1 Introduction
Groundwater is one of the most important resources of water in Egypt, ranking second after the River Nile. Although in terms of quantity the contribution of groundwater to the total water supply in Egypt is very moderate. Groundwater is the only available source of water for people living in the desert area. In terms of quality, groundwater, unless contaminated, is generally of better quality than surface water. The quality of groundwater depends on two main factors, namely the origin of the water and the type of rocks bearing it. Groundwater is obtained in many areas from fissures, and joints of the igneous and metamorphic rocks. It may be found in layers of weathered basement rocks. It can also be extracted from alluvial deposits and aquifers in coastal areas.
Demand for water has increased during the last decade particularly due to the declining availability of freshwater. This has expanded the development of searching and extracting groundwater resources. An evaluation of the renewable groundwater resources is essential for any Integrated Water Resource Management (IWRM) plans that are sustainable. In this study, the evaluation of the renewable groundwater resources in Egypt from water quality and quantity perspective is discussed.
2 Groundwater System in Egypt
They differ in general characteristics, including extension, hydraulic conductivity, transmissivity, storage, and recharge.
- 1.
Granular water-bearing rocks and
- 2.
Fissured and karstified water-bearing rocks
The main hydrogeological units in Egypt [3]
Lithology rock type | Unit | Recharge | Distribution | Productivity | |
---|---|---|---|---|---|
Surface | Sub-surface | ||||
Granular material | Nile Valley and Delta | Continues | Continues | Extensive | High |
Coastal aquifer | Occasional | Limited | Local | High | |
Nubian Sandstone | None | Limited | Extensive | High |
The Nubian Sandstone Aquifer is selected for further study in this respect since it is considered the main groundwater resource in the New Valley in the Western Desert of Egypt.
3 Regional Nubian Sandstone Aquifer System

The total area is about two million square kilometers. The Nubian Sandstone Aquifer in Egypt is assigned to the Paleozoic–Mesozoic. It occupies a large area in the Western Desert and parts of the Eastern Desert and Sinai.
“In spite of its vast area, it is considered as a broadly closed system, as it has natural boundaries to the east and southeast formed by the mountain ranges of the Nubian Shield and is bounded to the south and west by the mountainous outcrops of Kordofan Block, Ennedi, and Tibesti. To the southwestern part of the basin, the aquifer is bounded by the groundwater divide located between Ennedi and Tibesti Mountains. The natural northern boundary of the Nubian Sandstone Aquifer System is set to the so-called Saline-Freshwater Interface, whose location is considered spatially stable, although slight movement is believable,” Sefelnasr [9, 10].
“Groundwater can be found at very shallow depths, where the water-bearing formation (Horizon) is exposed or at very large depths (up to 1,500 m),” where the aquifer is semi-confined. “The deepest water-bearing horizons are generally encountered in the North,” as in the Siwa Oasis, while the shallowest are encountered in the southern portion of Kharga and the East Oweinat area. The aquifer transmissivity is generally medium to low, varying from 1,000 to 4,000 m2/day, Sefelnasr [10].
CEDARE/IFAD [6] in a program for the development of a regional strategy for the utilization of the Nubian Sandstone Aquifer System has estimated the fresh groundwater volume within the system at 372,950 km3.
“Groundwater quality is generally good in the major part, except near the coastal regions and Sinai,” RIGW [11] and Sefelnasr [10].
The following section is discussing in more detail the most recent study for the groundwater potential in the New Valley area of Egypt [12]. The objective of this study is to evaluate and manage the use of the valuable groundwater resources of the Nubian Sandstone Aquifer System (NSAS) in the study area. It is located in the middle part of Egypt’s Western Desert. It lies between latitudes of 24°–28° N and longitudes of 27°–31.5° E. It covers an area of about 440 km long by 460 km wide (about an area of 202,400 km2).
4 Conceptual Model
The hydrogeological groundwater flow modeling is based on a valuable tool to better understanding groundwater flow in aquifers and to better managing groundwater resources. Numerical models are one of the few tools available that can consider a complex array of aquifer variables (hydraulic properties, recharge, pumping, rivers, structure, and heterogeneity) and allow these variables to interact with themselves. Exploring these interactions with a model can be used to make predictions important for managing groundwater resources, such as predicting how water levels might respond to increased pumping or drought.
The model study area of “Dakhla Basin includes Farafra, Dakhla and Kharga Oases.” The Dakhla basin includes the upper Post Nubian Aquifer, separated from the Nubian by an aquitard. However, the model study includes the main deeper aquifer since the upper post-Nubian aquifer is almost declining and that it is not feasible to include it in the study. For this reason, the upper aquifer will be considered inactive in this model study. The Nubian aquifer in this respect still contains a huge groundwater storage that can help in the development of the New Valley region, provided that good water management plan is required since this Nubian system is not well recharged and should be treated as groundwater mining aquifer.
The oldest and most extended aquifer comprises the Paleozoic and Mesozoic continental deposits (zone 2). It is older than the Upper Cinemania (zone 1). A large part of the lower aquifer is exposed south of the 26° N parallel and is in unconfined condition.
More recent groundwater reservoir includes the Tertiary Carbonate rocks in western Desert of Egypt. However, this aquifer is heavily depleted, and most of the wells are becoming dry which forced the natives in the area abandon those wells and replace them with deeper wells drilled in the deep Nubian aquifer.
The two zones are separated by an impermeable shale layer (aquitard or confining bed) above which the Upper Cretaceous deposit is located.
According to the aforementioned discussion, it is only feasible to develop the lower deep Nubian aquifer. In this situation, the upper aquifer in zone 1 will be discarded because most of the springs and wells taping the upper limestone aquifer (PNSA) are becoming dry. This is beside the very low conductive layer that separates the two zones. A conceptual numerical model domain and its extensions are given below.

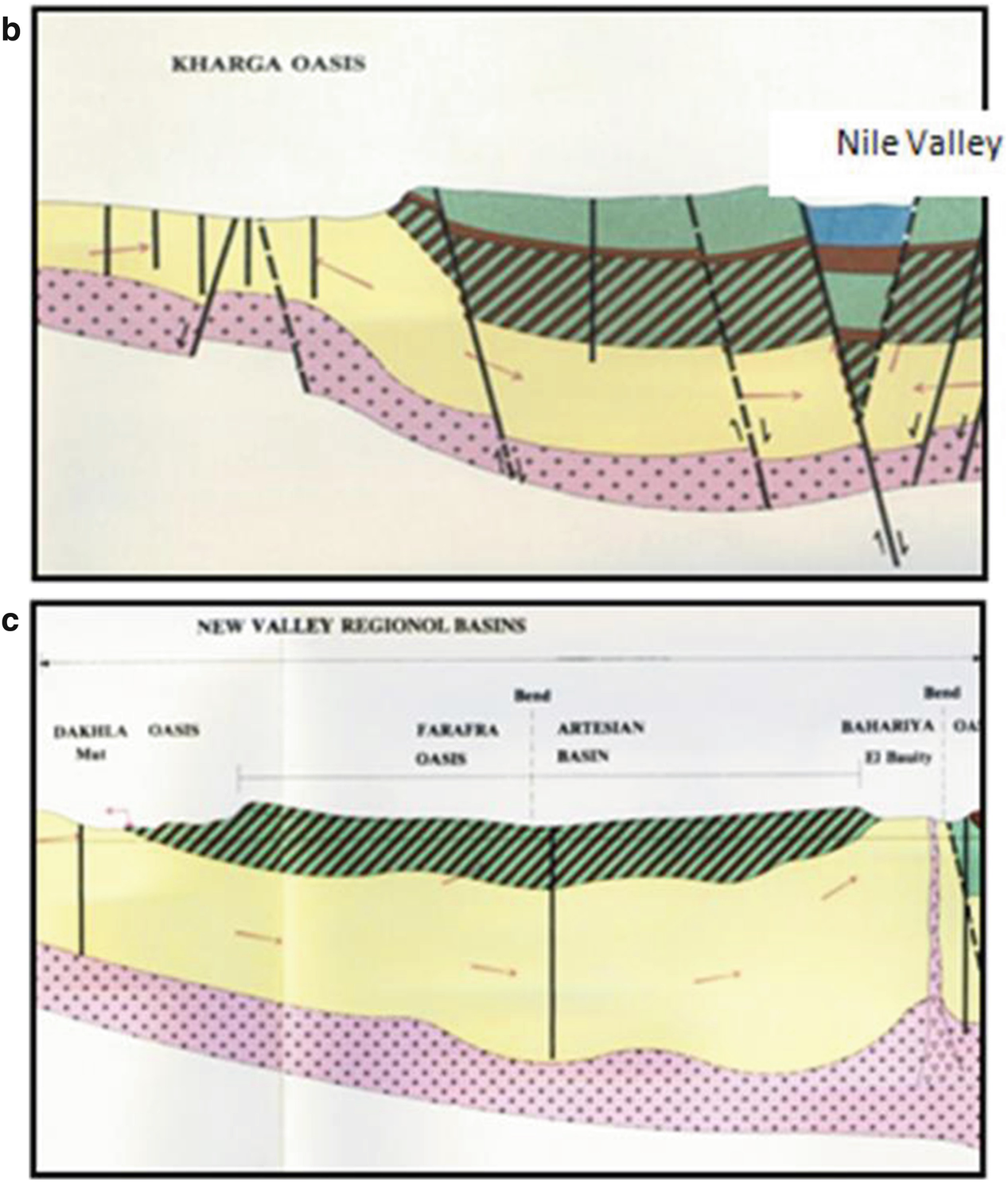
(a) Hydrogeological map of Egypt by RIGW [13] showing the study area. (b) Cross section A-A through the study area. (c) Cross section B-B through the study area
5 Numerical Simulation of the Study Area
5.1 Hydrologic Setting of the Study Area Model
In order to have more confidence in the hydrologic parameters GIS with Visual Basics model is used in order to fill the gaps in those parameters needed for the Nubian main model. Each Oasis will have a separate local model [14].
A second model is set for the entire model domain using the MODFLOW model software. The objective of the second model is to set the required aquifer boundary conditions.
Upon setting the model properties and its boundary conditions from the last models, a third final model domain was furnished including all wells replacement until the year 2010 and their discharges. It must be noted that water supply wells are added to the final model in order to have an actual groundwater management plan.
At this stage, simulating the development of the different scenarios is followed for the prediction of the related aquifer response using the latest well groups. The model will run for 100 years (2010–2110) in order to set the future water management plan for the three Oases.
5.2 Mathematical Formulation (Governing Equations)

T xx = K xx × Saturated thickness of layer in x direction (L2/T)
T yy = K yy × Saturated thickness of layer in y direction… (L2/T)
S = S s × Saturated thickness of layer
K xx, K yy (L/T) are values of the hydraulic conductivity along the horizontal principal axes in the layer. In case of the Nubian aquifer, it was found from many research works and CEDARE Report that almost the horizontal HC K xx = K yy = K
S s is the specific storage coefficient of the aquifer (L−1)
h is the piezometric head at any point in the model domain (L)
Q is the pumping rate in the model (L3/T)
S y is the specific yield or effective porosity of the unconfined aquifer.

5.3 The Model Package of the MODFLOW
Visual MODFLOW model is the software package developed by Waterloo Hydro-geological Inc. It is used to solve three- or two-dimensional groundwater flow and contaminant transport simulations with the finite difference approach.
This package combines MODFLOW and MT3D with the most intuitive and powerful graphical interface available.
Dimensioning the model domain and selecting units.
Conveniently assigning model properties and boundary conditions.
Running the model simulations.
Calibrating the model using manual or automated techniques.
Optimizing the pumping well rates and locations.
Visualizing the results using 2D or 3D graphics.
The MODFLOW software used the finite difference approach for its simulation principles. Complete explanations about using the finite difference techniques are given in [9].
5.4 Procedures for MODFLOW Implementation
5.4.1 The Model Domain
Relation between (geographical) and model coordinates
Coordinates | Geographical coordinates | Model dimension in m |
---|---|---|
X min | At Long 27° E | 0 |
Y min | At Lat 24° N | 0 |
X max | At Long 31.5° E | 460,000 |
Y max | At Lat 28° N | 440,000 |
According to the conceptual model, the MODFLOW model will have two zones. The model first zone will simulate the depleting limestone layer and the nonconductive shale layer. The model second zone will simulate the main and productive Nubian aquifer. It is a deep aquifer where its lower surface boundary is located at the basement complex surface. The model thickness will simulate the actual vertical thickness which is between the maximum ground surface elevation (little less than 700 m (amsl)) and the minimum elevation of −3,300 m (bmsl) at the basement complex surface boundary.

Topographical map of the model area

Contour lines of zone 1 bottom

Basement complex bottom layer 2
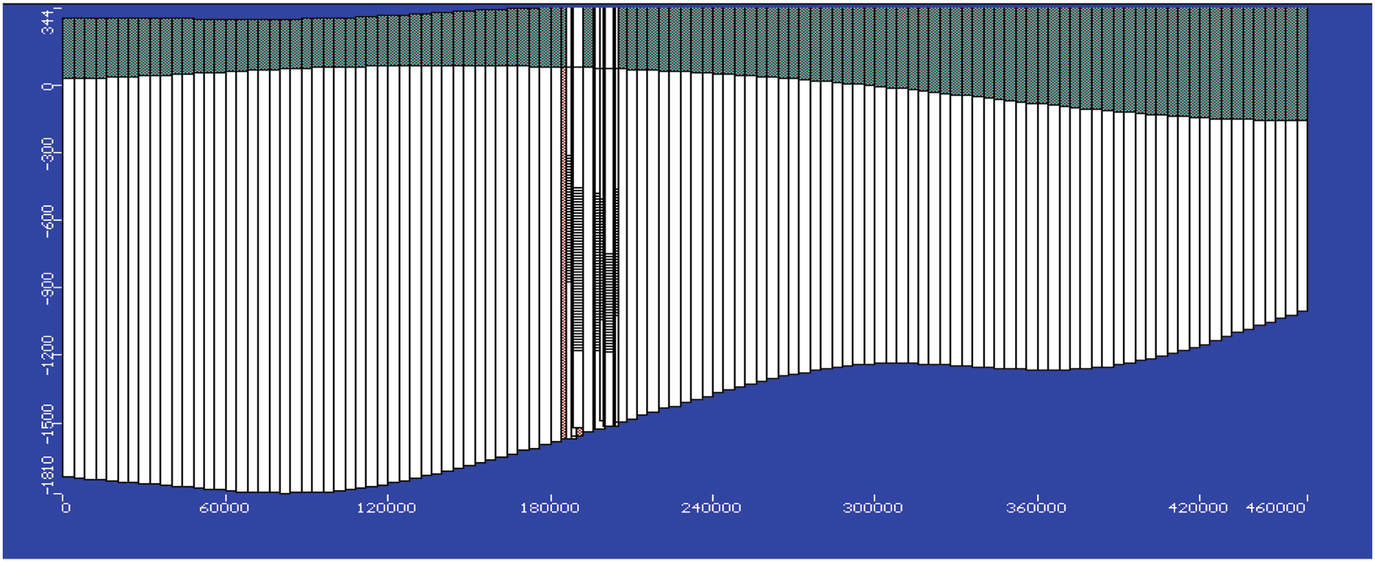
Cross section through the middle of the model domain at Dakhla Oasis (through Lat 26° N) with distance y = 220,000 m from the origin
5.4.2 Model Properties
Average hydraulic parameters of the Nubian Aquifer System (refer to CEDARE Report [15])
Region | No of Pumping tests | Saturated thickness (m) | Transmissivity (m2/s) | Hydraulic cond. (HC) (m/s) | Storage coefficient(s) (S) |
---|---|---|---|---|---|
Farafra | 8 | 2,600 | 1.20E-02 | 5.4 × 10−5 | ? |
Abu minqar | 3 | 2,500 | 5.469E-02 | 2.18 × 10−4 | ? |
Dakhla | 21 | 1,850 | 1.1E-02 | 6.1E-05 | 6.35 × 10−4 |
Kharga | 59 | 1,280 | 5.9E-03 | 2.90E-05 | 2.84 × 10−4 |
5.5 Local Models
In this research work, the local models were used to further adjust both HC and S s and find any missing data as S s. The method used for adjustment of both properties is by using the GIS with the visual basic model which was achieved by Gad and Soliman [14]. The method was used for adjustment or calibration process of both the HC and S s.
The adjustment started by using data shown in Table 3 of HC and S (obtained by CEDARE from pumping tests [6]) and comparing it with calculated data from the drawdown by Eq. (3). This equation was introduced to the data code of the visual basic (VB) which is in the GIS extension tool. Upon getting the drawdown values for the entire wells at selected network stations obtained by the VB, the GIS can draw the drawdown contours through the spatial analyst in the GIS tools as given below.
- 1.
Locate first the well group for each Oasis in a point shapefile using the selected coordinates in model domain as shown in Figs. 29, 30, and 31 in Appendix 1
- 2.
Once the well groups were located, and the well discharges are assigned to each well then the Visual Basic Code (VB Code) is designed to make a grid of points to stand for the observation points using the same coordinates of the well groups. There will be two coordinate systems (xw, yw)i for the well (i) and other coordinates for the observation points (xp, yp)k (i for the well number and k for the grid point number).
- 3.In order to calculate the drawdown at any grid or observation point, Modified Theis Equation is used in VB Code as,Table 4
New Valley extractions (1998–2010) (CEDARE Report [15])
Area
1998
2000
2005
2010
m3/s
Mm3/year
m3/s
Mm3/year
m3/s
Mm3/year
m3/s
Mm3/year
Farafra
5.05
159
2.85
90
3.5
110
4.5
142
Dakhla
8.75
276
8.75
276
10.3
325
11.85
374
Kharga
3.96
125
3.96
125
3.96
125
5.00
158
Table 5Drawdowns (DD) calculated and observed for Farafra parameters
Oasis name
Q (m3/s)
Observation well locations
Pumping time
Model DD (m)
Observed DD (m)
Note
Farafra
2.85
NF9 (Obs1)
5 years (1995–2000)
2.8
2.5
Av error 6.5%
3.5
X = 60,000 m
5 years (2005–2010)
3.8
3.4
K = 5 m/day
Y = 308,000 m
S s = 2.00E-05
5.05
10 years (1990–2000)
6.4
6.2
Fig. 8Piezometric head at various locations of wells at the New Valley [2]
![$$ {d}_k=\left(2.3{Q}_i/4 T\pi \right)\left[\log \left(2.25 Tt/{R_i}^2S\right)\right] $$](../images/437178_1_En_62_Chapter/437178_1_En_62_Chapter_TeX_Equ3.png)
d k = drawdown at an observation point k (m)
Q i = well discharge for well i (m3/day)
T = average transmissivity of the aquifer = K – Saturated aquifer thickness of the selected Oasis as given in Table 5 (m2/day)
K = the hydraulic conductivity (HC) (m/day) of the Oasis
t = selected pumping time in days
- 4.
For each well the drawdown was calculated and summed at each observation point k. This was obtained by VB code and located at the respective point. It must be noted that any observation point located at any well is discarded by the Code. The GIS will then draw the drawdown contours as shown for the three Oases (Figs. 9, 11, and 13). Notice that the drawdown zero contours were located inside the Oases boundaries. This condition was found in this respect when the pumping time will not exceed 10 years for any Oasis in order to prevent any groundwater interference between Oases. Figures 29, 30, and 31 in Appendix 1 give both Geographic coordinates and the model coordinates system for each well at Farafra, Dakhla, and Kharga Oases, respectively.
- 5.The adjustment process started by selecting the drawdown values for one or two wells near the center of each Oasis. Then compare the results with the observed and calculated values as mentioned before. In this research, it was found that Storage Coefficient S was much more sensitive on the drawdown than the H.C. K, so S s was selected in the calculations for its variation to try to narrow the gaps between both observed and calculated drawdown values (Figs. 9, 10, 11, 12, 13, and 14).Fig. 9
Drawdown contours for Farafra. Total Q = 5.05 m3/s run for a maximum period of 10 year as given in Table 5
Fig. 10Farafra aquifer correlation values (refer to Table 5)
Fig. 11Drawdown contours of Dakhla Oasis using GIS and VBC (observation well locations Obs2 is at Mut airport)
Fig. 12Relations between observed and model data for DD in Dakhla Oasis. See for observed well locations Obs2
Fig. 13Drawdown for 5Y contours for Kharga (Obs3 stands for well observation 3)
Fig. 14Kharga Oasis Parameter adjustment
Drawdowns (DD) calculated and observed for Dakhla Parameters
Oasis | Observation well with its model coordinates | Q (m3/s) | Pumping time | Model DD (m) | Observed DD (m) | Note |
---|---|---|---|---|---|---|
Dakhla | Airport Mut (Obs2) | 10.3 | 5 years (2005–2010) | 6.0 | 5.5 | Av error 5% |
X = 200,170 m | 3 years (2005–2008) | 4 | 3.6 | K = 8 m/day | ||
Y = 156,354 m | 8.75 | 5 years (2000–2005) | 5.3 | 4.8 | S s 8.00E-06 |
Drawdowns (DD) calculated and observed for Kharga Parameters
Oasis name | Q (m3/s) | Observation well with its model coordinates | Pumping time | Model DD (m) | Observed DD (m) | Note |
---|---|---|---|---|---|---|
Kharga | 3.96 | Garmashin 23 (Obs 3) X = 360,900 m Y = 104,600 m | 5 years (2000–2005) | 3 | 2.7 | Av error 7.5% K = 3.25 m/day S s = 2.40E-05 |
10 years (2000–2010) | 6 | 5.7 | ||||
Garmashin 20 (Obs4) X = 362,000 m Y = 93,170 m | 5 years (2000–2005) | 2.5 | 2.6 | |||
10 years (2000–2010) | 5.5 | 5.2 |
Regarding the local models of the three Oases, one can state that the difference between the HC values obtained by the GIS/VB and CEDARE values is close. However, the storativity values were adjusted, and the missing storage values were obtained. For this reason, Soliman [12] found that the local model results gave more confidence in the obtained model properties. However, a regional model for the three Oases is set to calibrate the model boundary conditions.
5.6 Regional Model
5.6.1 Model Preparations
The hydraulic conductivity and specific storage adjustments using the GIS with the Visual Basic extension program were found successfully for each Oasis. The regional model using the MODFLOW domain was built particularly for the calibration stage in order to adjust the model boundary conditions. The model domain would contain the well network and its discharges for the period of years (2000–2020). The well discharges are as given in Table 4 which was obtained from CEDARE Report. The well network location is found in Figs. 29, 30, and 31 in Appendix 1.

Initial head contours for Dakhla Basin developed from the Hydrogeological Map by RIGW
The final adjusted values for model properties
Location | S s (1/L) | K (cm/s) |
---|---|---|
Farafra | 2.00E-05 | 6.00E-05 |
Dakhla | 8.00E-06 | 8.00E-05 |
Kharga | 2.40E-05 | 4.00E-05 |

HC > distribution in the model domain

Specific storage distribution in the model domain
5.6.2 Boundary Conditions
As a first glance to the model area, the boundary of Assiut Governorate area at the upper eastern corner of the model domain can be taken as a constant head boundary due to the presence of some seepage flow as reported by the groundwater Institute in this location. This boundary is also formed from series of faults between the Nile Basin alluvial material and the Nubian aquifer as seen in Fig. 3b. In spite of both aquifers are different from each other, yet there is a possibility that both aquifers are hydraulically connected as reported also by the groundwater Institute in this location.
Since the groundwater level fluctuates around 50 m (amsl) in Assiut alluvial aquifer, therefore a constant head boundary of 50 m can be taken at the Nile Basin boundary at north east of the model domain.
Upon looking at the initial head map, two other sources of boundaries may be postulated. These are the southwestern corner (BC2) and the southeastern corner (BC3) of the model domain (Fig. 21 where more details are given). The rest of the model boundaries are approximated as flow lines.
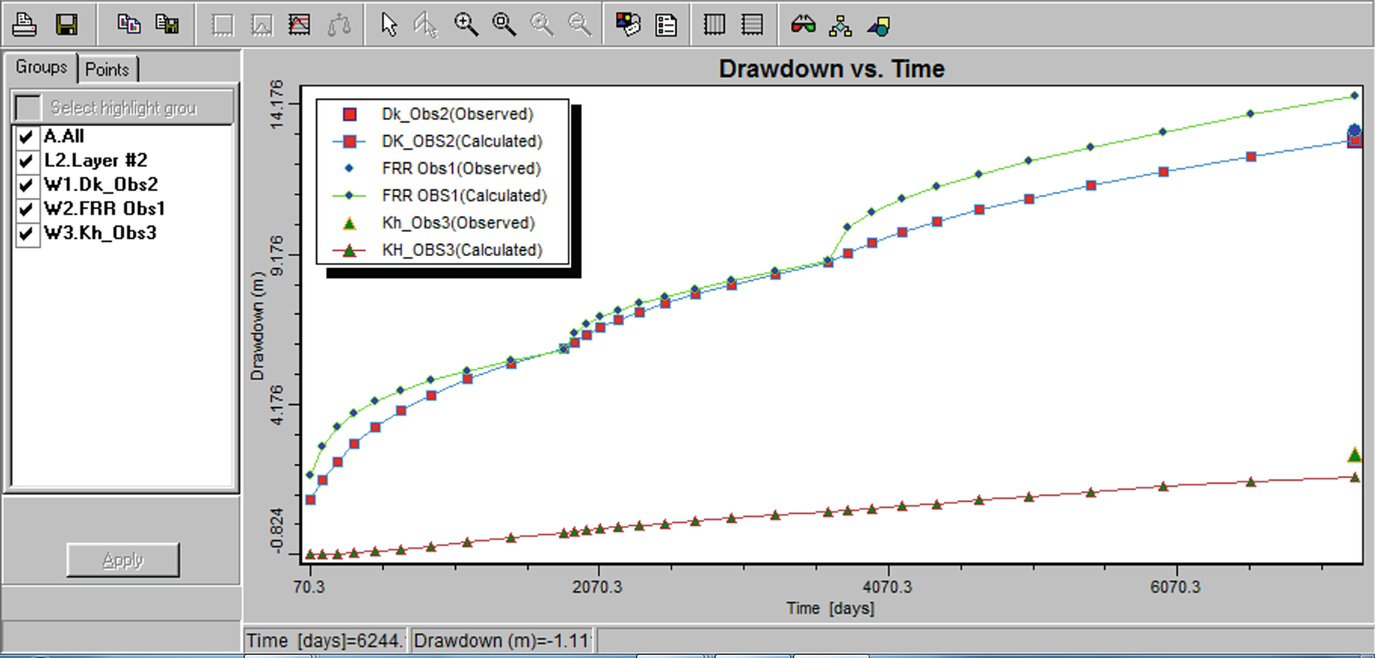
DD vs time at the tutee Oasis
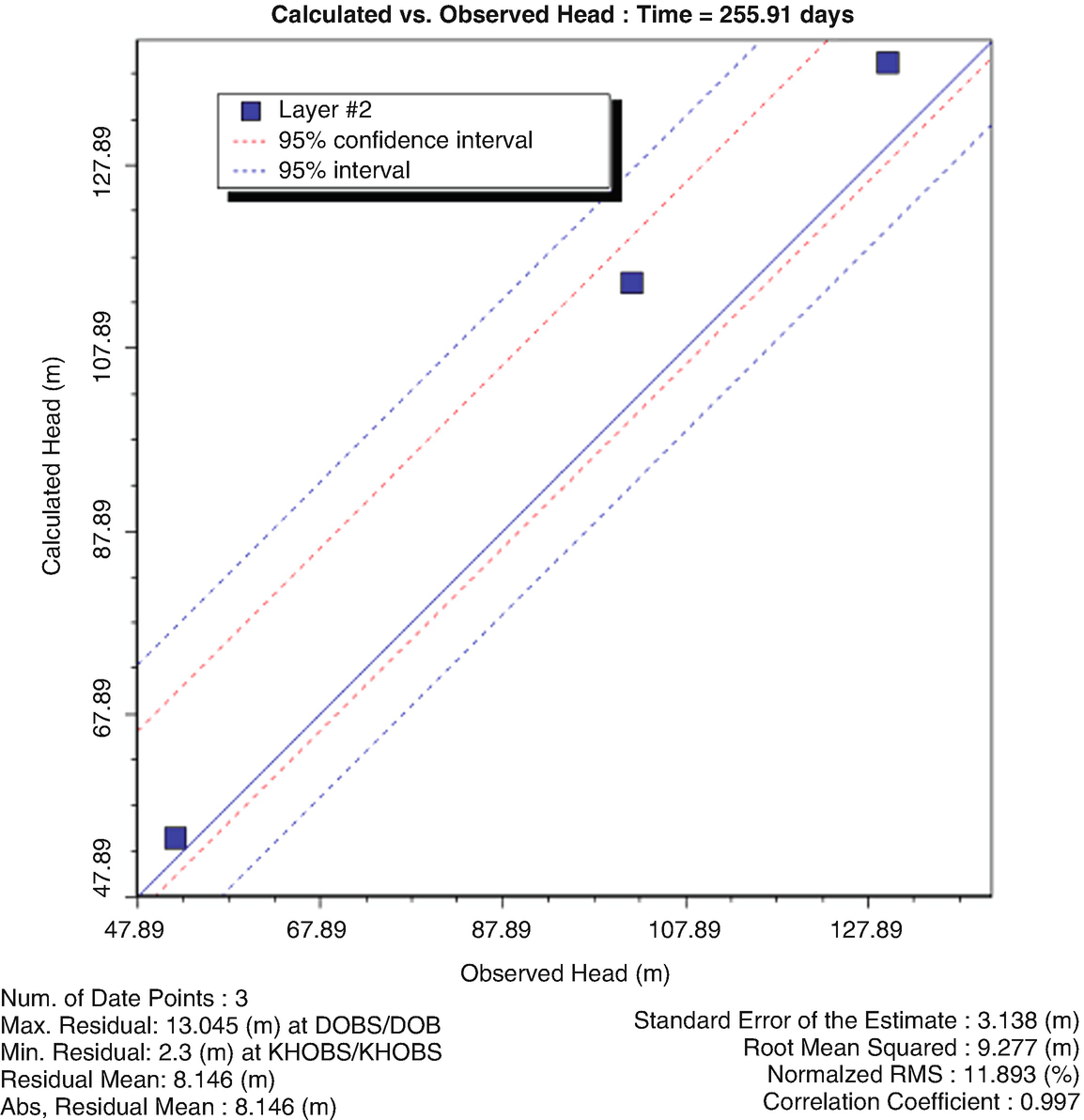
Calculated vs observed head at the observed wells in the three Oases. The observed data is from CEDARE drawdown data

Piezometric head map at 2020 resulted from the calibration process
- 1.
- 2.
Boundary condition BC1 is set at the Nile Basin boundary south of Asyut Governorate as mentioned before.
- 3.Boundary conditions BC2 and BC3 which took L section are set as follows:
- (a)
The number of cells of BC2 = 11 cells in the vertical direction and 8 cells in the horizontal direction. The average potential head was found from the calibration trials to be 180 m (amsl)
- (b)
Also from the calibration trials, the L section BC3 boundary was found to have four vertical cells and three horizontal cells. The average head value was also found to be =75 m (amsl) resulted from the calibration process.
- (a)

Initial head in the year 2000, boundary conditions and the three observation wells used in the model domain

Piezometric head map at year 2010 used as initial head of final model and showing also the three observation well locations
5.7 Final Model and Analysis
The final model domain is arranged to include all the wells which were replaced and constructed recently (September 2010) in the three Oases. Most of the new wells were drilled during 2008–2010. The boundary condition was already installed as mentioned in the regional model study.
Tables 9, 10, and 11 (see Appendix 2) include all the wells and its locations that have been obtained from the Groundwater Sector in the Ministry of Water Resources and Irrigation. It must be noted that all the water supply wells were also included in the tables. Two scenarios are adopted to run the final model as given below.
Scenario 1
In order to study the groundwater management in Dakhla Basin, an optimization study should be done. The optimization procedure of the Groundwater Sector of the Ministry of Irrigation is used. The process first of all deals with the Nubian aquifer in Dakhla Basin as a nonrenewable groundwater reservoir.
In this kind of aquifer, groundwater development should follow the groundwater mining system rules. This means that any groundwater exploitation should postulate constrictions for development. The Groundwater Sector of the Ministry adopted the main constriction in the groundwater management plan is that the maximum drawdown of any well field in such kind of aquifer should not be greater than 60 m resulted from running the pumping wells for 100-year period plus the historical drawdown value that exists before the 100 year period. This postulation took mainly into consideration the economic and the timescale factors. The timescale factor is postulated to have the 100-year period as enough to investigate for an additional water resources system to cover the lack of the water requirement of the area.
Well number 307 in Farafra was used to get the historical drawdown for Farafra Oasis.
Well Mut Airport was used to get the historical drawdown for Dakhla Oasis.
Garmashine well 23 was used to get the historical drawdown for Kharga Oasis.

Model outcome of head contours of 100-year period
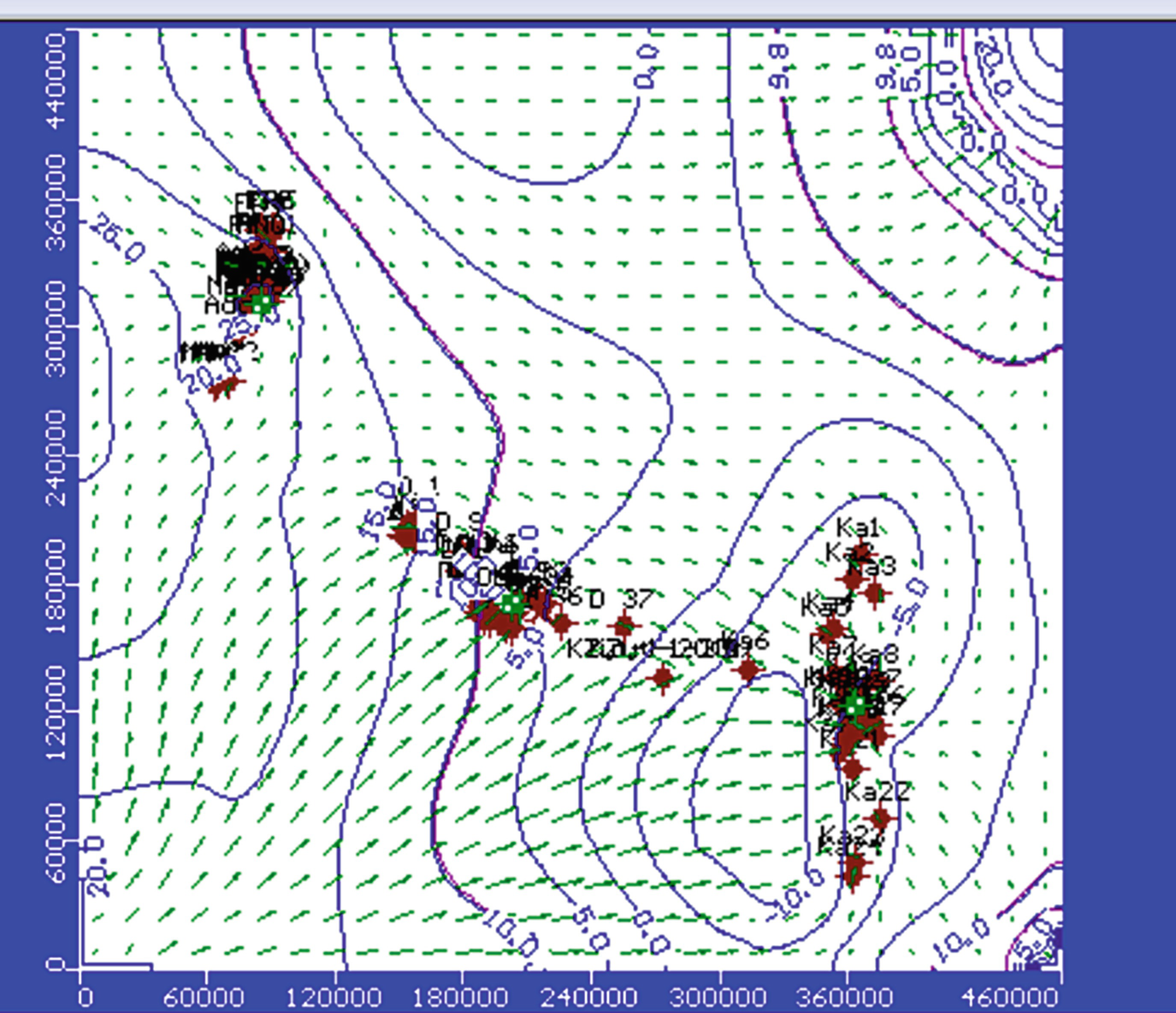
Drawdown contours for 100-year period

Drawdown vs time at the three observation wells in the three Oases
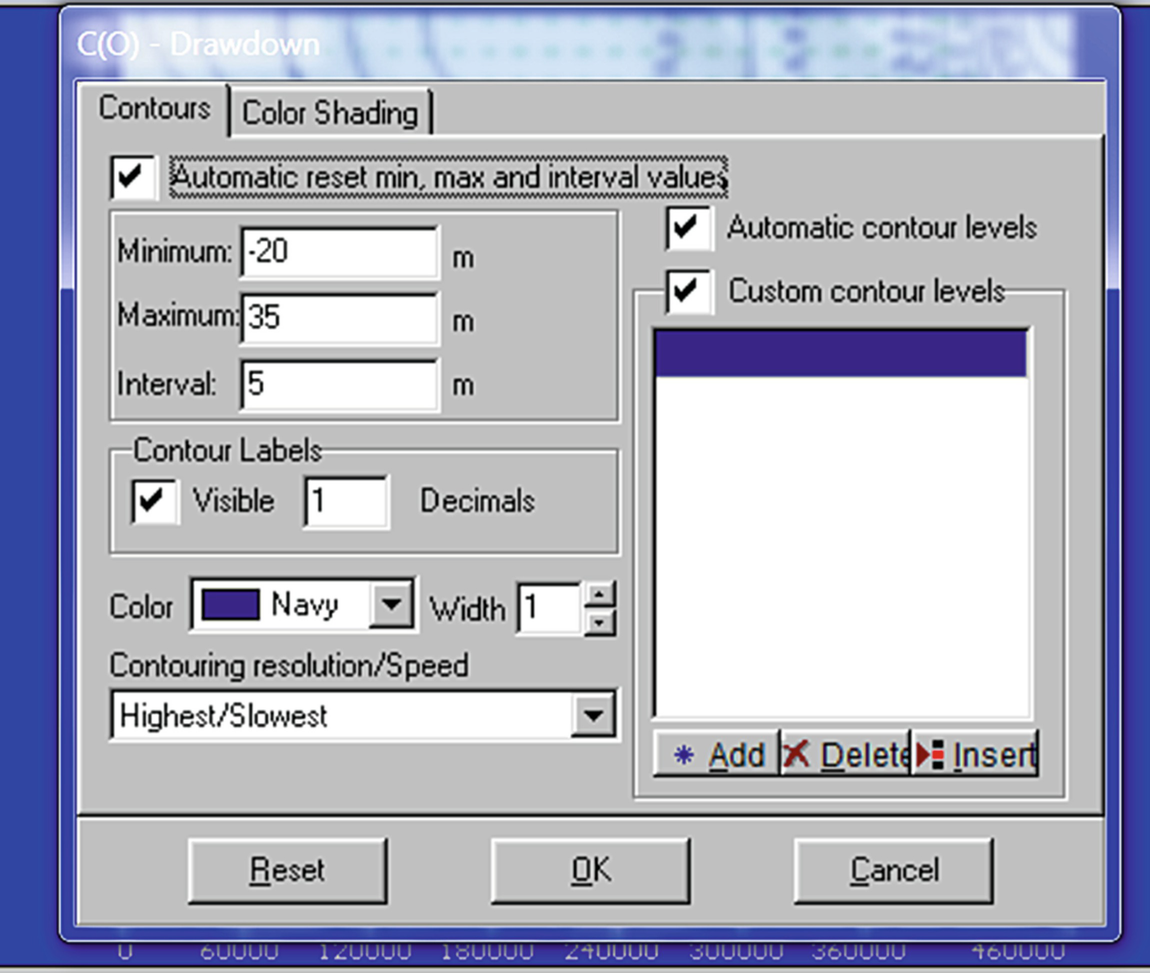
Maximum and minimum drawdowns at the year 2110
The value of drawdown −20 m denotes the maximum drawdown in Kharga Oasis whereas the drawdown value of 35 m denotes the maximum drawdown near Farafra area. The negative sign in the drawdown was only denoted algebraically by the model. This is also due to the presence of the 0.0 drawdown contour where any value above 0.0 is positive, and any value below 0.0 is negative. The 0.0 contour can be resembled by an island encountered by water, postulated as 0.0 level, and any drawdown inside the island is considered as having a negative value. It can be noticed that the flow inside the 0.0 contour approaches the steady state condition.
This can be visualized by subtracting the piezometric head in the year 2010 minus the piezometric head at the year 2110. The reason for that is due to the reduction of the well discharges in Dakhla Oasis in the year 2010 by 50% as given in Table 10 in Appendix 2. The model study considered this reduction until the year 2110.
According to optimization procedure for Kharga Oasis the maximum drawdown was found to be 20 m in 100-year period. The historical drawdown value started from the year 1960 which was considered as the beginning of New Valley development may be adopted from Fig. 8.
The Garmashine well 23 in Fig. 8 (see its location in Fig. 31 in Appendix 1) was used to give the historical drawdown. This value was found to be 42 m.
Therefore, the total drawdown in this respect until the year 2110 would be 62 m which is considered as critical drawdown value by the Ministry of Irrigation optimization process.
The historical drawdown in Farafra Oasis using Well 307-Farafra gave 26 m (see Fig. 8). On the other hand, the 100-year maximum drawdown in Farafra was found to be 35 m (Fig. 26). Therefore, the total drawdown became 61 m which do not favor any discharge increase. This is according to the Groundwater Sector postulation as mentioned before.
Concerning Dakhla Oasis, the 100-year maximum drawdown was found to be 24 m (Fig. 24). On the other hand, the historical drawdown of Mut Air port well gave 34 m. Accordingly, the total drawdown gave 58 m. Therefore, Dakhla Oasis allows further expansion which is discussed in the Scenario 2.
Scenario 2

Piezometric head map of the model output at the year 2110 after increasing the well discharge by 15%
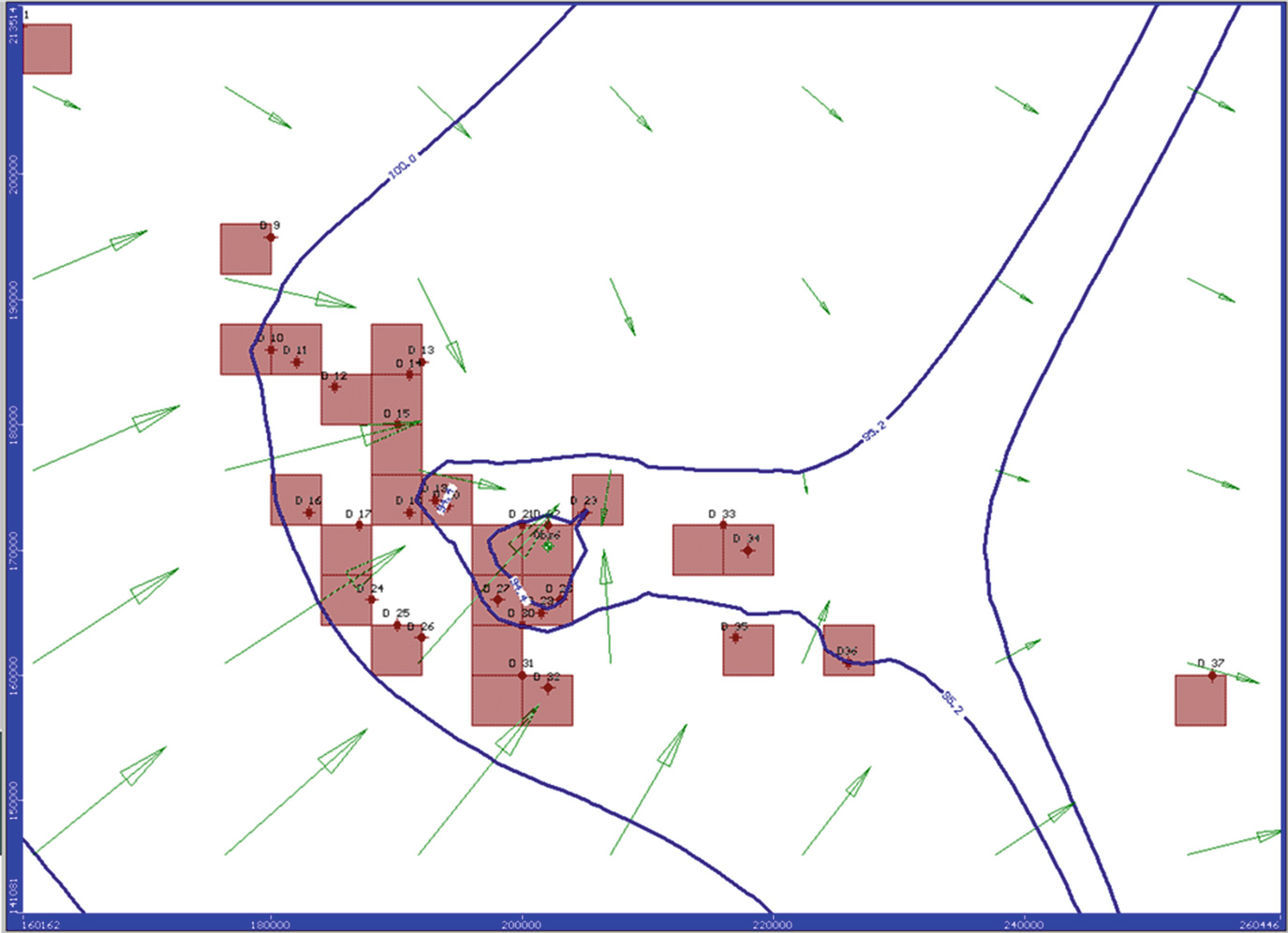
Zooming of piezometric head map of Fig. 24 at Dakhla Oasis Center
6 Conclusions
The study area in this research is located in the middle part of Egypt’s Western Desert. It lies between latitudes 24°–28°.N and longitudes of 27°–31.5° E. It includes the three Oases, Farafra, Dakhla, and Kharga, which are part of the New Valley area. It covers an area of about 440 km long by 460 km wide. It is equivalent to an area of 202,400 km2 which is named as the Dakhla Basin. The western limestone plateau bound it to the east and north of Kharga and Dakhla region.
The main objective of this research is to evaluate the groundwater resources and its potentiality for development in the study area. The aquifer of this groundwater resource is mainly the deep Nubian Sandstone Aquifer which is located in the three Oases. Due to the scarcity of the groundwater recharge in the Nubian aquifer, it is hydrogeologically treated as groundwater mining aquifer [12].
Three groundwater models were used in this respect. A local model using GIS accompanied by the visual basic was first prepared to adjust the model properties. A second model using MODFLOW was furnished to calibrate the boundary conditions of the main model. The third model was using the main model as a final model to find the main objectives which are the groundwater potentiality and its management in Dakhla Basin The model was run for a 100-year period to suit the groundwater mining conditions.
As an outcome of the model study for the optimum management strategy in Dakhla Basin, two scenarios were postulated. The objective is to maintain the resultant drawdown in the development areas within an acceptable range for economic purposes besides obtaining the most appropriate area for development in Dakhla Basin.
The salient fact from this study showed that Dakhla Oasis is the only place in the New Valley area where land reclamation development can safely take place.