – thereby supporting initiatives for sustainable resource management.
4 Water Supply in Egypt
4.1 Nile River
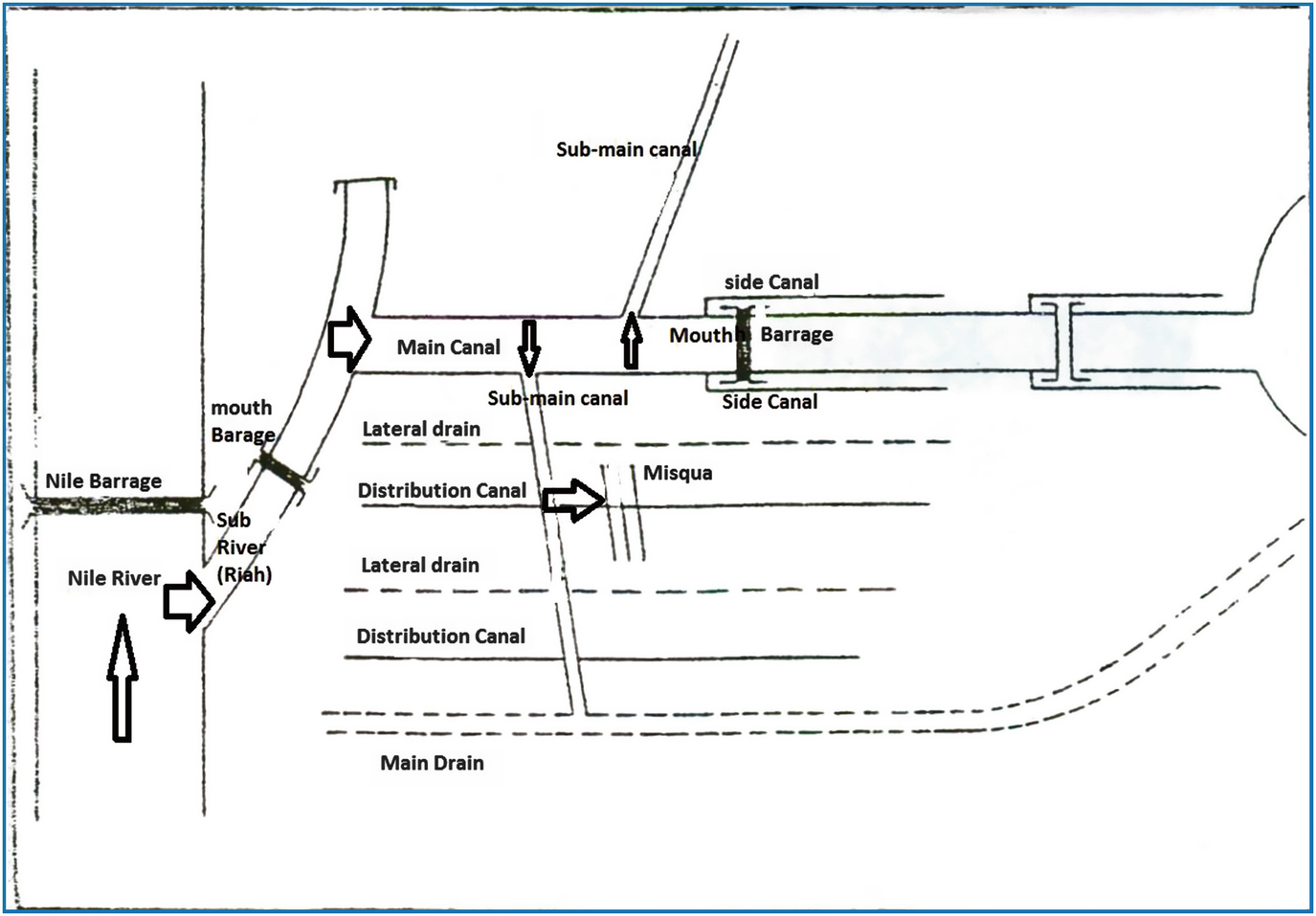
Nile water distribution system (irrigation canal system; author artwork)
4.2 Precipitation

The map of Egypt (Google map)
Renewable water resources in Egypt (downstream country) compared with Ethiopia (upper stream country) [12]
Aspect of water use | Egypt | Ethiopia |
---|---|---|
Average precipitation in volume (billion m3/year) | 51.07 | 936.4 |
Total actual renewable water resources (km3/year) | 57.5/85.8 | 122.00 |
Total renewable water resources per capita (m3/inhab/year) | 702.80 | 1,512.00 |
% of withdrawals of total actual renewable water resources | 117.20 | 5.10 |
% of total actual renewable water resources by agriculture in 2002 | 103.00 | 4.3 |
Dependency ratio (%) | 96.86 | 0.00 |
Irrigation potential (1,000 ha) | 4,420.00 | 2,700.00 |
Area equipped for irrigation (1,000) | 3,422.00 | 290.00 |
Rain-fed agriculture requires a rain (enough to saturate the root zone area and soil profile each time) in addition to continuous and steady rains along the growth season along the plant life cycle (from seed to seed) which usually extends for 150–180 days. But, excess Heavy rain and flash floods may cause runoff and soil erosion [13]. Most rain in Egypt is scattered and non-continuous through the plant life cycle and usually stops within 90 days only; thus, the amount of rain in the Mediterranean coastal lands (old and desert soils) is not considered a viable area for rain-fed agriculture due to its high spatial and temporal variability.
4.3 Groundwater
Groundwater is stored in aquifers, which are water-bearing rock formations that hold water in the inter-particle pore space and cracks within rock material. There are two basic types of aquifers including the unconfined aquifer and the confined aquifer. An unconfined aquifer (also called a water table aquifer) has an extensive water table open to recharge by precipitation. A confined aquifer does not have an extensive water table. Water is pressurized and some of it can flow from the well without pumping [14]. This water, generally described as fossil water, is mostly nonrenewable except for coastal and Delta aquifers. The chemical quality of the water is generally suitable for irrigation and domestic uses with an average total dissolved solids (TDS) less than 1,000 ppm. Salinity increases approaching the east/west extremities, and ranges from 500 to 3,000 ppm. High salinity water could be made suitable for crop irrigation by combining two parts of surface water to one part of groundwater [15]. This kind of groundwater is suffering from the residues of both pesticides and fertilizers in addition to salts that leached from the soil above.
Groundwater exists in the Western Desert and Sinai in aquifers that are mostly deep and non-renewable. The total groundwater volume has been estimated at about 40,000 BCM. However, current abstraction is estimated to be 2.0 BCM/year. The main obstacles in utilizing this huge resource are the great depths (up to 1,500 m in some areas) of these aquifers and deteriorating water quality at the increasing depths [9].
Shallow Groundwater in the Nile aquifer cannot be considered a separate source of water. The aquifer is recharged only by seepage losses from the Nile; the irrigation canals and drains and percolation losses from irrigated lands. Hence, its yield must not be added to Egypt’s total water resources. Therefore, it is considered as a reservoir in the Nile River system with a huge capacity, but only 7.5 BCM/year is rechargeable live storage.
The total available storage of the Nile aquifer was estimated at about 500 BCM but the maximum renewable amount (the aquifer safe yield) was estimated to be only 7.5 BCM. The existing rate of groundwater abstraction in the Valley and Delta regions is about 4.5–6.5 BCM/year (2013), which is still below the potential safe yield of the aquifer [9].
4.4 Seawater Desalination
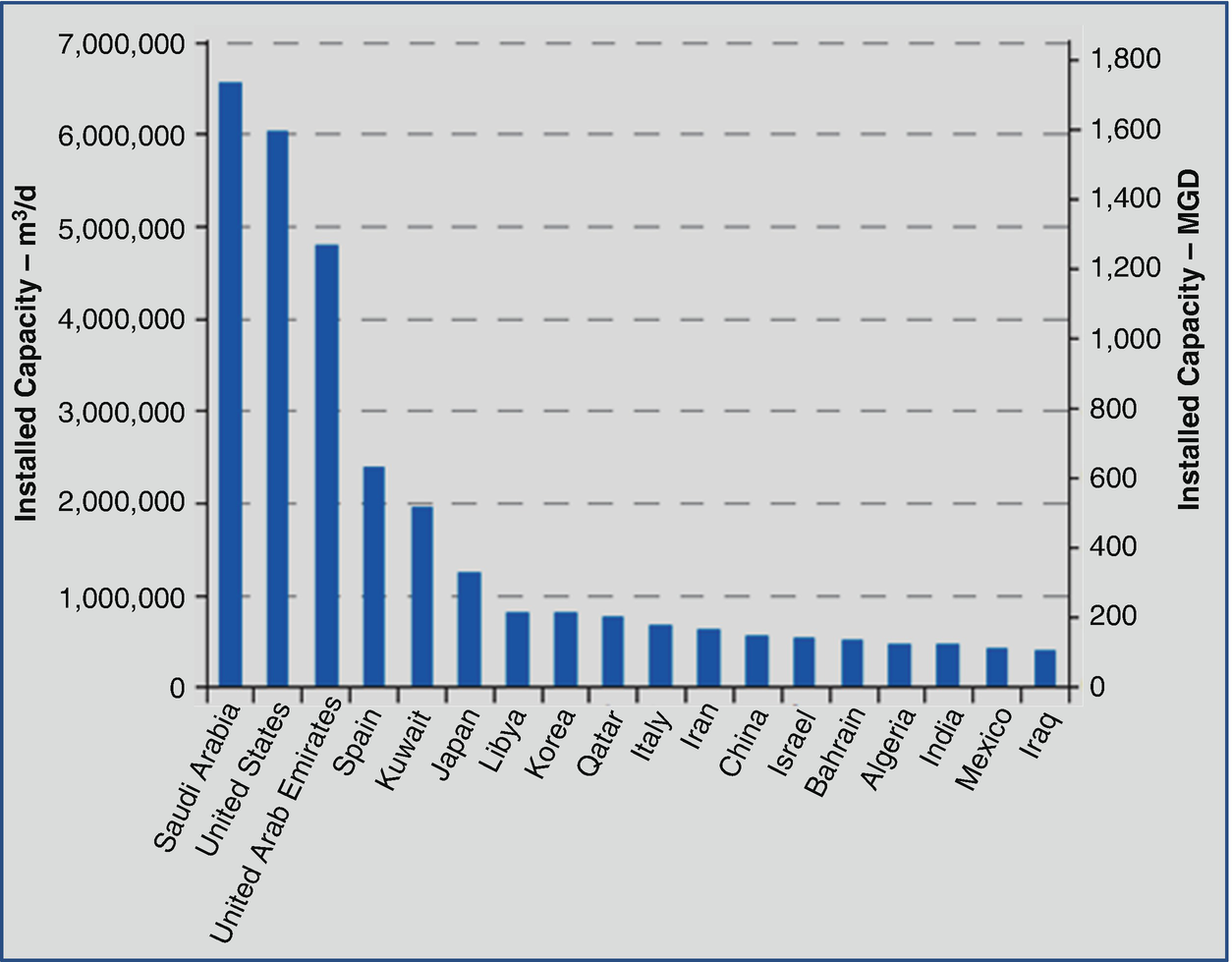
The top list of countries producing desaline water [16]

The cost items of de-saline water production and its sectorial uses [16]
- 1.
Seawater desalination is expensive.
- 2.
Seawater desalination will provide little benefit and has a poor track record.
- 3.
Seawater desalination invites corporate control and abuse of our water supply.
- 4.
Seawater desalination endangers the environment and public health.
- 5.
Seawater desalination could contribute to global warming.
- 6.
Seawater desalination threatens fisheries and marine environments.
- 7.
Seawater desalination poses a risk to human health.
- 8.
Seawater desalination promotes social and environmental injustice.
At the end of both reports the following statement was summarized: “Desalination of the sea is not the answer to our water problems. It is survival technology, a life support system, an admission of the extent of our failure.” Finally, according to the last conservative, the total volume of de-saline water in the world is low as 24 BCM/year, which represents 0.6% of the total global freshwater, most of them used mainly to conserve the human life, i.e. 57% for domestic and municipal uses, 23% for industry, 18% for generating power, and only 1% used in agriculture according to its high expense and low return from agriculture. Figure 8 shows the cost items and sectorial uses of the de-saline water.
4.5 Treated Wastewater
Treated domestic sewage is being reused for irrigation with or without blending with freshwater. The increasing demands for domestic water will increase the total amount of sewage available for reuse. It is estimated officially that the total quantity of reused treated wastewater in Egypt is about 0.3 BCM in 2013, with another 5 BCM primary treated. Industrial treated wastewater reached almost a BCM/year by recycling it within the facility. There is another untreated one BCM delivered in irrigation and drainage canal with a high load of heavy metals and pathogens [9].
Wastewater treatment could become an important source of water and should be considered in any new water resource development policy. However, proper attention must be paid to the associated issues with such reuse. The major issues include public health and environmental hazards as well as technical, institutional, socio-cultural, and sustainability aspects.
4.6 Reuse of Agricultural Drainage Water
The amount of water that returns to drains from irrigated lands is relatively high (about 25–30%). This drainage flow comes from three sources: tail end and seepage losses from canals, surface runoff from irrigated fields, and deep percolation from irrigated fields (partially required for leaching salt). None of these sources is independent of the Nile River. The first two sources of drainage water are considered to be freshwater with relatively good quality. The agricultural drainage of the southern part of Egypt returns directly to the Nile River where it is mixed automatically with Nile freshwater which can be used for different purposes downstream [9]. The total amount of such direct reuse was estimated to be about 4.07 BCM/year in 1995/1996. In addition, it is estimated that 0.65 BCM/year of drainage water is pumped to the El-Ibrahimia and Bahr Youssef canals for further reuse. Another 0.235 BCM/year of drainage water is reused in Fayoum while about 0.65 BCM/year of Fayoum is drained to Lake Qarun. Moreover, drainage pumping stations transport about 0.60 BCM/year of Giza drainage water to the Rossita Branch just downstream of the Delta Barrages for further downstream reuse.
According to the Ministry of Irrigation and Public Works 2016 [10], the total amounts of agricultural drains in the Delta cultivated lands (4.5 Million Feddans (MF)) reached 15 MCM. Officially the amount of reused agricultural drainage water is 8 BCM but in reality it exceeds 10 BCM. On the other hand, Upper Egypt which has nearly 1.5–2 MF (4,200 m2) of cultivated land is fed through both Nile River irrigation and drainage canals concurrently. The amount of agricultural drainage water that returns to the Nile course is expected to be between 4–5 BCM/year. Thus, the actual reuse of agricultural drainage water reached 10 BCM/year in the Delta with an additional 5 BCM/year in Upper Egypt for a total of 15 BCM/year [10].
5 Water Scarcity in Egypt
Water security is the availability of an acceptable quantity and quality of water for health, livelihoods, ecosystems, and production, coupled with an acceptable level of water-related risks to people, environment, and economies [14]. The share water in Egypt currently is 680 m per capita; this is below the scarcity level of 1,000 m per capita. Egyptian citizens should have renewable freshwater resources not less than 92 BCM for its 92 million population; however, only 62 BCM is available, leaving a water shortage of 28 BCM. Thus, Egypt must use the marginal and poor quality water and reuse and recycle when available. The reuse of agricultural drainage water, treated sanitary water, and treated industrial water, produces 17 BCM, helps to decrease the actual water shortage in Egypt from 31 BCM/year to 14 BCM/year. Improving the existing distribution system through the open non-cementing, non-piping irrigation canal, running along 30,000 km from the High Aswan Dam lake south Egypt to the last point of the irrigated soil in North Delta may add another 19 BCM/year usually lost by evaporation and seepage within this long wide mud irrigation canals net. The reuse of marginal poor quality water in Egypt has already been established and will continue to be developed in water policy in the future in the face of rising water scarcity.
6 Sectoral Water Demand
Water requirements in Egypt are continuously increasing due to population increases and the improving standards of living as well as the governmental policy to encourage industrialization. Demands for water can be categorized in classes representing the demand and water balance. Water requirements of the agricultural sector represent the largest component of the total water demand in Egypt.
6.1 Agriculture
Agriculture consumes more than 85% of Egypt’s water resources or about 103% of the shared water from the Nile river by almost of 57 BCM (see Table 1). Although the country has lost a part of its fertile land due to urbanization, this has been balanced by expansion of agricultural areas into new regions. Expansion in agriculture is carried out horizontally and vertically through intensive cultivation in the different crops to produce food, forage, leaf, and medicinal plants. In 1990 cultivated lands occupied 6.92 MF with a cropped area of about 12.43 MF (two seasons a year), while in 2010 cultivated areas and cropped lands were 8.6 MF and 17.50 MF, respectively [18].
6.2 Municipal and Domestic
Municipal water requirements include water supply for major urban and rural villages. Part of this water comes from the Nile system and the other part comes from groundwater resources. Municipal water demand was estimated to be 10 BCM in 2010, where approximately 97% of the urban population and 70% of the rural population of Egypt access piped clean drinking water supply. Sanitation facilities are less developed where approximately 90% of the urban population and 40% of the rural population was planned to be connected to a sewerage system in 2016. Municipal water is supplied by about 83% from Nile water and 17% from groundwater.
6.3 Industry
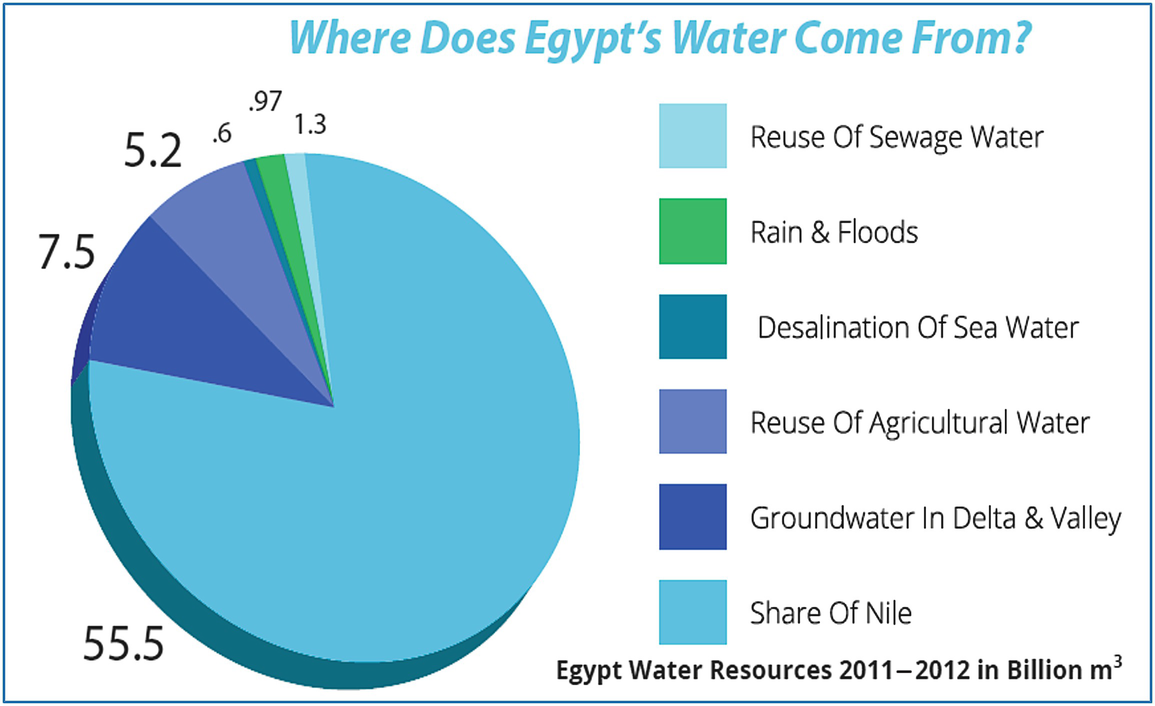
The distribution of freshwater in the different sectors in Egypt (2010) [19]
7 Allocation of Groundwater in Egypt
The regional Nubian Sandstone Aquifer System, occupying much of Egypt and continuing across the border in a westward direction into Libya, in the south and southwestward direction into the Sudan and Chad, and in the eastward direction into Israel, Jordan, and the Arabian Peninsula [21]. The thickness of the sediments varies from a few hundred meters in the south, to 4,000 m west of Abu Mongar.
Carbonate Aquifers occupy at least 50% of Egypt. They are made of fissured limestone, giving rise to natural springs with a total flow of 200,000 m3. Salinity varies between 1,500 and 7,000 ppm. Lower salinity was reported in Siwa Oases at 200 ppm [22].
The Moghra Aquifer system has a broad geographical distribution in the region west of the Nile Delta and south of the Qattara Depression [21], Fig. 4. It is characterized by its moderate to high salinity, which ranges between 1,000 and 5,000 ppm with a present dominant average of 4,000. The aquifer water is a mixture of Paleo water and renewable water.
The Nile valley and Delta aquifer is the most productive, containing around 200 × 103 million m3, renewable by seepage from Nile river irrigation systems. Thickness of this aquifer decreases from 300 m at Sohag Governorate in the Upper Egypt to few meters near Great Cairo (Cairo, Giza, and Qalyubia governorates) and also in the south near Aswan. This aquifer loses its water through the Rosetta Branch into the Mediterranean and Suez Canal [22]. The Nile receives a total amount of 1.6 × 103 million m3/year as drainage from aquifers. The total amount of groundwater already used for agriculture and domestic purposes is about 2.6 × 109 m3/year and is extracted from about 9,000 wells [18]. Approximately 1.0 × 103 million m3/year of groundwater is extracted through productive wells. In 1990, total extraction of groundwater amounted to 3.3 bm3 to meet irrigation and domestic water requirements. It is estimated that 4.8 bm3 of groundwater is currently used in the Valley and Delta, as well as 0.57 bm3 in the desert and Sinai for a total of 5.37 bm3 of groundwater. Groundwater extraction can be increased to 11 bm3 without depletion of underground reservoirs. There is a possible future increase of 8.3 bm3 [18].
The Coastal Aquifer lies 35 km from the seashore, 45 km north of Cairo and is recharged mainly from rainwater and from high-pressure water in the Nubian Sandstone aquifer. It is renewable, but deeper and with salinity varying between 3,000 and 5,000 ppm [22].
Rose basement rock has the same characteristics as the Carbonate Aquifer but is difficult to explore since it is very deep, (1,200–2,000 m depth) and has a high cost of digging (least L.E 5 million) [22].
Groundwater occurs in the western desert in the Nubian sandstone aquifer that extends below the vast area of the New Valley governorate and the region east of Owaynat Mountain. It has been estimated that about 200,000 BCM of freshwater is stored in this aquifer. However, groundwater is available at greater depths and the aquifer is generally non-renewable.
Utilization of such water depends on pumping costs and its depletion rates versus the potential economic return in the long run.
Groundwater in the Sinai is mainly encountered in three different water-bearing formations: the shallow aquifers in northern Sinai, the valley aquifers, and the deep aquifers. The shallow aquifers in the northern part of Sinai are composed of sand dunes that hold the seasonal rainfall. The aquifers in the coastal area are subject to salt-water intrusion. The total dissolved solids in this water range from 2,000 to 9,000 ppm which can be treated to reach a suitable salinity level for use to irrigate certain crops.
The groundwater aquifers in the valleys of the Sinai are recharged from rainfall and particularly heavy storms. The annual rainfall in the Sinai varies from 40 to 200 mm/year. Most of the rainfall water recharges the shallow groundwater aquifers in northern Sinai such as the Delta of Wadi El-Arish and the El-Beqaa flood-plain, while such aquifers are absent in southern Sinai. Although most of the shallow aquifers are renewable, only 10–20% of the deep aquifers are renewable by rainfall and flash floods.
The total groundwater abstraction in the western desert in 1995/1996 was estimated to be about 0.48 BCM while it is only 0.09 BCM/year in Sinai.
8 Groundwater Potential
Groundwater development started in the early 1960s and hasbeen continuing. It is observed that the present use is concentrated in the Nile aquifer system, followed by the desert fringes and the Nubian sandstone.
Major aquifers, renewability, salinity, and productivity in Egypt [18]
Aquifer | Confined/non-confined/semi-confined | Renewable or non-renewable | Salinity | Productivity |
---|---|---|---|---|
Nile valley and Delta | Confined | Renewable | Low | Very high |
Moghra | Semi-confined | Non-renewable | High | Low |
Nubian sand stone | Unconfined | Non-renewable | Low | Medium |
Coastal | Unconfined | Renewable | High | Medium |
Carbonate | Non-confined | Non-renewable | High | Low |
Rose | Non-confined | Non-renewable | High | Low |
Available water resources in Egypt, 2000 and 2017 (% of total) [18]
Water supply source | Supply in year 2000 (BCM) | Estimated supply 2017 (BCM) |
---|---|---|
River Nile | 55.5 | 55.5 |
Rainfall | 1.0 | 1.5 |
Desert and Sinai groundwater | 0.6 | 3.5 |
Valley and Delta groundwater | 4.8 | 7.5 |
Municipal reused drainage water | 0.7 | 2.5 |
Agricultural reused drainage water | 5.1 | 8.4 |
Improved irrigation system | 0.0 | 7.0 |
Total available water 2000/2001 | 67.6 | 85.9 |
8.1 Nubian Sandstone Aquifer
Because the Nubian sandstone aquifer is the largest store of groundwater in Egypt, we will discuss it in detail. The Nubian Sandstone Aquifer System underlies virtually all of Egypt, much of eastern Libya, and significant areas of both northern Chad and northern Sudan [18]. It can be broadly described as two distinct aquifer systems vertically separated by layers of lower permeability that allow some upward leakage [23]. The deeper Nubian Sandstone Aquifer System is older and extends to the entire area while the Post Nubian Aquifer System lies above that in more recent geological formations and covers roughly the northern half of the larger system [18].

Transboundary aquifers [29]

Nubian sandstone aquifer [29]
8.2 Precipitation in the Nubian Sandstone Aquifer
Arid and hyper-arid climate conditions in most of the region magnify the aquifer’s importance. Almost all of the water used by people living above the aquifer comes from either groundwater abstraction or diversion from the Nile. The average annual mean rainfall is less than 50 mm and on average vast areas over the aquifer system receive no measurable precipitation. The wettest area at the northern most tip of Libya receives around 425 mm of rain. Northern Darfur in Sudan is the wettest area to the south but sees only an average of around 200 mm each year [30].
8.3 Desert Oasis Development: Dakhla Oasis
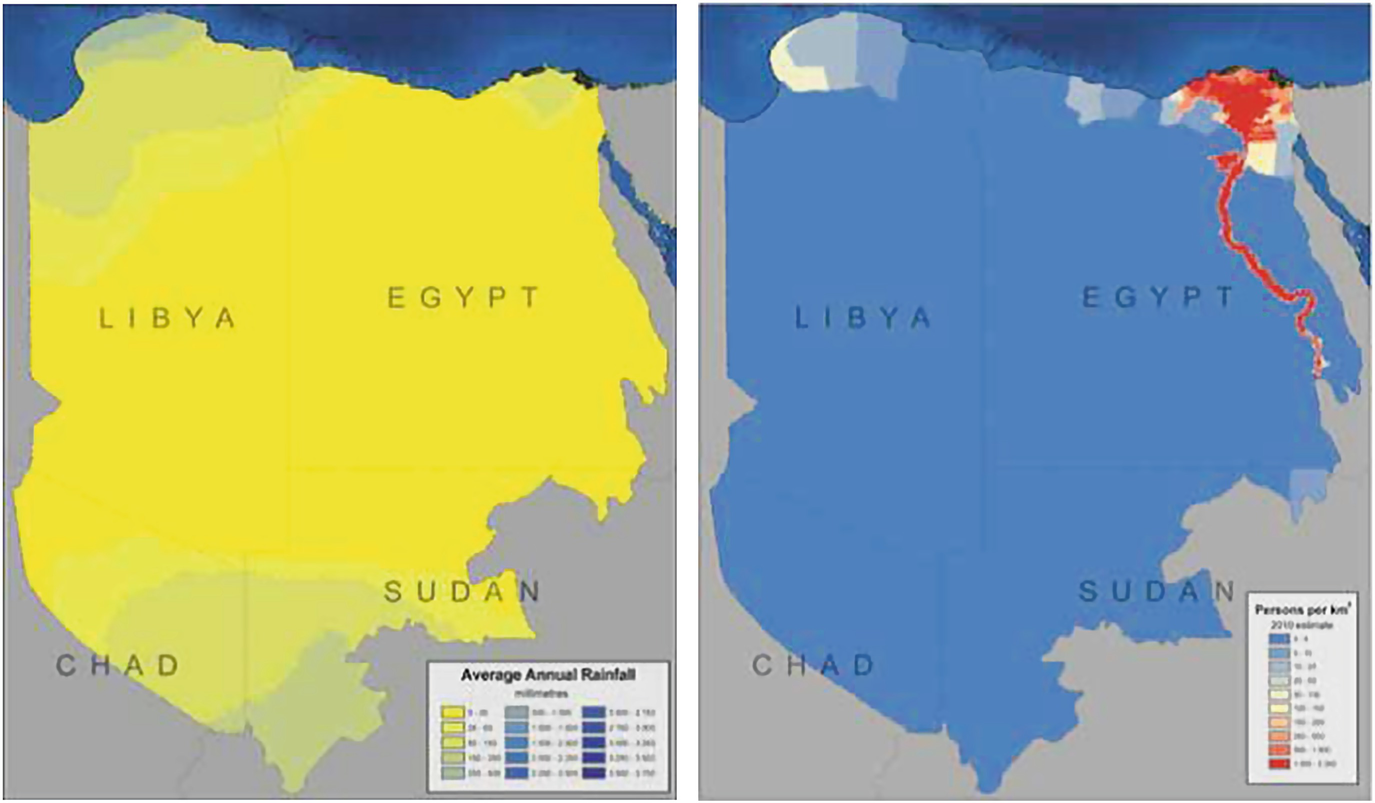
Nubian sandstone system [29]; average annual rainfall (left), and population density (right)
9 Benefits of Groundwater Use for Agricultural Irrigation
Is usually found close to the point-of-use (often only a well’s depth away)
Can be developed quickly at low capital cost by individual private investment
Is available directly on-demand for crop needs (given a reliable energy source for pumping) and thus affords small-holders a high level of control year-round
Is well-suited to pressurized irrigation and high productivity precision agriculture
Has “democratized” irrigation by permitting irrigated agriculture outside canal command areas.
- 1.
Counterproductive competition between irrigation users.
- 2.
Conflicts with rural and/or urban drinking-water provision, making it more difficult to achieve MDGs.
- 3.
Impacts on natural aquifer discharge (spring flow, riverbed flows), which result cumulatively in an unacceptable impact on “downstream” surface water-flows.
Making a clear distinction between “groundwater-only irrigation areas” and “conjunctive-use irrigation areas,” since these present very different prospects, approaches, and challenges for resource optimization.
Focusing resource-management efforts on constraining consumptive use (rather than just groundwater withdrawals), especially in groundwater-only irrigation areas
Assessing groundwater-surface water connectivity in alluvial environments, as a basis for taking advantage of the opportunities of “conjunctive management” whilst avoiding the risk of “double-resource accounting.”
9.1 Hazards of Excessive Groundwater Exploitation
The salinization of aquifers – which is a very insidious and often complex process arising from a variety of physical mechanisms.
Troublesome land subsidence due to the settlement of interbedded aquitards in alluvial and/or lacustrine formations increasing (and in some cases spiraling) electrical energy costs for pumping, especially where use is “buffered” by subsidies or flat-rate tariffs – with serious implications for many electricity utilities and for the unit energy consumption and carbon footprint of irrigated agricultural production [31, 32].
9.2 Climate Change
Climate change will affect the natural water balance and water availability in several ways. First, changes in spatiotemporal patterns and variability of precipitation affect the replenishment of water resources. Second, increase in water and soil salinity due to high temperature of global warming. Third, the contamination of irrigation water will decrease water efficiency, water return benefits, and causing land degradation which leads to increase in the water requirements needed to produce food. This is not easy under the water deficiency in Egypt. Thus, much pressure will hit the ground and surface water to meet the increasing demands of different sectors of agriculture, industry, domestic, and municipal.
Groundwater reportedly provides drinking water to at least 50% of the global population and accounts for 43% of all water used for irrigation [33]. Groundwater also sustains the base flows of rivers and important aquatic ecosystems. Uncertainty over the availability of groundwater resources and their replenishment rates pose a serious challenge to their management and in particular to their ability to serve as a buffer to offset periods of surface water scarcity [34]. Groundwater supplies are diminishing, with an estimated 20% of the world’s aquifers being over-exploited [35], leading to serious consequences such as land subsidence and saltwater intrusion in coastal areas [6]. Groundwater levels are declining in several of the world’s intensely used agricultural areas and around numerous mega-cities [33]. In the Arabian Peninsula, freshwater withdrawal, as a percentage of internal renewable water resources, was estimated at 505% in 2011 [6], with significant volumes of groundwater reserves being transboundary in nature [12].
9.3 The Diagnosis of Groundwater Salinization
Rising water-table due to excessive canal seepage and/or field application in head-water areas leading to soil water-logging and phreatic salinization, or sometimes naturally saline shallow groundwater becoming mobilized.
Leaching of soil salinity across irrigation areas on first habilitation of arid soils and/or salt fractionation by “efficient” irrigation, with accumulation in tail-end sections of canal commands if no groundwater outflow occurs.
More frequent coastal lateral intrusion or inland up-coning of saline groundwater due to excessive abstraction of fresh groundwater.
Additionally, there are hyper-arid areas in which virtually all groundwater is naturally saline, except where there exists some infiltration from surface watercourses and irrigation canals forming “freshwater lenses.” The implication is that groundwater salinization threats need sound diagnosis, close monitoring, and careful management.
9.4 Groundwater Quality Impacts of Irrigated Agriculture
Leaching of soil nutrients is a problem that has been exceptionally widespread in the industrialized nations with (largely successful) attempts to increase grain, oil-seed, vegetable, fruit and milk production per unit area through the replacement of traditional crop rotations with near monocultures, but as yet has been less severe in the developing world where inorganic fertilizer applications have generally been much lower – in theory at least the problem of soil nutrient leaching should also be more manageable in irrigated than rain-fed agriculture.
Contamination with pesticides is a potentially serious problem but one more confined geographically to recharge areas of aquifers exhibiting high vulnerability to pollution from the land-surface, where the more “mobile” pesticides (mainly certain herbicides and soil insecticides) have been regularly used at high application rates.
Mobilization of salinity is an issue of very serious concern in arid and hyper-arid areas where the “irrigation frontier” has (or is) being extended through clearing of native desert scrubland with high salinity levels retained in the sub-soil profile.
10 Use of Groundwater in Agriculture in Egypt
- Principle 1:
Improving efficiency in the use of resources is crucial to sustainable agriculture.
- Principle 2:
Sustainability requires direct action to conserve, protect, and enhance natural resources.
- Principle 3:
Agriculture that fails to protect and improve rural livelihoods, equity, and social well-being is unsustainable.
- Principle 4:
Enhanced resilience of people, communities, and ecosystems is a key to sustainable agriculture.
- Principle 5:
Sustainable food and agriculture requires responsible and effective governance mechanisms.
- 1
Environmental services include: Climate, Nutrient cycling, Biodiversity, Water cycles, Coastal protection…
- 2
Natural resources include: Land, Oceans, Water, Genetic resources, Forest resources, Aquatic systems, Nutrients, and Energy.
- 3
Agriculture includes: Crops, Livestock, Forestry, Fisheries, and Aquaculture
- 4
Agricultural products include: Food, Feed, Fiber, Fuel, and Medicinal crops
- 5
Economic and social services include: Growth, Poverty reduction, Employment, Stability, Health, and nutrition.
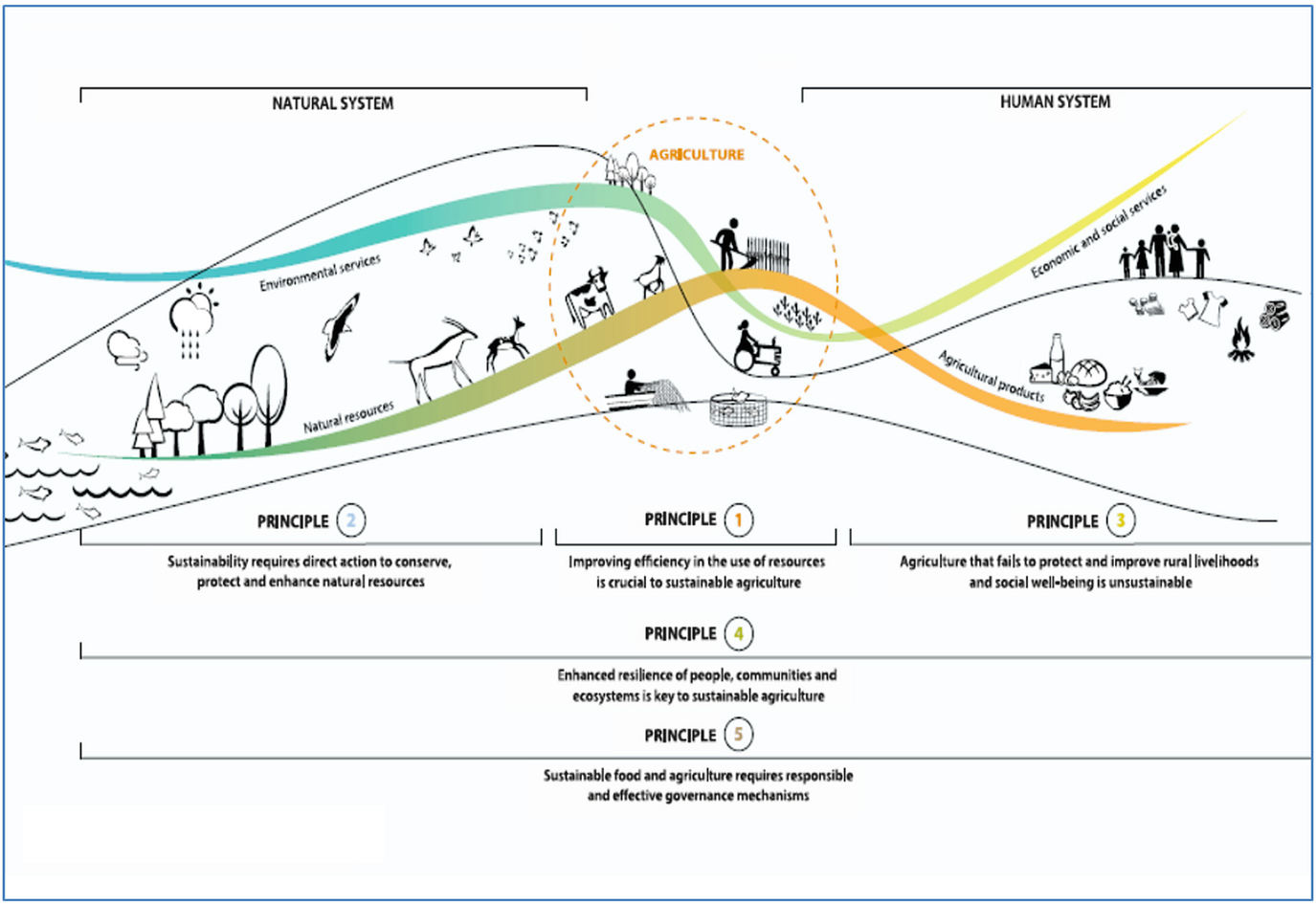
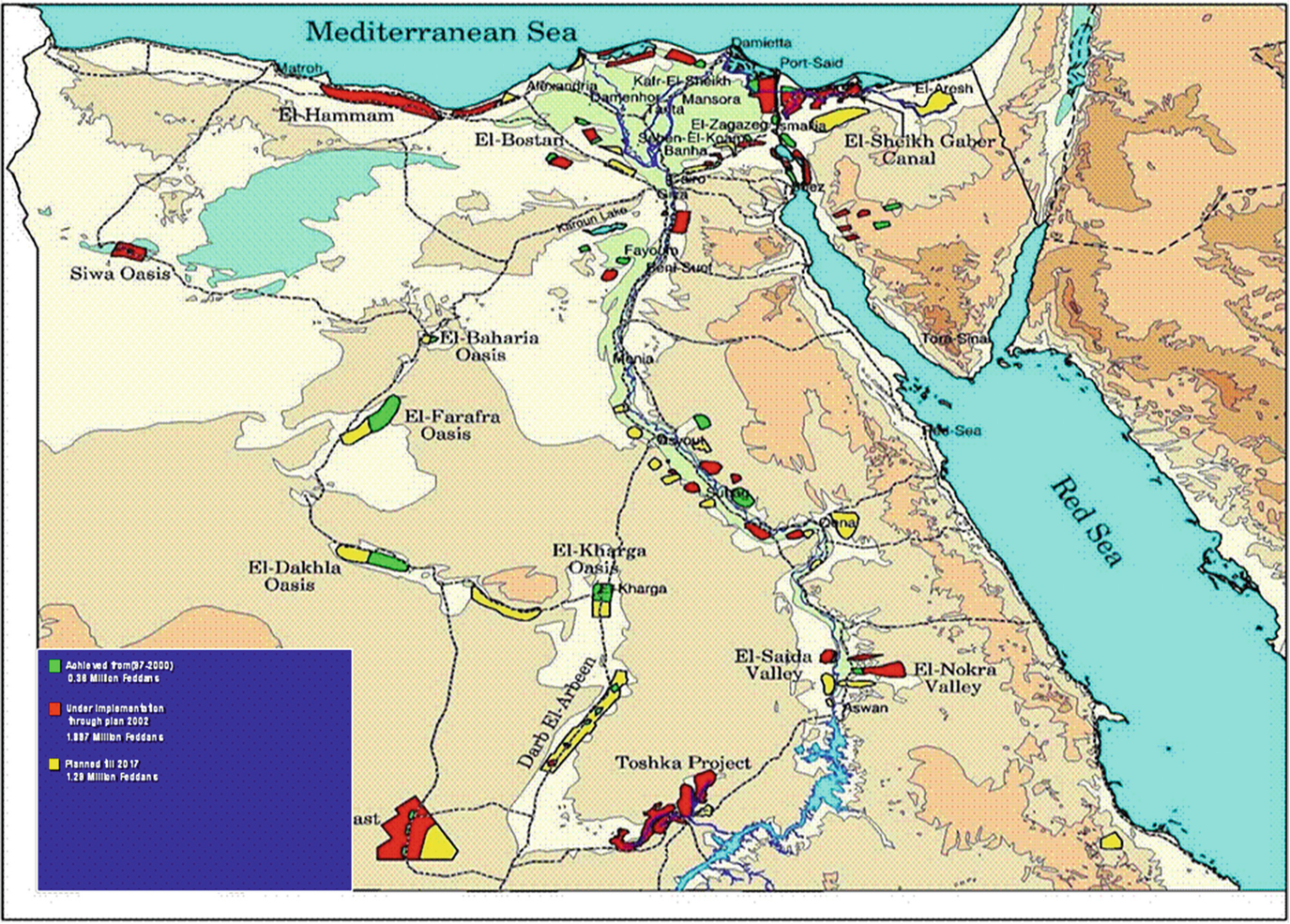
The master plane of Egypt for agriculture extension up to year 2017 (nothing achieved) [39]

The location of the reclamation project (2016) for reclaiming 1.5 MF [10]
10.1 Use of Groundwater in Irrigation in the Nile Valley and Delta and Their Fringes
The total dissolved salts in Nile water is 500 g/m3 measured at Giza Nile [37], and this concentration will add 3.5 tons of hazardous soluble salts every year to each feddan (4,200 m3) at the rate of total annual irrigation water and consumptive use of 7,000 m3. This number will increase threefold when using agricultural drainage water of 10 BCM/year (this amount represents about one third of total irrigation water in the delta soils with total area of 4.5 MF). The same trend of salt accumulation will exist when using shallow, poor quality water table in irrigation. Actually the Egyptian farmers obliged to frequently and continuous use of this poor quality water because the Ministry of Water Resources and irrigation only delivers 5,000 m3/acre/year, instead of 7,000 m3/acre/year, the actual water requirements per acre due to high temperature weather in Egypt. Thus, the farmers are obliged to manage and find a way to get an additional 2,000 m3 from any near water resources to complete their field requirement of irrigation water. Most of the farmers in the delta areas or in the Giza Governorates make groundwater wells at a depth usually ranging between three meters and not to exceed 20 m to help them in completing their water needs.
10.2 Wadi El-Natrun

Wadi El Natrun depression

Haphazard wells in Wadi-El-Faregh includes Wadi El-Natrun that led to depletion of groundwater
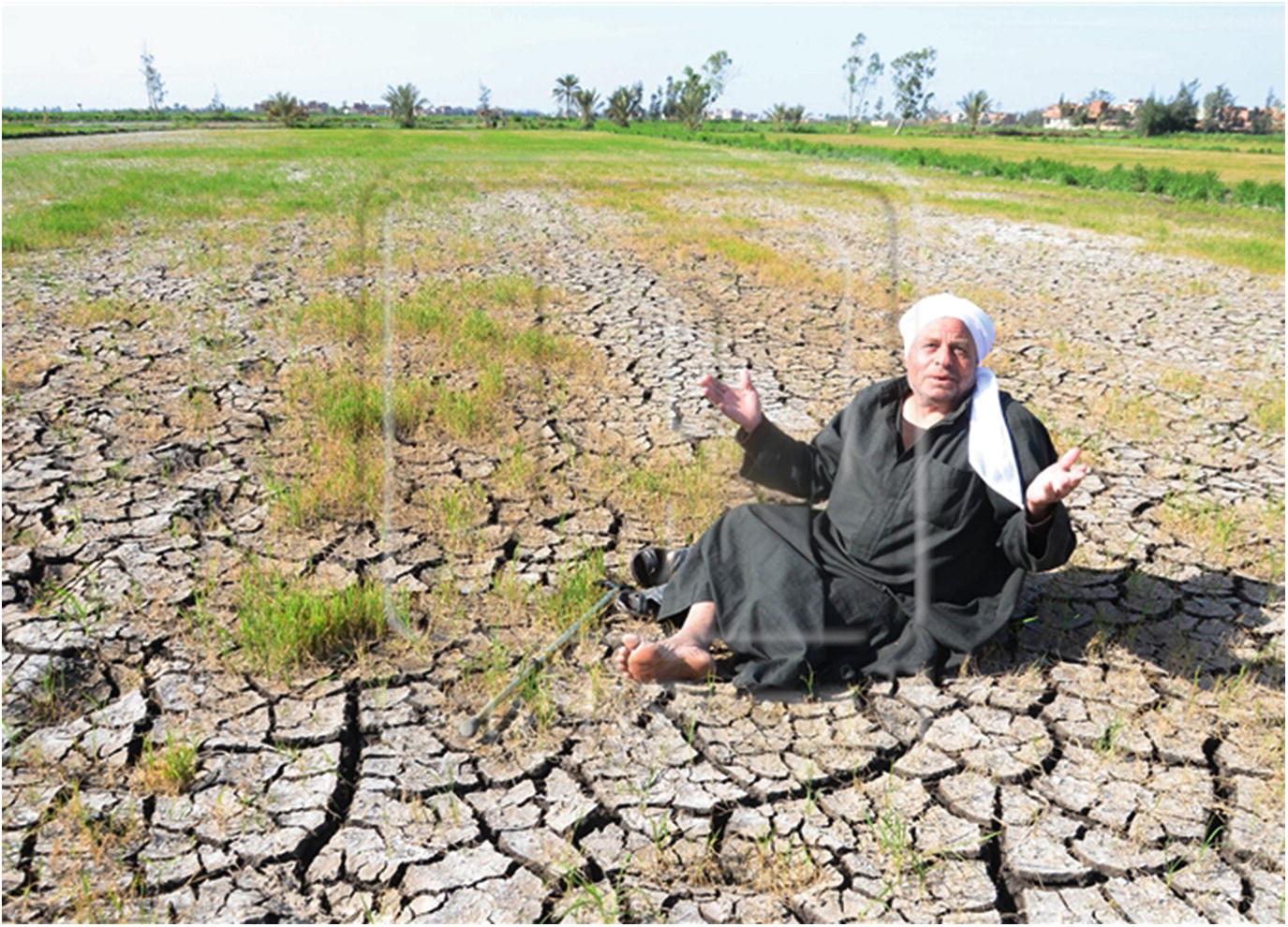
Salt and white crust in some delta soils resulting from using shallow groundwater in irrigation (Author 2015)
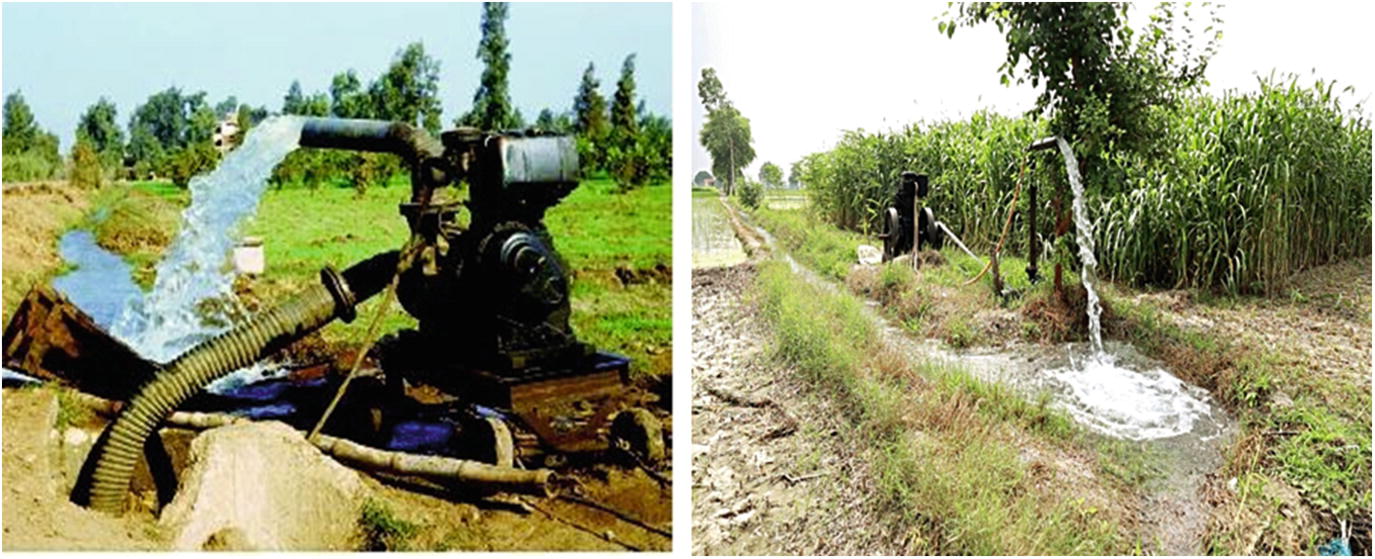
Shallow groundwater in Wadi El-Natrun (right) and in the delta lands (left) [Author 2015]
10.3 Siwa Oasis
The Siwa Oasis is a natural depression about 18 m below sea level. It is located in the northern part of the Western Desert of Egypt (about 90 km east of the Libyan border) and 300 km south of the Mediterranean Sea. Groundwater is the only source of drinking and irrigation water in region. Old artesian wells originated from the top shallow aquifer (220 wells) are the traditional source of irrigation water in Siwa Oasis. Under the need for more water source, the upper limestone aquifer has been tapped by hand-drilled boreholes to add new source of irrigation water. For historical, demographic, and economic reasons as well as its high potential for agricultural development, the Siwa Oasis is considered the most important oasis in the western desert of Egypt. In addition to the existing cultivated area, there are more than 17,000 feddans that were determined to be suitable for agricultural development. The area under cultivation has been gradually increased in recent years as the population of the oasis is on the rise. New land reclamation development projects aimed at the exploitation of water resources have been in progress. Therefore, there is an urgent need for monitoring water quality in the Siwa Oasis [38, 42]. It is noted that the water quality in the Siwa Oasis has been deteriorating over time, and there is an urgent need for long-term monitoring of water quality of the available water resources in the Siwa Oasis.
A scientific study concluded that the Siwa Oasis is suffering from high salinity, soil as a result of the exchange, which will negatively impact agricultural areas where irrigation water is misused, leading to lower productivity of crops [43, 44].
The Siwa Oasis (1,000 km2) is today suffering from an excessive rise in the subsoil water levels. The rate of groundwater has risen from 1.33 to 4.6 cm/year. Consequently, the fertile soils are subjected to deterioration and salinization. These events will require new chain water management scenarios that make use of suitable water (multiple reuses) before it reaches the last disposal point to increase the cultivated area (17,000 feddans). The salinity of groundwater representing the area changed from fresh to saline groundwater, 168.8–7,472.8 mg/L and total hardness from soft to hard, 30.7–1,829.7 mg/L as CaCO3 [44].
The groundwater in the Siwa Oasis lies in two different aquifers: Nubian sandstone and fractured dolomite limestone. The fresh groundwater and brackish and saline contained Nubian sandstone and fractured dolomite limestone. Chemical analysis of the groundwater shows the total dissolved solutes changed from fresh to saline 168.8–7,472 mg/L, total hardness ranges from soft to hard, 30.7–1,829.7 mg/L as CaCO3. The effective ions that cause an increase of water salinity are in descending order: As cations; Na+ > Mg2+ > Ca2+ while the anions Cl− > SO4 2− > HCO3− in both brackish and saline groundwater samples and HCO3− > Cl− > SO4 2− in fresh. Also, the ion ratios indicated that the groundwater has a mixed mineralization that is possibly pure meteoric water affected by leaching and dissolution and cation exchange of both terrestrial and marine salts [45].
Because Siwa Oasis is a depression inside a vast depression of western desert, their main problems are poor drainage with no drainage outlet, and water logging. The second problem is the shallow under pressure groundwater that pops up to the ground. Many scientists call Siwa oasis “the dead oasis,” but others see Siwa for its potential for agricultural development.
11 Conclusion and Recommendation
Egypt relies most on Nile water (55.5 BCM/year), then the reuse of agricultural drainage water (10 BCM/year), and finally the groundwater (5.5 BCM/year) as an additional water source for drinking (first) and irrigation (second). There are also the uses of reclaimed and primary treated wastewater (5.5 BCM/year) with little amounts of rain that precipitate on the delta lands which has not exceeded 1.3 BCM/year. Use of groundwater in agriculture activity is effective in the new reclaimed lands in the deserts where the Nile water is not available or access. Most of the groundwater in Egypt is non-renewable water except the shallow groundwater in the Nile valley and delta lands and its fringes in addition to some famous depression and oasis like Wadi El-Natrun in west delta (the Valley of Sodium salts) and Siwa oasis south the northwest coast of Mediterranean.
The share water per capita in Egypt nowadays is 666 m; below the scarcity level of 1,000 m per capita/year. That means the Egypt should have additional renewable freshwater resources to raise the total water resource to 93 BCM/year for the 93 million capita to reach the scarcity level (1,000 m3/capita.year). The actual situation refers to, the total available water resources is only 62 BCM with a deep gap of water shortage by 31 BCM. Thus, Egypt policy has no choice to use and reuses the marginal and poor quality water. The reuse of both agricultural drainage water and treated sanitary wastewater in addition to recycle of treated industrial wastewater offering about 17 BCM helps to minimize the water shortage to 11 BCM instead of 28 BCM. However, the improvement of water delivery system through the open mud irrigation canal extended for 30,000 km long may add another 19 BCM/year lost by evaporation and seepage from these outdated canals. Finally we can say, the reuse of poor quality water in Egypt is vital, essential and will be continuing until found another renewable water sources to meet the increasing water demands.