22.1 Introduction
Teaching modern physics in secondary school requires innovative and effective approaches since its teaching has been integrated in European school curricula since few years. This approach needs to found a scientific culture linking new theories with instruments and methods typical of physics. Optical spectroscopy representing a conceptual, methodological, and historical bridge between classical and modern physics provides a fertile experimental basis of the modern atomic theory. Its disciplinary contribution to the teaching of modern physics concerns the phenomena of quantized emission and absorption of radiation which are basilar concepts representing the main investigative tool based on light–matter interaction. Optical spectroscopy, from an epistemological point of view, is a methodological and experimental context in which the role of the energy is pivotal, a validation instrument of interpretative models through indirect measures, a way through which interpret a code, hidden in the emitted light, in order to obtain information concerning states and changes of a microscopic system, as the atom, allowing to highlight the link between light emissions and atomic energy levels. From an educational point of view, competence concerning specific inquiry modalities employed in physics can be gained during physics lectures. Existing educational proposals (Luo and Gerritsen 1993; Oupseph 2007; Scheeline 2010; Amrani 2014; Onorato et al. 2015) include simple experiments allowing qualitative and quantitative measures, but those proposals have been designed and implemented in limited contexts, since students obtain the bare measurement without focusing on the emission process or the functioning of the experimental setup. Obtaining optical spectra from luminous sources is quite easy: a CD or a cheap diffraction grating are easily available objects and the produced spectra can be collected with a digital camera or a smartphone and analyzed (quantitatively or qualitatively) with specific APPs. On the other side, commercial experimental devices are often implemented in expensive and excessively structured setups that limit students’ understanding of their principles of functioning, limiting one again to the bare measure itself. The general problem is thus that laboratorial educational proposals on optical spectroscopy in secondary school are offered in the form of sterile commercial devices, leaving teacher having the task of integrating them in a coherent educational path embedding the physics of the emission process and the importance of controlling the measuring process.
The aim of Physics Education Research Unit from Udine University (IT) is to build an educational path on optical spectroscopy allowing students to be directly involved in experimental and interpretative tasks. The pivotal laboratorial activity to effectively teach and learn physics could be supported by the opportunities offered by ICT (Information and Communication Technologies). An example has been already discussed in Gervasio and Michelini (2009) and Michelini and Stefanel (2015) in the case of an educational path on single-slit optical diffraction implementing inquiries activities based on measurements performed by students themselves searching for models explaining the observed phenomena. With this in mind, our research group analyzed the most popular commercial devices and APPs performing spectroscopic measurements, in order to design a prototype for a digital spectrometer implementing some proposals based on ICT making use of a specifically designed software allowing qualitative and quantitative analysis of a digitalized spectrum. The developing of the complete low-cost technical solution will be illustrated in the following with examples of significant measures.
22.2 Some Existing Proposals
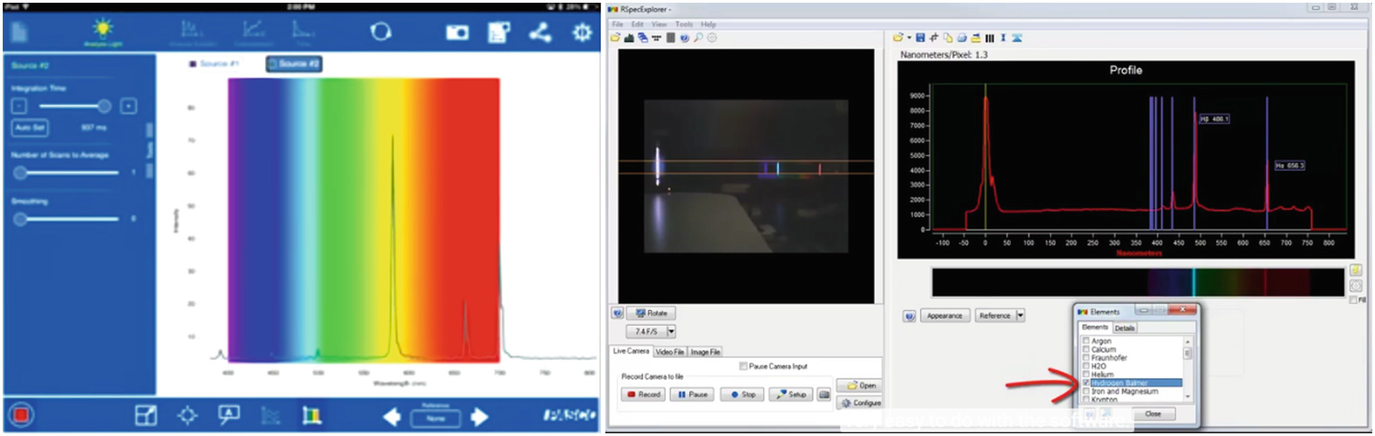
PASCO PS-2600 (left) and RSPEC EXPLORER SYSTEM (right) user interfaces
Another spectrometer, whose functioning has been studied, implementing a focusing element (a lens), rather than an optical fiber, is the RSPEC EXPLORER SYSTEM digital spectrometer.2 A diffraction grating is placed in front of the webcam lens connected via USB to the PC. When the webcam is pointed towards the light source, its spectrum, in the range between 380 and 980 nm, is registered on the CCD sensor. Users limit to select the portion of the frame where the spectrum is visible. The software digitalizes it, providing a graph in which intensity (in arbitrary units) is shown as a function of the wavelength. The quality of the measures, which resolution is about 3 nm, allows to compare the recorded spectra with reference ones. The main limit of this device is represented by the fact that no calibration phase is required and the grating could not be removed, making impossible to change the dispersive power of the instrument (i.e., the pitch of the grating) and to appreciate the effect of placing a grating in front of a light source. The user limits himself to line up the zeroth order of the diffraction pattern with a reference to automatically and uniquely associate a position along the pixel array with a specific wavelength (Fig. 22.1, right).
Both the quickly described devices are quite similar to “closed black boxes,” in the sense that, despite their high quality performance, they do not allow user to approach the physics of the measure or to change the experimental conditions. Absorption measures are automatically generated by the software as well as the calibration, that is an important phase of the measuring process.

User interfaces and logos of three APPs performing spectroscopic measurements. LIGHT SPECTRA LITE (top left), SPECTRA UPB (bottom left), and SPECTRUM ANALYZER (right)
22.3 The SPETTROGRAFO System
Our innovative device SPETTROGRAFO is a system implementing the simplicity of use of the existing commercial proposals and the feasibility of the analysis algorithms with new functionalities, missing in the analyzed devices, as the one to use grating with different pitches or to use colored filters to study selective absorption of light, or to have the possibility to dim the intensity of excessively luminous sources. Those little improvements allow the user to gain a better control of the measuring process, maintaining a good accuracy in the measured quantities using a low-cost instrument. The preliminary design of the prototype has been described in Buongiorno et al. (2018); here we report the final version of the system and relative specific technical characteristics with examples of employment in physics education.
22.3.1 The Hardware Components
Technical data of SPETTROGRAFO system
Hardware part | Data |
---|---|
Webcam | Focus: 15 cm—infinity 60 frame per second Field of view is 60° |
CCD | 640 × 480 pix 1.2 Mpix |
Case | 6 cm × 6 cm × 6 cm |
The dispersive element, a transmission diffraction grating could be placed in front of the lens of the webcam pointing towards the source whose light has to be analyzed. Light sources need to have small dimensions in order to produce spectra with enough separation between adjacent colors (we remind that a spectrum is the reproduction of the shape of the source at different angular positions with different colors). The device can be used also with extended light sources, making use of an external couple of black panels, working as a diaphragm with adjustable width.
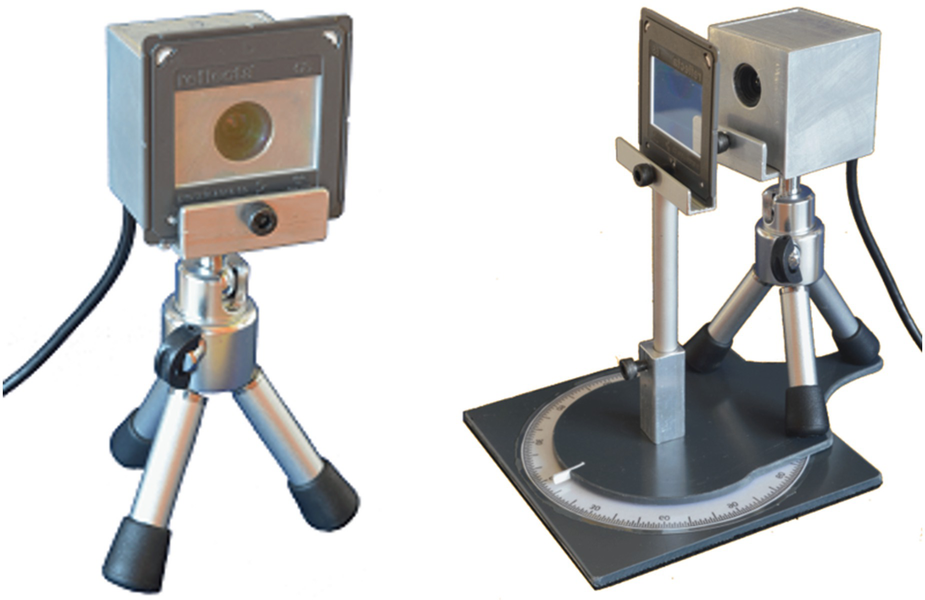
The SPETTROGRAFO system (left). The horizontal support allows to place the accessories (diffraction gratings, colored and Polaroid filters) in front of the camera lens. The system could be placed on a rotating basis (right), allowing measures in “optical goniometer mode” (see below)
22.3.2 The Software Characteristics and Peculiarities

Main user interface. Recorded image by the webcam (left) from which it is possible to select the area to be analyzed that is automatically digitalized in a graph (bottom right). In this example, the source is a hydrogen lamp observed through a 1000 lines/mm grating
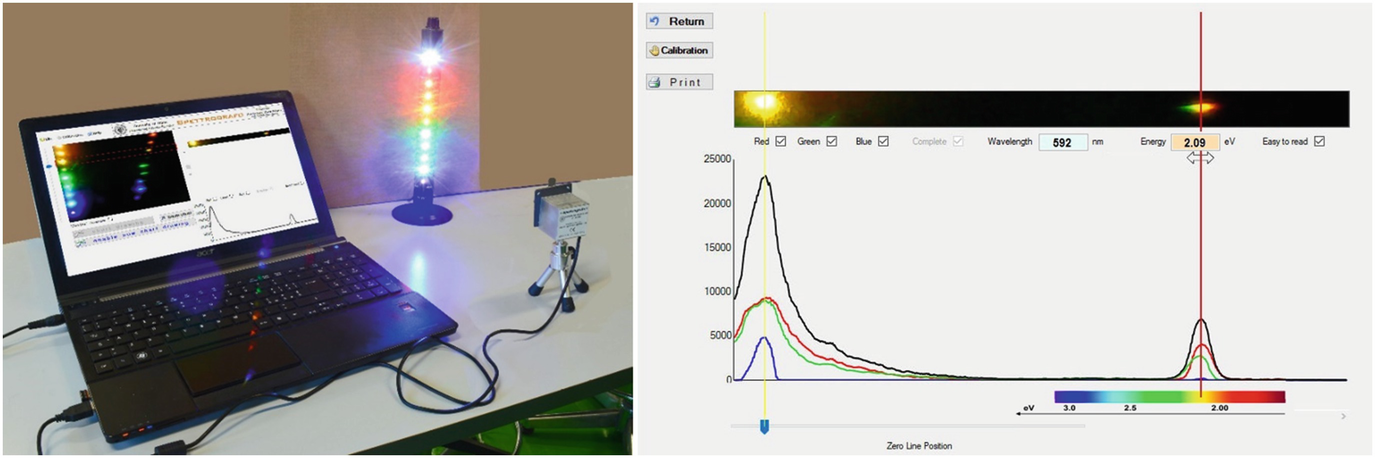
SPETTROGRAFO system connected to PC, pointing the source (left) and calibrated yellow LED spectra (right). The yellow marker on the left targets the zeroth order, while the red marker, moving along the spectrum, allows to measure the energy or wavelength of the corresponding position

Data can be exported in tabular form allowing further analysis with a spreadsheet. The advantage of describing a spectrum in terms of energy or wavelength allows different educational proposals: light could be seen as a wave or as a stream of photons with specific energies.
22.4 Examples of Significant Measures
In principle, every light source could be coupled with SPETTROGRAFO system: discrete spectra from gas-discharge or fluorescent lamps, continuous spectra from incandescent lamps, or band spectra from LEDs and absorption spectra could be detected and analyzed. To perform a measure, it is enough to point the webcam lens towards the source (Fig. 22.5, left). One advantage is that no optical bench is needed: operatively it is enough to assure the alignment between source, grating, and sensor in a way that the spectrum is horizontal with respect to the array of pixels. Preliminary tests showed that the distance between the grating and the source does not affect the precision of the measures.
22.4.1 Analysis of Discrete Emissions from Gas-Discharge Lamps in “Static-Mode”

Spectra of a cadmium (left) and helium (right) gas-discharge lamp taken with the SPETTROGRAFO system
Measure of the main emission lines of Cd and He performed with SPETTROGRAFO system compared with values taken from official database (https://www.nist.gov/pml/atomic-spectra-database)
Cadmium | Helium | ||||
---|---|---|---|---|---|
λmeas (nm) | λstd (nm) | Δ% | λmeas (nm) | λstd (nm) | Δ% |
639 | 643.85 | 0.75 | 656 | 667.82 | 1.77 |
506 | 508.58 | 0.51 | 577 | 587.56 | 1.80 |
480 | 480.00 | 0.00 | 496 | 501.57 | 1.11 |
469 | 467.81 | −0.25 | 471 | 471.31 | 0.07 |
– | – | – | 447 | 447.15 | 0.03 |
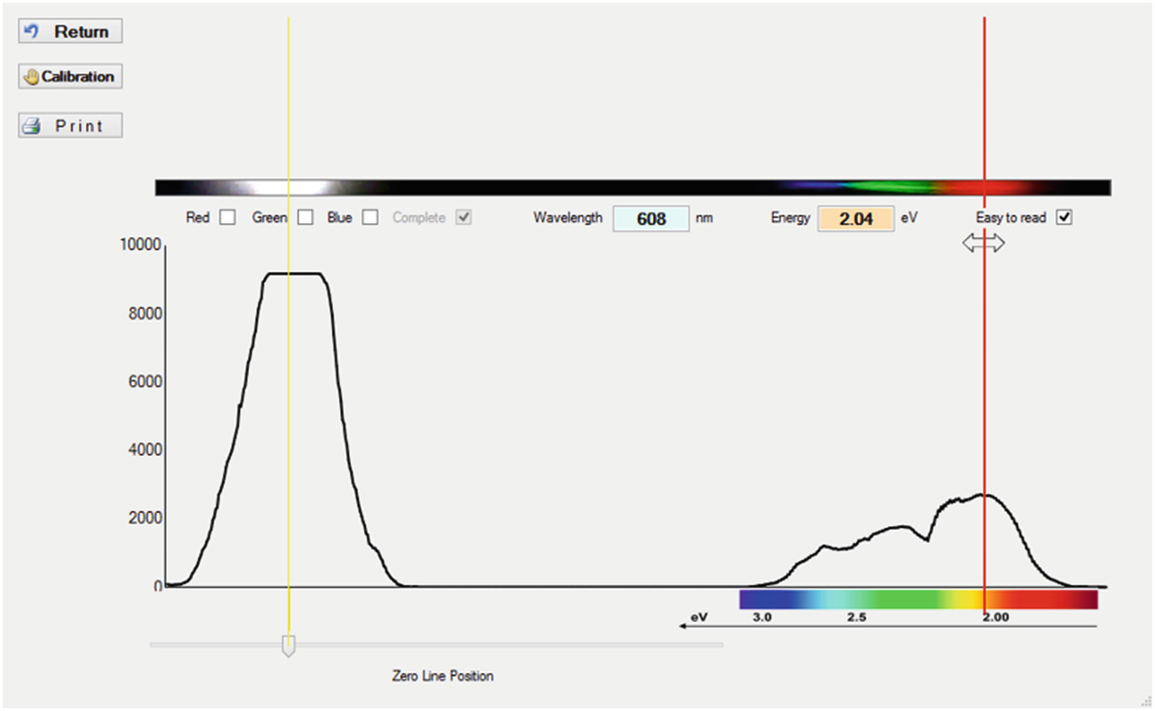
Spectra of an incandescent lamp taken with the SPETTROGRAFO system
22.4.2 Analysis of Discrete Emissions from Gas-Discharge Lamps in “Optical Goniometer Mode”
Measure of the wavelength associated to the peak emission of a blue LED
m = 1 | m = 2 | m = −1 | m = −2 | ||||
---|---|---|---|---|---|---|---|
Α | λ (nm) | Α | λ (nm) | α | λ (nm) | Α | λ (nm) |
13° | 449.9 | 27° | 454.0 | 13° | 449.9 | 27° | 454.0 |
22.4.3 Selective Absorption of Colors and Evaluation of Transmissivity Curve
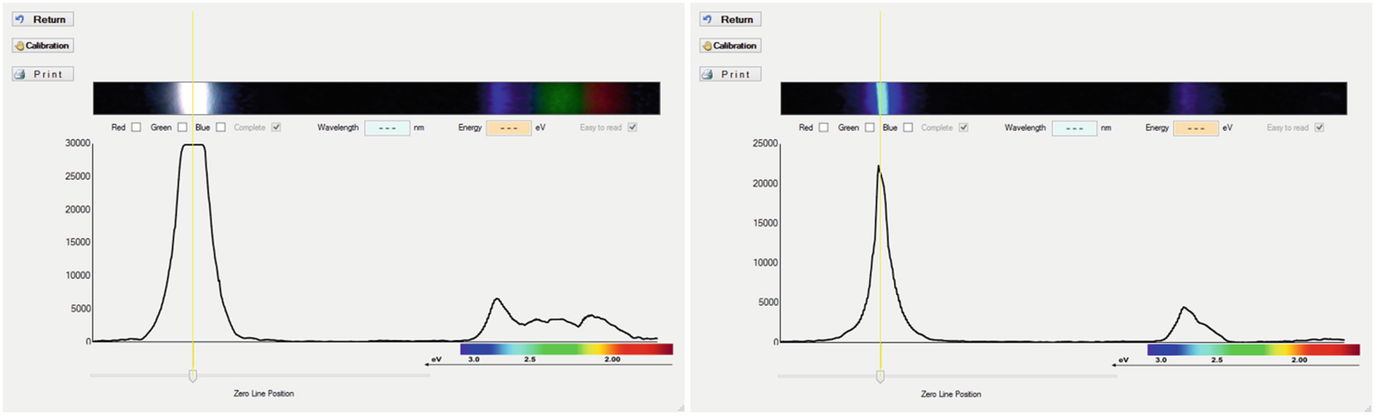
Reference spectra (left) and absorption spectrum having placed a blue filter in front of the reference source (right)
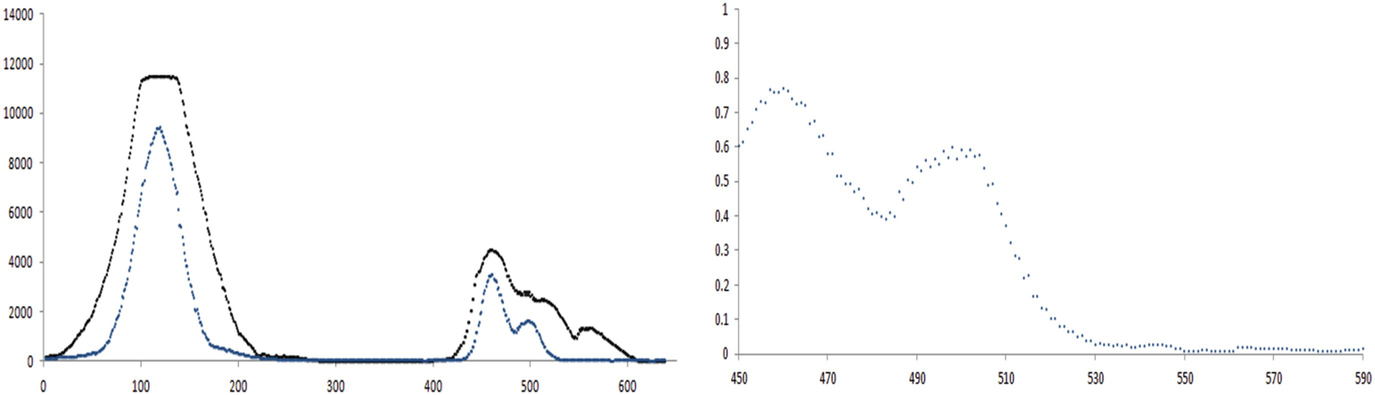
Elaboration with a spreadsheet: light spectrum from a white LED compared with the spectrum of the same light passing through a blue filter. Abscissa values refer to the column of pixel; on the left the peak due to the source is visible (left) and transmittance of the blue filter (right)
22.5 Conclusions
The main required features for an effective educational lab system for spectroscopic measurements, i.e., the importance of the calibration process, the insight into the physical processes, the possibility to change the accuracy, and the data acquisition modality emerged after having analyzed potentialities and limits of some available digital spectrometers and mobile APPs. A cheap but affordable digital device, SPETTROGRAFO, has been thus designed and realized in order to implement in a simple setup all the emerged needs. The original device allows analysis of optical spectra of different light sources and it consists of a USB webcam with the possibility to place in front of it different diffraction gratings, and colored filters to study selective absorption of colors. Virtual images of spectra are recorded on the CCD sensor of the webcam and observed with the aid of a specifically designed software. Calibration occurs via software itself by selecting the used diffraction grating or with the aid of a calibration source, and the tests showed that precision of the measures have uncertainties less than 5%. Recorded spectra are digitalized in a graph representing luminous intensity as a function of the wavelength, or energy. The device can be also mounted on a rotating base allowing to measure the diffraction angle and thus quantitatively evaluate the wavelength with hand-and-pencil calculations, as in the classical experiment of the optical goniometer.
SPETTROGRAFO system, prototype for a digital spectrometer, offers itself to be used both in secondary school educational labs and in university introductive physics courses, thanks to its inexpensiveness with respect to other commercial devices, to it easiness in use, and to the possibility to explore the functional role of every components of the measure setup. The main advantage of the system is that it is not presented to students as a mysterious “black box” working performing measurements without any awareness of the inner processes by students, rather, it allows students to develop a functional understanding of the measuring process, which is one of the main goal of an educational lab.
Up to now the device has been presented to a group of ten secondary school teachers and used by them in the context of “National School for Teachers on Modern Physics” within IDIFO6 project3 held in Udine University (IT) in September 2017, a teacher professional development activity. In the same IDIFO6 project, the device has been used in the educational lab for freshmen in biotechnology as a part of a wider didactical innovation project and it has been implemented in Masterclass and CLOE (Conceptual Lab of Operative Explorations) activity held in Udine University in the period January–March 2018 for secondary school students in the framework of a wider schools-university collaboration project.