The most convincing evidence of plant evolution is the record of fossil plants. Documented deep in the earth’s crust are the progressive changes and modifications undergone by various groups of the plant kingdom through millions of years. Every year, students of fossil plants unearth new specimens that help piece together what paleobotanists hope some day will be a continuous story of the development of the plant kingdom from an age of more than one billion years ago to the present time. During that long period of time profound changes have occurred in the plant world. Groups have arisen, flourished, and become extinct; without the fossil record present-day botanists would be unaware that such groups of plants ever existed.
THEODORE DELEVORYAS, MORPHOLOGY AND EVOLUTION OF FOSSIL PLANTS
A STERILE EARTH
We look at the amazing forests and grasslands of Earth and glorify in the “green planet” that grows so much plant material that can sustain so many different kinds of animal life. But it has not always been this way. Earth was a hostile, barren place for most of its 4.5-billion-year history. There were no land plants that could live on its harsh surface, so bare rock was exposed to intense chemical weathering, releasing all its nutrients into the ocean without any marine organisms to absorb them. The only photosynthesizing organisms for the first 1.5 billion years of life’s history were blue-green bacteria (cyanobacteria), which lived in the shallow waters of the oceans and formed stromatolites (
chapter 1). Then, about 1.8 billion years ago, we see the first evidence of algae, which are true plants with eukaryotic cells (having a discrete nucleus for their DNA, plus organelles such as chloroplasts for their photosynthesis). Both cyanobacteria and algae continued to grow huge mats of slime on the shallow seafloor.
The extremes of heat and cold, the intensity of rainstorms and runoff without the protection of plant cover, plus the absence of an ozone layer (because of the lack of free oxygen in the atmosphere) meant that few plants could venture out of the water and onto land. As long as there was no ozone layer, both plant and animal cells would be bombarded with high levels of ultraviolet radiation, which causes mutations in genes and eventually kills cells. Only the protection of being immersed in water screens most life from ultraviolet light without the protection of the ozone layer.
Based on chemical evidence, it appears that about 1.2 billion years ago the first organisms began to colonize land. They were probably very simple associations of algae and fungi called cryptogamic soils, which are very similar to the crusts of organic material found on the desert surface when it is not disturbed. The lichens that break down bare bedrock are an example of this because lichens are not an organism, but a symbiotic association of algae and fungi. The cryptogamic soils would have been the only life on Earth’s surface and would have served to help bind and stabilize the land against erosion by wind and rain, even as they helped marine algae and cyanobacteria pump more and more oxygen into the atmosphere.
Naturally, with no significant plant resources to consume on land, there was no animal life on land, either. Animal life needs not only food to eat, but also enough free oxygen in the air to breathe—which apparently did not accumulate in the atmosphere until about 530 million years ago. The combination of extreme heat and cold, lack of shelter and food, and unchecked erosion made the land a dangerous habitat that most creatures could not yet exploit.
THE FIRST LAND PLANTS
Thus the verdant planet we take for granted has not been this way for very long. For plants to begin to conquer the land, they had to be more than mats of low-growing algae, immersed in water. Algae grow well as long as they are submerged, but once they are on land, they must be kept moist or they die.
Algae must also be immersed in water to reproduce. The sperm of aquatic algae simply swim directly to the egg through the water. Green algae and many other primitive plants, for example, alternate between sexual generations (when haploid sperm and eggs are released) and asexual generations (when they clone themselves without using sex) (
figure 7.1). The diploid (with two sets of chromosomes) plant is called a
sporophyte, on which meiosis takes place to create spores within a
sporangium and results in sexual reproduction. The haploid plant (with one set of chromosomes, after having gone through meiosis) is called a
gametophyte. It generates separate sperm, eggs, or both within separate specialized structures. Alternation of generations is a common reproductive mechanism in many groups of primitive plants and animals, including most corals and anemones and sea jellies, as well as in a group of tiny shelled marine amoebas called foraminiferans.
Figure 7.1
Generalized life history of a seedless vascular plant: the adult sporophyte produces spores, which grow into a gametophyte; it, in turn produces eggs and sperm, which combine to produce another sporophyte. (From Donald R. Prothero and Robert H. Dott Jr., Evolution of the Earth, 6th ed. [New York: McGraw-Hill, 2001])
The sporophyte in primitive land plants (such as ferns) is the visible part of the plant. It releases airborne haploid spores produced by meiosis that may land in a moist spot and germinate to form a tiny (less than 1 centimeter [0.4 inch] tall) gametophyte plant. The gametophyte bears separate sperm and eggs, and the sperm can swim to the eggs only where it is moist, which restricts the options of the most primitive land plants. This “weak link” in their reproduction prevented them from exploiting drier habitats.
The possibility of desiccation, or drying out, is another challenge faced by land plants. If it is not bathed in water, the surface of a plant dries up like a stranded alga unless it is protected by some sort of waxy covering, or cuticle, to conserve the water. But the cuticle also reduces water exchange on the surface, so the plant now has more difficulty taking in carbon dioxide and releasing oxygen, as well as regulating the transpiration of water vapor. Tiny pores called stomata provide openings through the cuticle. They can be opened or closed to regulate water and gas exchange through the cuticle. However, in the process of opening their stomata, water is lost as well.
Figure 7.2
Four-part spores from the Late Ordovician of Libya, the earliest evidence of land plants. Magnification × 1500. (Photograph courtesy Jane Gray)
So what does the fossil record show about how plants invaded the land? The first fossil evidence comes from spores that came from mosses and liverworts, two low-growing plants still found in most habitats (
figure 7.2). The fossil spores are Ordovician in age (about 450 million years old), although there may also be some possible spores of Middle Cambrian age (about 520 million years old). There are some 900 genera and 25,000 living species of these most primitive land plants. They have invaded nearly every land niche, even the cool moist shorelines of Antarctica. However, they cannot live in salt water. They have many key adaptations that help them survive on land, including the ability to shut down their metabolism in adverse conditions, such as drought or extreme temperatures; the tendency to grow in clumps; the capacity to propagate vegetatively through fragments that become new plants; and the ability to colonize barren areas of exposed rock where there is little soil or to grow on the surfaces of other organisms, such as trees.
UPRIGHT PIONEERS: VASCULAR PLANTS
For plants to live on land and grow tall, they need complex organ systems to transport fluids against gravity, aid in respiration, remove wastes, and support them. A marine alga such as kelp can have strands many meters long, but because all of it is constantly bathed in seawater, it does not need a system to transport water from one end to the other.
The plants that do have such systems are known as vascular plants because they have a network of tubes to carry fluids and nutrients from one part of the plant to another—just like our own cardiovascular system carries a fluid (blood) to all parts of our body to supply them with nutrients and take away waste products. Vascular plants, however, are being “stretched on the rack.” The water and nutrients are down in the soil, but the sunlight for photosynthesis comes from above. The root end picks up nutrients and water from the soil, and moves them up to the leaves, where photosynthesis takes place (so carbon dioxide is absorbed and oxygen released), and a certain amount of water is lost.
Once plants began to grow up out of the water, they encountered two problems. First, moisture and nutrients had be transported to the higher part of the plant. Second, the plant was attempting to stand up against the force of gravity, which kept tugging it down. The solution lay in the evolution of elongate conducting cells, or
tracheids, lined with a metabolic water by-product,
lignin. Lignin is very rigid, thus lending support. It is also hydrophobic, with a surface that repels water rather than absorbing it (like waxed paper), thus speeding water through the tracheids. This conducting tissue occurs as a single central strand within the stem. In more advanced plants, tracheids can become massed to form larger woody trunks. Such vascular plants are formally known as tracheophytes, because they have tracheids inside them.
ISABEL COOKSON’S DISCOVERY
The earliest fossils of tracheophytes are tiny and not easily preserved, since the plants were made of soft organic material with no woody tissues that enhance the chances of preservation. None are known yet from the Ordovician, but by the Silurian (about 433 to 393 million years ago) there were simple plants known as
Cooksonia (
figure 7.3). Paleobotanist William Henry Lang named them in 1937 to honor the avid collector Isabel Cookson, who found the first specimens in Perton Quarry in Wales.
Cooksonia was about as simple as a vascular plant can be. Most of the specimens are crushed flat and show just a simple stem (usually less than 3 millimeter [0.12 inch] in diameter) that branches into two smaller stems. Most were no longer than 10 centimeters (4 inches) long. Many of the branched stems are topped by what, on the original compressed fossils, looked like small spheres, where the spores would form, so they are sporangia. However, better specimens and more detailed work has recently shown that the sporangia were not shaped like little round blobs, but more like a funnel or trumpet, with a conical opening in the center and a “lid” on top of the opening that disintegrated to release the spores (see
figure 7.3C).
Cooksonia had no leaves. It must have performed photosynthesis through its entire surface. It certainly had no more advanced structures like seeds and flowers. Instead of individual roots, it appears that
Cooksonia sprouted out of short horizontal connecting stems, or
rhizomes, as do many living plants that have underground runners, creating numerous clones and reproducing vegetatively. There are dark areas along these flattened, poorly preserved specimens that may be the traces of vascular tissue, although it is not well preserved enough to be certain. In addition, at least some specimens seem to have had stomata as well, further confirming that
Cooksonia photosynthesized over its entire surface, while more advanced plants focus their photosynthesis in organs like leaves or needles.
Figure 7.3
Cooksonia: (A–B) fossils; (C) reconstruction of its appearance in life, showing the funnel-shaped sporangia. ([A–B] courtesy Hans Steuer; [C] courtesy Nobumici Tamura)
At least four spore types are now associated with plants called Cooksonia, so most paleobotanists regard that genus as a “taxonomic wastebasket” for multiple lineages of very primitive plants. However, the preservation and details of the specimens are not good enough to confidently split Cooksonia into a number of genera, as taxonomy requires. Someday, however, it will be classified into multiple genera, as most other taxonomic wastebaskets are eventually.
THE GREENING OF THE PLANET
Other than paleobotanists, most people might not find such a simple tiny plant very exciting. But
Cooksonia and the origin of vascular plants represent a monumental ecological and evolutionary breakthrough. The existence of vascular land plants and green habitats on land opened the landscape for many more opportunities, especially for animals. In the Late Ordovician, we see soils with burrows that were probably made by millipedes, most likely the first land animals of all. Then from the Silurian, there are fossils of many other land arthropods, including scorpions, spiders, centipedes, and the first wingless insects. The land was no longer barren, but was beginning to develop a complex food web of plant eaters and a diversity of predatory arthropods that ate the herbivores and one another. Finally, about 100 million years after arthropods colonized the land, the first amphibians crawled out of the water as well (
chapter 10). The land was never again completely barren, but always had a green mantle of plants.
As we go through the later Silurian, there was an even greater variety of simple vascular plants. Then in the Devonian, the plants exploded in diversity, with the first forests appearing by the Late Devonian. In addition to mosses and liverworts, much more advanced plants, such as ferns, evolved. Two other important groups of living plants also appeared in the Late Silurian or the Devonian. One was the lycophytes, or “club mosses,” which creep along the ground. These living fossils are low and unimpressive, but their ancestors in the Late Paleozoic grew in gigantic forests made up mostly of “club mosses” more than 36 meters (118 feet) tall, the largest land plants the world had ever seen up to that point (
figure 7.4).
Figure 7.4
Lycophytes: (A) living Lycopodium, or “club mosses,” which today are mostly small, low-growing plants; (B) reconstruction of the 50-meter (164-foot) Lepidodendron, a lycophyte tree that grew in the swamps of the Carboniferous; the details of the trunk, bark, leaves, cones, spores, and seeds are reconstructed from isolated finds. (Courtesy Bruce Tiffney)
The other important new group was the “horsetails,” “scouring rushes,” or sphenopsids (
figure 7.5). Today, these primitive plants (one genus, a living fossil called
Equisetum) grow in great abundance in sandy and gravelly soils close to water. Their fibrous stems contain tiny particles of abrasive silica, so they are hard for animals to eat. Early pioneers called them “scouring rushes” because a crushed handful of them made a good scouring pad for pots and pans. Horsetails are very distinctive because each long hollow stem segment is covered by a series of flutings or ridges along its length and is separated from adjacent segments by a distinct joint, from which all the leaves sprout. Each horsetail stem branches from a rhizome, which sprouts many clones through vegetative reproduction.
Equisetum is a notoriously tough plant and grows rapidly in the right habitat. It quickly invades the wet parts of an entire garden if not kept in its own pot, and its underground stem is almost impossible to eliminate, so a horsetail comes back no matter what happens to it. The extinct sphenopsids of the Carboniferous included horsetails that were over 20 meters (66 feet) in height (see
figure 7.5A).
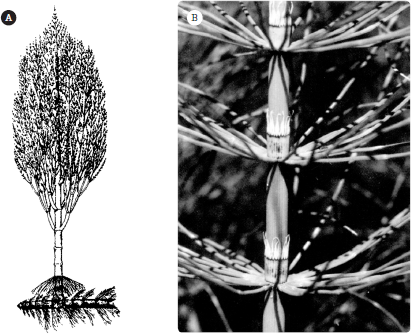
Figure 7.5
Sphenophytes: (A) the giant Carboniferous horsetail Calamites, which reached 20 meters (65 feet) in height; (B) living Equisetum, showing the leaves radiating from the joints in the stems. (Courtesy Bruce Tiffney)
In addition to all these primitive spore-bearing plants, the Late Devonian yields the first plants that reproduced with seeds, which have a hard coating that helps them germinate without being immersed in water. Some of these extinct “seed ferns” (not true ferns, but a more advanced fern-like plant that bore seeds) formed the first large trees, up to 12 meters (40 feet) in height.
The Devonian forests of “seed ferns” were succeeded in the Carboniferous (360 to 303 million years ago) by a gigantic explosion in the diversity of ferns, mosses, club mosses, horsetails, and “seed ferns.” The Carboniferous coal swamps in which they grew produced huge volumes of vegetation across large areas of the tropical areas of North America, Europe, and Asia. When these plants died and sank into the muck, they were not quickly reduced to nothing, as happens in swamps today. There were almost no animals (like termites) that had evolved to digest the hard woody tissues of lignin that made up the trees, so they just accumulated without decomposing, were compressed and subjected to high temperatures, and turned into coal.
This enormous volume of organic matter locked into the coal in the crust then transformed Earth’s atmosphere and climate. As the coal accumulated, it pulled carbon dioxide out of the atmosphere and sealed it inside the planet’s crust. Soon, the “greenhouse” climate of the Early Carboniferous (with ice-free poles, high carbon dioxide, and high sea levels that drowned most of the continents) was transformed into an “icehouse” climate by the Late Carboniferous (with ice caps on the South Pole, lower carbon dioxide, and much lower sea levels as all the polar ice pulled water out of the ocean basins). Earth remained in the grip of these “icehouse” conditions for almost 200 million years longer, until the Middle Jurassic (middle part of the Age of Dinosaurs), when it flipped back from “icehouse” to “greenhouse” due to huge changes in the mantle and in the ocean basins (
chapter 14).
The cycle from “greenhouse” to “icehouse” climate and back again has happened several times over the past billion years of the planet’s history. In fact, the existence of plants and animals is why Earth is habitable, and not a runaway “greenhouse” like Venus or a frozen “icehouse” like Mars. Earth’s living systems produce carbon reservoirs in the form of limestones (mostly by animals) and coals (by plants) that lock up carbon dioxide in the crust. This acts as a thermostat, preventing the planet from becoming a runaway “greenhouse” or a runaway “icehouse.”
Sadly, we have been unintentionally changing the planet by undoing this natural cycle. Since the beginning of the Industrial Revolution, we have burned many millions of tons of coal and released the carbon dioxide once locked in it. Now that carbon dioxide is out of control, driving our human-induced “super-greenhouse” at rates never seen in the geological past. Without knowing it, we have upset the delicate balance of carbon in Earth’s atmosphere, oceans, and crust. Our planet is already showing the extreme weather events that come from climate change, and our children and grandchildren will be paying the price for the dangerous experiment we performed when we broke the planetary thermostat.
SEE IT FOR YOURSELF!
Very few museums have displays about the earliest plants. The Field Museum of Natural History, in Chicago, has specimens of
Rhynia, a close relative of
Cooksonia, on display, as well as excellent fossils and dioramas of the coal-swamp forests. The Denver Museum of Nature and Science has an exhibition on primitive plants and a diorama of a coal swamp, as does the National Museum of Natural History, Smithsonian Institution, in Washington, D.C.
The oldest forest on Earth grew near present-day Gilboa, New York, during the Devonian (380 million years ago), and fossils of various parts of the trees are displayed at the Gilboa Museum (
http://www.gilboafossils.org), Gilboa Town Hall, and New York State Museum, Albany.
FOR FURTHER READING
Gensel, Patricia G., and Henry N. Andrews. “The Evolution of Early Land Plants.” American Scientist 75 (1987): 468–477.
Gray, Jane, and Arthur J. Boucot. “Early Vascular Land Plants: Proof and Conjecture.” Lethaia 10 (1977): 145–174.
Niklas, Karl J. The Evolutionary Biology of Plants. Chicago: University of Chicago Press, 1997.
Stewart, Wilson N., and Gar W. Rothwell. Paleobotany and the Evolution of Plants. 2nd ed. Cambridge: Cambridge University Press, 1993.
Taylor, Thomas N., and Edith L. Taylor. The Biology and Evolution of Fossil Plants. Englewood Cliffs, N.J.: Prentice-Hall, 1993.