nine
water
“And the fish suspending themselves so curiously below there and the beautiful curious liquid And the water plants with their graceful flat heads, all became part of him.”
—WALT WHITMAN,
“THERE WAS A CHILD WENT FORTH”
My mother grew up by the Vermilion River and my father alongside Lake Michigan. I have, therefore, no familial connection to the Illinois River, no handed-down tales to pass on. In getting to know this river I was raised beside, I’ve relied as much on library research as on my own observations. These sometimes tell two different stories.
In one archival photograph from the early 1900s, four men and two boys stand on the river’s edge beside what looks to be an immense pile of stone butterflies. The solemn fellow in the foreground holds one of them, wings spread, in the palm of his upraised hand. The others in his outfit stand in the background, stiff and expressionless as fence posts. These men are, in fact, mussel gatherers showing off their catch. They will sell their heap of shells to one of fifteen button factories that line the shores of the lower Illinois River.
By 1948, the last one had closed. Pollution and overharvesting killed off the mussels, and plastic replaced mother-of-pearl in the production of shirt buttons. The species depicted in the photograph are as alien to me as the process of turning them into objects of human attire.
In 1948, diving ducks also began to disappear from the Illinois. Ring-necked duck, canvasback, ruddy duck, and lesser scaup: these are species I learned to identify from stuffed specimens and in distant field sites. I have been trained to recognize their patterns of coloration and differently pitched calls, but I do not recognize them as fellow inhabitants of the river system I grew up in—although it served for centuries as the fly-way for their migrations.
My newlywed parents began building their house on the bluff in 1955. In this same year, the valley’s population of scaups (“highly social . . . note the purplish gloss on head . . . shows bold white stripe on secondaries . . . calls are short low croaks”) plummeted to zero. Researchers attribute their disappearance to the synchronous demise of the river’s fingernail clams. Likely poisoned by organochlorine contamination of the river’s sediments, they had served as the ducks’ major food source. The clams have never come back, either.
Dabbling ducks, such as wigeons (“pale grey head and bluish bill”) and gadwalls (“rarely congregates . . . call, very low and reedy”), feed on the seeds of aquatic plants. Their departure from the Illinois corresponds to the arrival of herbicides. As agriculture became increasingly mechanized and chemically dependent, the flow of silt and weed killers from surrounding fields created waters barren of all such vegetation. Wild celery, coontail, and sago: these species, according to old accounts, once flourished in the quiet, shallow waters of Peoria Lake. They vanished completely in the 1950s, along with the birds that ate them. I don’t know how to recognize these plants.
The story of the fish begins fifty years earlier. At the turn of the twentieth century, over two thousand commercial fishers worked the Illinois and supplied their harvests to markets as far away as Boston. Special fishing trains also carried sport fishers to and from Havana, the river town just downstream of Pekin. As measured by pounds of fish caught per mile of stream, the Illinois was considered the most productive inland river in North America.
This remarkable fecundity was a gift of geology. Much of the Illinois flows through a floodplain left behind by the ancient Mississippi. This flat pan of ground allowed the river to spread out a far-flung web of interconnected backwaters—the perfect nursery, spawning grounds, and winter refuge for fish. In the summer, periodic droughts firmed up the bottom, improving conditions for vegetation. The plants, in turn, deterred the wind from stirring up sediment during periods of spring and autumn flooding, when the river poured itself into the twisting sloughs, swales, potholes, marshes, and subsidiary lakes that surrounded it.
Then came the Chicago Sanitary & Ship Canal. This part of the story is a kernel of central Illinois lore. The S&S Canal opened January 17, 1900, and effectively connected Lake Michigan to the Illinois River, creating a continuous navigational route down to New Orleans. This is the meaning of the second S (“ship”). The first S (“sanitary”) refers to the flushing of Chicago’s wastewater into this canal and, from there, through the Des Plaines River and into the Illinois. Consequently, the level of the Illinois rose considerably. Backwaters flooded and stayed flooded. Bottomland groves of pin oak and pecan trees died. A wave of industrial pollution moved slowly and inexorably south (reaching Pekin around 1915), and downstream residents protested vociferously. Finally in 1939, the U.S. Supreme Court was moved to reduce by one-half the diversion of water into the Illinois. In the meantime, locks and dams began shaping the river into a series of stepped navigational channels. By World War II, the river resembled its present configuration. Straightened, leveed, drained, and dammed, the Illinois River became a sewage canal for industry and a barge canal for shipping—S&S. A report published the year I turned seven features a photograph of Illinois River fish with open sores and fins eroded down to stumps.
The federal Clean Water Act of 1972 brought a modicum of improvement to the Illinois River. As annual amounts of industrial waste released into the river declined, water quality improved. The long-term ecological effects, however, are less clear. Like a cloth already frayed, the river shows signs of continued damage even at lower levels of stress. Recovery has been uneven, at best. Mussels have returned to some parts of the river, and fin erosion is a less common problem in fish. On the other hand, the level of pesticide contamination remains high, and aquatic plants have been unable to reestablish themselves.
In the Upper Illinois, fish advisories continue to caution sport anglers to severely limit—or eliminate—their consumption of fish known to contain high levels of cancer-causing chemicals. These warnings, most strict for children and women of reproductive age, are especially emphatic about the danger of eating large fish, in which the amplifying effects of biomagnification have had the longest time to operate. The bigger the fish, the more concentrated the poison.
This, then, is the river I know. Isolated from its floodplain by levees, factories, and farms, the Illinois River flows alone. Barge convoys, big as football fields, suck the river into their wakes and then send it crashing against the banks. The accompanying tugs churn the river like egg beaters, constantly resuspending toxic materials. These include vintage chemicals—PCBs, DDT, dieldrin, chlordane, heptachlor—as well as more contemporary pollutants contributed by industrial discharges, chemical spills, and farm runoff. The resulting waves slosh silt and poison into whatever fish-spawning backwaters still exist.
More than 350 different spills of hazardous substances into the waterway were reported between 1974 and 1989 alone. The continued absence of bottom-dwelling animals makes the aquatic biologist Doug Blodgett of the Illinois Natural History Survey in Havana suspect that such spills remain frequent. Killing as it goes, each spill creates a toxic pulse that moves through a given section of stream within hours. Once-a-month monitoring does little to detect most of these transient accidents. Such spills are, of course, in addition to routine industrial discharges.
The fastest way to get to the Illinois River from my parents’ house is to follow Derby Street into Normandale. This was the route I used in high school—unbeknownst to my parents, who considered the riverfront dangerous.
Derby Street itself is an avenue of nostalgia and munitions. Store-fronts with names like Karen’s Kountry Kottage and Grandma’s Feather Bed alternate down the block with various gun and ammo shops. One is notable both for the missiles on display in the parking lot and for the half of a Jeep (with GI mannequin positioned in the driver’s seat) mounted trophy-style on the side of the building. Actually getting to the river from here is tricky. It requires a stroll through the subdivision, a climb over chain-link fencing, and a firm decision to ignore No Trespassing signs. Then, abruptly, there is the water—brown, familiar, blank.
Silence is comfortable here. The river embraces silence. The Illinois River seemed to me, as a teenager, not so much dangerous, or even endangered, as reassuring.
Standing here now, aware of all that is not here, I know myself as a natural historian of ghosts. Since 1908, twenty species of fish have disappeared from this river. One in every three native amphibian species have been completely, or almost completely, extirpated from the state. In their extinction, they join one in every five crayfish and more than half the species of mussels. The river reminds me of a poem by Robert Frost that asks, “What to make of a diminished thing?” and provides no answer.

Public drinking water is regulated nationally by the Safe Drinking Water Act, which became law in 1974. The act directs the EPA to set maximum contaminant levels that represent the highest limits allowable by law of particular toxic substances in public water supplies. In this way, all public drinking water is monitored on a regular, ongoing basis. Different contaminants have different legal limits. For example, there is one maximum contaminant level for the herbicide atrazine (3 parts per billion) and another for the dry-cleaning fluid perchloroethylene (5 parts per billion). The maximum contaminant level for PCBs is 0.5 parts per billion, while those for the banned pesticide chlordane and the PVC feedstock vinyl chloride each stand at 2 parts per billion. The legal limit for the phthalate plasticizer DEHP is 6 parts per billion. Since 1999, amendments to the law compel water utilities to inform customers, in their water bill and at least once a year, what pollutants have been detected in their drinking water and whether water quality standards have been violated. According to these reports, 10 percent of the nation’s drinking water systems are out of compliance with EPA standards.
For at least two reasons, this figure may underrepresent the magnitude of the problem.
First, as with pesticide regulations, the legal limits for each chemical have been arrived at through a compromise between public safety and economics. Maximum contaminant levels are not solely a health-based standard. Instead, they take into consideration cost and the ability of available technology to reduce contaminants to particular levels. These then become the legal benchmark. For many chemicals, two numbers exist: the enforceable maximum and the health-based, maximum-contaminant-level goal. The enforceable values for the carcinogens benzene, vinyl chloride, and trichloroethylene, for instance, have been set at 5, 2, and 5 parts per billion, respectively. Their maximum-contaminant-level goals, however, are all zero.
Like an accountant who proficiently measures and records individual values but fails to sum the results, this system of regulating contaminants in water suffers from a constricted one-chemical-at-a-time vision. It ignores exposures to combinations of chemicals that may act in concert. The carcinogen arsenic, for example, occurs naturally in some groundwater and has a maximum contaminant level. However, if water that contains arsenic is also laced with traces of herbicides, dry-cleaning fluids, nitrates, or industrial solvents—even at concentrations well below their respective legal limits—the resulting mixture may well pose hazards not recognized by a laundry list of individual exposure limits. Exposure to one compound may decrease the body’s ability to detoxify another, for example.
This system of regulation is also blind to the many common chemicals in drinking water that have no maximum contaminant levels. As of 2009, enforceable limits had been established for only 90 contaminants. All the rest are unregulated and therefore not routinely monitored. For example, of the 216 chemical pollutants identified as breast carcinogens in animals, at least 32 are found in drinking water, but only 12 of them are regulated under the Safe Drinking Water Act. At least 20 breast carcinogens known to be in drinking water are not regulated. Also federally unregulated are pharmaceuticals and ingredients found in personal care products, such as shampoo, makeup, insect repellants, and deodorants. Furthermore, when maximum contaminants do exist, they are not always set with cancer in mind. For example, the maximum contaminant level for nitrates—anhydrous ammonia’s calling card—is 10,000 parts per billion (10 parts per million) for finished drinking water. But this standard was set to protect formula-fed babies from developing anemia in response to acute nitrate poisoning. It does not reflect new knowledge about the ways in which nitrates are converted in our digestive tract to carcinogenic N-nitrosamines (a transformation we discussed in Chapter Seven). Recent evidence suggests that nitrates, at levels below their legal limit in drinking water, may raise cancer risk.
To the question, then, of whether drinking water is regulated on the basis of contemporary scientific knowledge, the answer is no. Perhaps most revealing of all is the fact that regulation for some contaminants is based on the annual average of four quarterly measurements. In other words, drinking-water standards are violated only when the yearly average concentration of said contaminant exceeds its maximum contaminant limit. A one-time transgression does not automatically create a violation. This distinction is important in the Midwest, where herbicide concentrations in drinking water drawn from rivers and streams often reach hair-raising levels during the spring quarter, the months of planting and rain. In 1995, in the first study of its kind, researchers sampled water from faucets in kitchens, offices, and bathrooms every three days from mid-May through the end of June in communities throughout the Corn Belt. Herbicides turned up in the tap water in all but one of twenty-nine towns and cities. Atrazine exceeded its maximum contaminant level in five cities, including Danville, Illinois, where its concentration in water reached six times the legal limit. (Danville is southeast of Pekin, near the Indiana border. My Uncle Jack grew up there.) A 2009 study showed that not much has changed since then: atrazine in fifty-four public drinking water sources across the midwestern and southern United States peaks at concentrations above the maximum contaminant level.
Biologically speaking, we live only in the present. Our bodies do not respond to contaminants on the basis of averages; they must cope the best they can with the load of contaminants already received as well as with those streaming in at any given moment. If, during the period of April through June, a girl living in rural Illinois drinks enough weed killer to overwhelm her body’s ability to detoxify it, and if, as animal evidence suggests, these chemicals are capable of altering development of her breast tissue, then the damage has been done, regardless of what happens during the months of August, October, or January.
Many researchers now believe that exposure to even minute amounts of carcinogens at certain points in early development can magnify later cancer risks greatly. What are the implications for the unborn child who happens to reach one of these key points at the same time a bevy of farm chemicals in the local water supply is reaching its peak? What are the implications for the adolescent girl whose breast buds start to form during this particular quarter of the calendar year?
Exposure to waterborne carcinogens is more commonplace than many people realize. In the same way that intake of airborne pollution involves the food we eat as well as the air we breathe, intake of contaminants carried by tap water involves breathing and skin absorption as well as drinking. These alternative routes are especially important for the class of synthetic contaminants called volatile organics—carbon-based compounds that vaporize more readily than water. The dry-cleaning solvent perchloroethylene is a common one. Most are suspected carcinogens.
We have already seen in Chapter Eight how volatile organic compounds combine with nitrogen oxides to create poisonous ground-level ozone, a major air contaminant. As a contaminant of tap water, they present additional dangers. Volatile organics are easily absorbed across human skin and enter our breathing space when they evaporate. The higher the water temperature, the greater the rate of evaporation. Humidifiers, dishwashers, and washing machines all transform volatile waterborne contaminants into airborne ones, as does cooking. These sources of exposure are thought to be particularly worrisome for infants, and for women home all day engaged in housework.
The simple, relaxing act of taking a bath turns out to be a significant route of exposure to volatile organics. In at least two studies, the exhaled breath of people who had recently showered or bathed contained elevated levels of volatile organic compounds, including chloroform. In fact, a ten-minute shower or a thirty-minute bath contributed a greater internal dose of these volatile compounds than drinking half a gallon of tap water. Showering in an enclosed stall appears to contribute the greatest dose, probably because of the inhalation of steam.
The particular route of exposure profoundly affects the biological course of a contaminant within the body. The water that we drink and use in cooking passes through the liver first and is metabolized before entering the bloodstream. A dose received from bathing is dispersed to many different organs before it reaches the liver. The relative hazards of each pathway depend on the biological activity of the contaminant and its metabolic breakdown product, as well as on the relative sensitivity of the various tissues exposed along the way.
The importance of showers as an exposure route to drinking water contaminants was the result of an accidental discovery. In 1984, investigators who were looking into the dumping practices of an electroplating company in Rockford, Illinois, uncovered a plume of chlorinated solvents in the groundwater. A municipal well and 150 private wells were contaminated. Levels varied but in some cases exceeded five hundred parts per billion. Southeast Rockford was thus catapulted onto the Superfund National Priorities List. (The electroplating company specialized in brass fittings for caskets.)
Five years later, researchers found elevated levels of these same chemicals in the air space of homes receiving water from the affected wells—and in the blood of their human occupants. Curiously, blood levels correlated more closely with household air levels than with actual water levels. Air levels, in turn, were roughly correlated with length of “shower run times.” These results were based on a small study population and therefore have low statistical power. However, they support the notion that inhalation contributes more significantly to overall body burden of volatile organic compounds than drinking—even when water contamination is dramatic. Bottled water, by itself, is not the answer.
In 1990, epidemiologists looking at cancer maps of Illinois noticed high death rates from bladder cancer in the northwest portion of the state. Further investigation confirmed the excess incidence but found it was largely confined to one town within one ZIP code. That town was Rockford.
Some time ago, I came across a survey form used in 1918 by Illinois inspectors of wells and cisterns. Among its many questions, one required measuring the distance between the water source under inspection and all possible sources of pollution. Singled out for specific mention were feedlots, privies, stables, cesspools, and “dumping grounds for slops.” The survey also inquired whether small animals could fall in at the top and, most important, whether any cases of typhoid fever had ever been attributed to use of this water.
The survey’s approach to protecting drinking water seemed to me to show remarkable foresight. The thrust of its questions reflect an understanding about the relationship between the safety of drinking water and the kinds of activities that go on near the source of that water. “What care is taken in collecting and storing water?” “State general condition of health of those using water.” “Is the drainage from all these places toward or away from the Well, Spring, or Cistern?” “If there is any other possible source of pollution, state it.” Apparently, somewhere in the transition from the age of bacterial contagion to the age of chemical carcinogens, this type of consciousness was lost.
A lengthy report on groundwater quality in my hometown was released in 1993. It contains a detailed description of contamination in two of Pekin’s seven drinking-water wells. Located near the river, both wellheads are close to various industrial sites, many underground storage tanks, and the local sewage treatment plant. The chemicals detected in the water—tetrachloroethylene and 1,1,1-trichloroethane—could have migrated in from any number of possible sources. Both are suspected carcinogens. The report’s assessment team expresses specific concern about a site on Second Street once occupied by Valley Chemical and Solvents Corporation. Upon closing its doors in 1989, Valley Chemical left behind a shameful trail of soil and water contamination. Its property was, you might say, a dumping ground for slops.
The city of Pekin responded swiftly to this report. Committees were established and city ordinances proposed. A course on groundwater protection was even added to the public grade school curriculum. Before all this flurry of activity, however, the initial reaction was one of astonishment. “Nothing has been done through the years to protect that aquifer,” the mayor of Pekin admitted in the newspaper. “Nobody really ever thought about it. We always had good water and nobody ever thought that would change.”
“Have any cases of cancer ever been attributed to use of this water?” is a more difficult query than one asking about typhoid fever cases. No one is more knowledgeable about the nature of this difficulty than Kenneth Cantor, an environmental epidemiologist and senior scientist at the National Cancer Institute. Cantor has studied the relationship between water pollution and human cancer for much of his career.
In the introduction to a review of the subject, Cantor and his colleagues note that the various studies of cancer and chemical contamination of drinking water are “impossible to summarize in a straightforward way” because they typically involve exposures to poorly defined mixtures of chemicals over unknown periods of time. Widespread chemical contamination of drinking water may be an unintentional, ongoing human experiment, but it is one that runs without the benefit of controls or experimental design.
The most out-of-control, run-amok experiment in drinking water contamination ever set in motion may be Camp Lejeune. In the early 1980s at this U.S. Marine Corps Base Camp in North Carolina, two drinking water systems were discovered contaminated with two different industrial solvents: TCE (trichloroethylene) and PCE (perchloroethylene). Other A-list contaminants also started turning up—benzene, toluene, vinyl chloride—and at levels not trivial. In some cases they were hundreds of times over their maximum contaminant levels and approached the highest concentrations ever measured in a public drinking water well. The people so exposed were not just Marines, as the base was a temporary home to many young families, including mothers who drank the water while pregnant. Contamination appears to date back to the 1950s, meaning that a million people may have been exposed over a decades-long period before Camp Lejeune became a Superfund site in 1989. Those million people, including the children conceived and raised at Camp Lejeune, are now scattered across the country, making meaningless the epidemiologist’s usual task of looking for a geographic cluster of cancers.
Federal studies were commissioned. And the results—which largely revealed nothing—have been fiercely contested by both scientists and former Marines alike. In April 2009, the Agency for Toxics Substances and Disease Registry, admitting omissions and scientific inaccuracies, took the unusual step of retracting its 1997 study that had concluded the contaminated water was not linked to health problems on the base. Meanwhile, the National Research Council’s long-awaited 2009 report concluded that it could not conclude: too many years had gone by. There was no historical information about who used the water, for how often, and for how long. There were difficulties in modeling drinking water distribution throughout the system, as the contaminated wells had cycled on and off over the years. The evidence that the solvents in question were inherently carcinogenic was conflicting. And most eyebrow-raising of all: the NRC concluded that no other future study, no matter how it was designed, could ever get to the bottom of the matter. In effect, it declared all further investigation to be a waste of time. “It cannot be determined reliably whether diseases and disorders experienced by former residents and workers at Camp Lejeune are associated with their exposure to contaminants in the water supply because of data shortcomings and methodological limitations, and these limitations cannot be overcome with additional study.”
Is this report an honest appraisal of the limits of science? Or is it an excuse to turn a blind eye? These questions are being asked both by other scientists—some of whom believe that further research is warranted and that not-yet-deployed methodologies could help unravel the mystery—and by the Marines themselves. One of them is a male survivor of breast cancer, which is an exceedingly rare disease among men. (In the United States, a man’s lifetime risk of breast cancer is one-tenth of 1 percent.) As of July 2009, he had located seventeen other former Marines, all men, with breast cancer, all of whom had been stationed at Camp Lejeune. “It was almost a relief to find out my cancer actually came from somewhere,” he said. “I’m not just some idiot who got breast cancer for no reason.” Soon after, twenty-five more male Marine veterans with breast cancer and Camp Lejeune histories came forward. Further investigations are planned.
Other studies of cancer and contaminated drinking water have dispelled the notion that cancer is a disease of no reason. None of them are perfect. Most of them are ecological in design, that is, they simply describe patterns of association between health problems and environmental problems. All together, however, they tell a consistent story. And all together, these studies may have the power to make cancer patients living in communities with contaminated water see themselves as “not just some idiot.” They certainly have had that effect on me.
As we saw in Chapter Four, bladder cancer mortality was elevated among men (but not women) living near Pennsylvania’s infamous Drake Superfund site, an old chemical dumping ground full of known bladder carcinogens. On Cape Cod, high rates of bladder cancer and leukemia were associated with living in homes serviced by vinyl-lined water pipes that were leaching perchloroethylene. Recall also from Chapter Four the nationwide study in which cancer mortality was found to be elevated in U.S. counties where drinking water was contaminated by leaking hazardous waste sites.
Similar studies have been conducted in other settings, both urban and rural. In New Jersey, researchers found associations between volatile organic compounds in municipal water and leukemia among women (but not men). In Iowa, lymphoma rates were elevated in counties where drinking water was drawn from dieldrin-contaminated rivers. In Massachusetts, childhood leukemias in the industrial town of Woburn were linked to a pair of water wells contaminated with chlorinated solvents. In North Carolina, a cancer cluster in the rural community of Bynum was linked to consumption of river water contaminated upstream with both agricultural and industrial chemicals. This study is particularly compelling because, once the normal latency period for cancer is factored in, the sudden increase in cancer deaths that emerged in the 1980s corresponds closely with the time of peak exposure to known carcinogens in the river (1947 to 1976). Likewise in Woburn, the surge in childhood leukemias coincides with a period of known water contamination and abates a few years after the imputed wells closed down. (Public outcry about the plight of Woburn’s children played a direct role in the creation of the Massachusetts Cancer Registry. It also inspired the book and film A Civil Action.)
Corroborating evidence also comes from abroad. In a study from China, liver cancer was strongly associated with drinking water from ditches containing agricultural chemicals. In Germany, excess cases of childhood leukemia in villages near uranium mines have been tentatively linked to radium-contaminated drinking water. And in Finland, high rates of non-Hodgkin lymphoma were discovered in a rural community where water was contaminated by chlorophenols, probably from local sawmills. Used for treating lumber, chlorophenols are related chemically to the phenoxy herbicides, which are also linked to non-Hodgkin lymphoma.

In a practice that began early in the twentieth century, the city of Chi - cago began in 1908 to pour chlorine into wastewater before sending it downstream. In the same year, the waterworks of Boonton, New Jersey, became the first to add chlorine to water intended for drinking. Chlorination proved a cheap, effective means of halting waterborne epidemics during World War I. By 1940, about 30 percent of community drinking water in the United States was chlorinated, and at present, about seven of every ten Americans drink chlorinated water.
Over the past three decades, dozens of studies have emerged that link chlorination of drinking water to cancer. “Mounting evidence suggests an association of bladder cancer with long-term exposure to disinfection byproducts in drinking water,” asserts Kenneth Cantor. While the data are strongest for bladder cancer, suggestive evidence also exists for cancers of the rectum, colon, and esophagus.
Upon hearing this news, many otherwise even-tempered individuals may feel tempted to throw up their arms in frustrated despair as though they had just been asked to choose between death by cancer and death by cholera. Happily, this is not our predicament. Far less gloomy options are available—as we will see below. They will not be realized, however, unless we recognize the hazards created by the approach presently used to combat disease pathogens in our drinking water and, with this knowledge, insist on safer practices.
Chlorine gas is a noxious poison. However, the problem with chlorinated drinking water does not lie with chlorine itself. Rather, the problem begins when elemental chlorine spontaneously reacts with organic (carbon-based) contaminants already present in water. Their organochlorine offspring are known as disinfection by-products. More than six hundred different water disinfection by-products have been discovered, but only a sliver of these has been tested for carcinogenicity. One disinfection by-product for which there is damning evidence is MX. MX is a complicated organochlorine whose full chemical name—3-chloro-4- (dichloromethyl)-5-hydroxy-2(5H)-furanone—is so unwieldy that it is referred to only by the science fiction-y designation Mutagen X, or, more typically, by the hip-sounding abbreviation MX. Unique to drinking water, MX was flagged as a mammary gland carcinogen in rodent bioassays. As its name suggests, it is characteristically capable of damaging chromosomes in ways that trigger genetic mutations. MX is not regulated in drinking water and not monitored routinely. Thus, asking if MX might be playing a role in the story of breast cancer among U.S. women is an unanswerable question. We have no exposure data. A 2002 nationwide survey of chlorination by-products in drinking water samples did reveal that MX was found in levels higher than previously reported, with the highest levels collected from utilities using chlorine dioxide or chlorine for primary disinfection.
In contrast to MX, trihalomethanes and haloacetic acids, two subgroups of disinfection by-products, are regulated and monitored. Chloroform is the most common and well-known member of the first group. As with any waterborne volatile compound, our route of exposure to trihalomethanes and haloacetic acids is threefold: ingestion, inhalation, and skin absorption. (Indeed, trihalomethanes appear as one of the major chemical culprits in the bathing studies already discussed.) All trihalomethanes and haloacetic acids are regulated as two groups, regardless of the individual components of the mixture. The maximum contaminant level of total trihalomethanes is 80 parts per billion. The maximum contaminant level of haloacetic acids is 60 parts per million. In the EPA’s table of drinking-water standards, along the rows labeled “Total Trihalomethanes” and “Total Haloacetic Acids” and under the column titled “Potential Health Effects” are the words risk of cancer.
Many studies all telescope into these three words. One of the most ambitious of these investigations was led by Kenneth Cantor himself more than twenty years ago. His research team personally interviewed nine thousand people living in ten different areas of the United States. Individual histories were then combined with water utility data to create a lifetime profile of drinking-water use for each respondent. In the final analysis,
bladder cancer risk increased with the amount of tap water consumed, and this increase was strongly influenced by the duration of living at residences served by chlorinated surface water. . . . There was no increase of risk with tap water consumption among persons who had lived at places served by nonchlorinated ground water for most of their lives.
These results have been subsequently corroborated.
Giving people cancer in order to ensure them a water supply safe from disease-causing microbes is not necessary. Part of the solution lies in making wider use of alternative disinfection strategies. These include granular activated charcoal (which binds with contaminants and removes them) and ozonation (which bubbles ozone gas through raw water to kill microorganisms). Both techniques have been used successfully in many U.S. and European communities. And compared to chlorine, ozone is a more effective assassin of the diarrhea-inducing, water-borne parasite Cryptosporidium.
Part of the solution is to direct research dollars toward identifying and characterizing the full suite of water disinfection by-products. Choosing the most enlightened method of purifying water depends on knowing what hazardous chemicals are being created during the process. Half of the more than six hundred disinfection by-products so far ascertained were discovered by a single team of researchers overseen by chemist Susan Richardson at the EPA’s National Exposure Research Laboratory. She and her coworkers are now hard at work looking for others; she believes there may still be hundreds of others not yet identified. More crucially, Richardson is attempting to reveal which water treatment scenarios are linked with which toxic chemicals and, in turn, which toxic chemicals are linked to cancer. Along the way, she has discovered that ozonation creates disinfection by-products of its own. Will they prove as hazardous as chlorination by-products? There are many pieces to this particular public health puzzle, and Richardson’s work is on the forefront of the effort to find and assemble them.
Part of the solution lies in directing a spirit of urgency, inventiveness, and ingenuity toward the development of altogether new approaches to the sanitizing of water. No doubt many technologies await discovery, requiring only the devotion of resources and a collaboration of creative minds to bring them into existence.
Finally, part of the answer lies in keeping carbon-based contaminants out of drinking water in the first place. This last dictum is doubly important. Less organic content means fewer trihalomethanes. Less organic content also knocks down the number of microorganisms, thereby reducing the amount of chlorine needed for disinfection. Tellingly, water from lakes, rivers, and reservoirs generates more trihalomethanes upon chlorination than does water drawn from aquifers. This is because, in general, surface water carries more organic matter than groundwater. Some of the progenitors of trihalomethanes are natural and unavoidable: decaying leaves, fallen feathers, and grains of pollen, for example. These all contribute to the total carbon load in a body of water. But many others are neither natural nor unavoidable: sewage, chemical spills, industrial discharge, soot and other fallout from air pollution, agricultural runoff, and motor oil, for instance. Drastically reducing these inputs would go a long way toward solving the problem of disinfection by-products—as well as other grosser forms of water contamination.
This part of the solution requires that water utilities and the water-consuming public become vigilant about the protection of watersheds and aquifers. Kenneth Cantor puts it this way: “Disinfection is the final barrier against transmission of waterborne pathogens, but it must not be the sole barrier. Source protection is required.”
Source protection means more than keeping swimmers out of reservoirs and erecting fences around wellheads. In some regions, this kind of protection will require new thinking about agriculture, which needs to substitute the techniques of organic farming for practices that pour soil and pesticides into river systems. In regions where cattle feedlots and hog farms periodically send lava flows of manure into watersheds, it will require new thinking about animal husbandry. In other areas, it will require new thinking about industry. Manufacturers must find safer alternatives to organic solvents and other synthetic, carbon-based chemicals that are released into water directly, fall off barges in transit, waft into the air only to rain down elsewhere, or eventually worm their way into water via landfills and dump sites. Finally, in all regions, protection of water supplies will require new thinking on the part of individual citizens, who are asked to assume the frightening cancer risks that others have decided, on their behalf, are acceptable.
Back at the waterworks, additional improvements are possible. For example, making chlorination the last step of water treatment, rather than the initial one, lowers the amount of trihalomethanes generated, especially if the water is carefully filtered through granulated charcoal first. Artificial membranes can remove a slew of contaminants, including pesticides and solvents. Water can also be aerated to allow volatile organic compounds, including trihalomethanes, to vaporize. Because they transfer contaminants from drinking water to other environmental media, I consider these kinds of solutions less beneficial than a comprehensive strategy of primary prevention. Aeration sends waterborne organic compounds into the atmosphere where we can inhale them, and filters and membranes fill with toxic chemicals, which must go somewhere. Even while providing some immediate respite from exposure through tap water, these technological shell games keep carcinogens in circulation.
In 1910, a New Jersey court examiner declared that chlorination left “no deleterious substances in the water.” He was wrong. Nevertheless, it is clear that the disinfection of drinking water with chlorine has prevented widespread contagion and death, even as it has also contributed to the burden of human cancer. I do not advocate a ban on the chlorination of drinking water. But neither do I believe we should blithely continue old disinfection practices as though our bodies and our water supplies still existed in the world of ninety years ago. In 1910, chloroform was not considered a deleterious substance. When its toxicity was later recognized, its use as a surgical anesthetic was phased out. We need not be forced to drink it now as the price for contagion-free water.

At dead center, in the channel used by barges, the Illinois River is about as deep as the deep end of a swimming pool. If you dove to the bottom here, you would first pass through a flocculent layer of silt many feet deep. Underneath this fluffy mass is the clay trough of the riverbed. If you could somehow continue the descent, drilling down through this foundation, you would eventually find yourself once again in the water—the water under the water—which is held between the glittering sand grains of the Sankoty Aquifer.
This underground basin not only lies beneath the river but stretches out for miles along its east flank and extends south toward Havana. It occupies what was once the valleys and snaky tributaries of the ancient Mississippi River—before they were bulldozed by glaciers. The Sankoty Aquifer is the source of Pekin’s drinking water.
Technically speaking, an aquifer refers not to the groundwater it holds but to the collection of grit, gravel, clay, and rock the water flows through. The Sankoty ranges from 50 to 150 feet thick and consists mostly of quartz sand grains ranging in size from dust to marbles. They are said to be distinctly pink. Sankoty sand grains are also, I’m told, neatly sorted and stratified by size, indicating they were once carried along and then deposited by the flow of a melting glacier. The resulting outwash is referred to as a valley train. Aquifers can include any porous and permeable material, such as unsorted scrap gouged up and laid back down by the glacial ice itself—this would be called till—or layers of wind-blown silt, which geologists have named loess.
Regardless of materials, aquifers come in a few basic varieties. Bed - rock aquifers are covered by a lid of impermeable materials. Artesian aquifers, often lying on a slant, are under hydrostatic pressure. Water-table aquifers are like uncovered pots with rain and melting snow periodically dribbling in through the overlying soil. Their surface, the water table proper, rises and falls with seasonal fluctuations in precipitation. The Sankoty is a very large water-table aquifer.
The chemical contamination of the Sankoty Aquifer is an ongoing story with no identifiable beginning, no defining catastrophic event, and nothing that could reasonably be called a resolution. Even before the 1993 assessment roused Pekin’s residents into action, there were signs of trouble. As part of a 1989 survey, the Illinois Environmental Protection Agency discovered “a substantial level” of 1,1,1-trichloroethane in one of Pekin’s drinking-water wells and low levels of benzene and tetrachloroethylene in another. A year later, the Illinois EPA issued two groundwater contamination advisories after accidents at loading docks sent into the public water wells of Creve Coeur a variety of gasoline additives and crude oil derivatives. Then, in the spring of 1991, high river levels contributed to the flow of chemical contaminants into a community drinking well in north Pekin.
This last discovery was particularly unsettling. The interchange between groundwater and surface water is normally a one-way affair with aquifers, recharged by rain, emptying themselves into the rivers and streams that lie across them. Barring a flood, flow of river water into groundwater is not supposed to happen. Abrupt increases in river volume or heavy pumping from wells can, however alter the direction of these unseen currents and possibly divert surface water into the underground world of aquifers. Water levels inside certain Sankoty wells, for example, appear to fluctuate in tandem with lock-and-dam operations on the Illinois River, implying a more reciprocal communion between these two bodies of water than was once presumed.
In light of this and other ominous realizations, the results of the Illinois EPA groundwater assessment actually appear quite mild. Industrial chemicals turned up in a few discrete locations, but the field team found no signs of aquiferwide contamination. Indeed, the authors of the study expressed surprise at not stumbling on an even bigger problem, especially after they reviewed the history of industrial practices within the local area: “Any contaminant that could have been produced, almost certainly was produced. And yet, we do not find widespread contamination of the ground-water environment.”
It is an eerie paradox, and it is difficult to know how alarmed or reassured to feel. Certainly there is danger in breathing sighs of relief too soon. Just as the presence of a single cockroach in the kitchen sink speaks of the hundreds more behind the wall, periodic detections of contaminants in groundwater aquifers are often harbingers of widespread contamination yet to come. Groundwater flows both leisurely—sometimes only inches per year—and smoothly as it moves along the pores and cracks of the underground landscape. Without speed or turbulence, dispersion of chemical contaminants is also slow. Over time, an intermittent detection in one well can eventually become a constant detection in several. Moreover, even the merest trace of contamination can portend a serious problem if what is being detected is the bottom edge of a falling curtain of chemicals slowly moving its way down the aquifer’s overlying substrate.
As a general rule, contamination in lowland areas of discharge—where aquifers give up their water to rivers and streams—is considered a lesser problem than contamination in the upland areas of recharge where aquifers receive rain and snow from the atmosphere. Areas of recharge are the headwaters of aquifers, and contamination here can fan out and fill the whole. In either case, once groundwater becomes contaminated, little can be done to remedy the problem. In contrast to the alfresco run of surface water, groundwater has no oxygen to hasten the breakdown of chemical contaminants nor open air to facilitate the evaporation of solvents and other volatile organics. Contamination lingers in the still, watery vaults of aquifers.
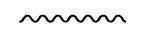
With great pomp and flair, the city of Pekin passed its proposed ground-water protection ordinance in 1995. It has since been hailed as a model for the state. Essentially, the statute regulates land use in three recharge areas, each a narrow stretch of ground a mile or so long where the groundwater underneath flows into the city’s wells. Additionally, the ordinance draws a 2,000-foot ring of protection around each of the wellheads. Inside these seven circles, the city restricts, and in some cases prohibits, the siting of new businesses that handle large quantities of hazardous materials. Existing businesses are largely grandfathered, although some have promised to make improvements.
Ever since the ordinance was drafted, personal knowledge about recharge, discharge, depth to water table, glacial deposits, and other details of hydrogeology has become a matter of civic pride in Pekin. Ground-water maps overlaid with a grid of the city’s streets have appeared on the front page of the newspaper so that residents can locate themselves vis-àvis the aquifer. The west end of Derby Street lies within a recharge area, for example, as do sections of Sabella, Charlotte, and Henrietta Streets. The east bluff does not. The owner of a gas station on Fourth Street, upon discovering himself inside one of the protected zones, pledged to install double-walled tanks to prevent leaks. He even praised the ordinance after attending a public hearing. “It’s great, it should have been done years ago, and nobody paid attention to it.”
Pekin’s ordinance is a candle in the dark. It has sparked open discussion about the relationship between health and the environment, and it has lit in people’s hearts new respect for the body of water they walk over and drink from. Its ability to safeguard the Sankoty Aquifer against the toxic activities that continue to go on ninety feet above it, however, is not at all clear. Toxic runoff from storm sewers empties into several creeks and at least one lake that overlay the recharge zone. And as the district superintendent of the water company points out, the rain itself contains pollutants. No local zoning laws can legislate against pesticide-laced raindrops or solvent-contaminated snowflakes falling in a recharge zone. Solutions to these problems need to be hammered out in chambers larger than small-town city councils.
In the meantime, industrial chemicals and pesticides persist in making cameo appearances in Pekin’s drinking-water wells—sometimes briefly exceeding their maximum contaminant levels, sometimes remaining well below the legal limit. They include benzene, perchloroethylene, 1,1,1-trichloroethane, the phthalate plasticizer DEHP, and a couple of lawn chemicals. This brings us to the present moment. We know the story of the Sankoty Aquifer begins with a glacier. We know that someone, sooner or later, ends up drinking whatever poisons are spread on the earth above it. What happens next is the part of the story that is still unwritten.
About 40 percent of Americans draw their water from aquifers. The rest drink from rivers, lakes, and streams. Of course, ecologically speaking, everyone drinks from aquifers: all running surface water was at one time groundwater, aquifers being the mothers of rivers. As Rachel Carson pointed out, contamination of groundwater is, therefore, contamination of water everywhere. And because the human body is 65 percent water by weight, contamination of water everywhere is, therefore, contamination of people everywhere.
In January 2008, the Illinois Groundwater Protection Program released its comprehensive report on the status of the state’s aquifers, based on analyses of groundwater data collected over eighteen years. The authors found “a statistically significant increasing trend of community water supply wells with volatile organic compound detections per year. More importantly, this data show an increasing trend of groundwater degradation.”
In April 2009, the U.S. Geological Survey released its comprehensive report on the status of the nation’s domestic drinking-water wells. It finds synthetic organic chemicals—nitrates, herbicides, insecticides, solvents, disinfection by-products, ingredients of gasoline, refrigerants, and fumigants—in more than half of the 2,100 wells it sampled. Concentrations of most contaminants were low but usually occurred in mixtures. The authors note that the toxicity of mixtures can be greater than that of any single contaminant, but “available human-health benchmarks do not allow full assessment of the potential health effects of contaminant mixtures because benchmarks have been established for only a few specific mixtures and are not yet available for all contaminants.”
Groundwater provides no archival photographs to consult. It offers no shores to walk along, no reflective surfaces to peer into, no fish, bivalves, grasses, or game birds to inquire about. Our relationship to aquifers is deeply biological, but it is not visual.
I once descended to the bottom of a well in order to look at groundwater in its natural habitat. This happened in Hawaii, where drinking water is drawn from a flattened lens of rain that is trapped between volcanic rock and ocean water. (Freshwater floats on salt.) At the Halawa pumping station, I rode a cable car down a 300-foot shaft to arrive in a blasted-out cavern filled with water. It was very dark and very quiet.
Illinois, I believe, would provide a more exotic underground landscape. In the descent, one could examine the mashed remains of preglacial forests, bluffs, dunes, islands, and cliffs. The bedrock floor would be inlaid with the trenches of ancestral riverbeds. The water table’s undulating ceiling would offer a subdued reflection of the overlying topography.
Cultivating an ability to imagine these vast basins beneath us is an imperative need. What is required is a kind of mental divining rod that would connect this subterranean world to the images we see every day: a kettle boiling on the stove, a sprinkler bowing over the garden, a bathtub filling up. Our drinking water should not contain the fear of cancer. The presence of carcinogens in groundwater, no matter how faint, means we have paid too high a price for accepting the unimaginative way things are.