Introduction
Scorodite (FeAsO4·2H2O) is advocated as an acceptable carrier for the immobilization of arsenic-rich wastes released during pyrometallurgical or hydrometallurgical processing of arsenic-containing ores, owing to its naturally wide occurrence in oxidized zones of arsenic-bearing ore deposits [1]. Considerable research has been carried out either looking into the experimental determination of its synthesis-production on one hand and its solubility and thermodynamic stability [2–4] on the other. From an industrial application point of view there are basically two routes available for the production of scorodite: the hydrothermal route that involves autoclave processing at elevated temperature (150–180 °C) and pressure [5, 6] and the atmospheric pressure precipitation route at around 80–95 °C first developed in our laboratory at McGill [7–9]. The McGill Atmospheric Process [10, 11] became commercial reality thanks to Ecometales in Chile back in 2012 [12]. At the same time Dowa developed in Japan an oxidation based atmospheric scorodite process variant that demonstrated at pilot plant scale [13–15]. Other companies have also been active testing different scorodite production options [16]. The stability of scorodite is, however, highly pH dependent (typically at 4≤ pH ≤ 7) and satisfactory only under oxic disposal conditions. Its stability may be inadequate under more alkaline conditions (pH = 7–8.5) while it undergoes reductive break down when Eh < 200 mV [17–19]. Therefore, there is strong interest in investigating stabilization technologies to enhance the stability of scorodite over a wider range of disposal conditions.
New stabilization approaches other than conventional cement-based ones [20] were conceived and investigated by our group based on the concept of encapsulation. Two types of encapsulation materials were examined, metal phosphates (namely, hydrated aluminum phosphate (AlPO4·1.5H2O) and hydroxyl- or fluoro-apatites (Ca5(PO4)3OH,F)) [21, 22] and aluminum hydroxyl gels [23]. Scorodite was encapsulated by direct deposition of phosphate minerals under controlled supersaturation conditions [21]. The encapsulation appears to be effective in suppressing the release of arsenic under both oxic and anoxic conditions by more than one order of magnitude. Arsenic release from the coated scorodite with hydroxyl/fluoro apatite was partially blocked as it was rather in the form of very thin layer but more importantly was associated with release of soluble phosphorus; as such the coating may eventually disappear [22]. However, the aluminum hydroxyl gel was proved to be highly effective encapsulating material compared with metal phosphates [23]. Thus for example there was only 0.2 mg/L of As released from the sulphate gel/scorodite system (Al:As = 1.0) equilibrated at pH 7.3 under oxic condition, one to two orders lower than naked scorodite.
In the present paper, we review the aluminum gel stabilization system for scorodite providing new data that prove the gels form mineralized products of aluminum hydroxyl-oxides that are protective under both oxidizing and non-oxidizing conditions. The encapsulation process involves blending atmospherically produced scorodite particles with aluminum hydroxyl gels and allowing sufficient ageing for the formation of a protective aluminum hydroxide/oxide matrix that suppresses arsenic release under wider pH and redox potential range conditions than ever reported before for scorodite.
Experimental
Synthesis of Scorodite, Aluminum Hydroxyl Gel and Encapsulation of Scorodite
The scorodite substrate material was synthesized by atmospheric precipitation via supersaturation control, which has been developed at McGill’s HydroMET Laboratory [8, 11]. In this procedure 0.5 L As(V)-Fe(III)-H2SO4 solution containing 40 g/L arsenic(V) and iron to arsenic molar ratio of one were placed in a 1-L Applikon® Bioreactor and heated to 95 °C. When the temperature inside the reactor reached ~65 °C and the pH had dropped to 0.4, 5 g of hydrothermally produced scorodite was added to the reactor as seed. In the presence of seed, precipitation started and was allowed to proceed for 24 h, after which the slurry was filtered using a pressure filter with 0.22 µm pore size membrane filter. Solids were then subjected to several washing and consecutive Toxicity Characteristic Leaching Procedure (TCLP) type leachability steps. The freshly prepared scorodite particles were subsequently used for encapsulation with aluminum hydroxyl gels.
Aluminum(III) hydroxyl gels were produced by partial (molar ratio OH:Al = 2.5) quick neutralization of 2 mol/L Al(SO4)1.5 solutions with 5.0 N sodium hydroxide (NaOH) at room temperature as described in our previous paper [24]. Mild stirring had to be applied during mixing as excessive force was found to be counterproductive causing gel thinning. The freshly prepared aluminum hydroxyl gels were used to encapsulate scorodite particles.
The encapsulated scorodite was achieved by blending scorodite and aluminum hydroxyl gels together and subsequently aged at room temperature for 7 days. A low gel/scorodite ratio (Al:As = 0.1) was applied for blending the two products. Some samples of encapsulated scorodite were subjected to SEM characterization and stability testing. A single washing step was employed prior to stability testing in order to remove any entrained soluble material from the aged samples.
Stability Test
The scorodite particles encapsulated by aluminum hydroxyl gels, as well as the scorodite substrate material, were subjected to oxic and anoxic stability testing with an orbital shaker at a liquid to solid ratio (L/S) of 20.
The oxic stability was evaluated by equilibrating these solids in de-ionized water that was initially adjusted to pH = 9 ± 0.2 with 0.5 mol/L Ca(OH)2 slurry. The anoxic stability testing was conducted at controlled reducing potential conditions (Eh = 200 ± 20 mV) via addition of sodium sulfite (Na2SO3) solution. The pH of the solution was monitored and periodically adjusted to pH 9 ± 0.2 with 0.5 mol/L Ca(OH)2 slurry. The system pH was allowed to drift to pH 7 or lower and subsequently readjusted. Both of oxic and anoxic stability test lasted 167 days.
Analysis and Characterization
Arsenic concentration in aqueous samples was analyzed using a Thermo Jarrell Ash IRIS Inductively Coupled Plasma-Atomic Emission Spectrometer (ICP-AES). Powders were characterized by X-ray powder diffraction using a Bruker D8 Discovery X-ray diffractometer with Cu Kα radiation (λ = 1.5405 Å). Field emission scanning electron microscope (FE-SEM) images were taken on a Hitachi S-4700 electron microscope equipped with an energy dispersive spectrometer for X-ray evaluation. Elemental mapping of particle cross sections was achieved by a Hitachi S-3000 N variable pressure scanning electron microscope (VP-SEM).
Results and Discussion
Synthesis of Scorodite, Aluminum Hydroxyl Gel, and Aluminum Gel/Scorodite Mixture

Typical appearance of a scorodite substrate material, b aluminum hydroxyl gel, c blend of scorodite and aluminum hydroxyl gel, and d encapsulated scorodite after ageing for 7 days

SEM images of a naked scorodite substrate material, b dried aluminum hydroxyl gel, and c blend of scorodite and aluminum hydroxyl gel (Al:As = 1.5, aged for 28 days)
Characterization of Aluminum Gel Coating

Back-scattered electron (BSE) image of scorodite encapsulated with aluminum hydroxyl gel (Al:As 0.1) and elemental X-ray maps (3000x, 25 keV)
Stability Evaluation

Comparison of near-equilibrium (167 days at 22 °C) arsenic concentrations for different systems under oxic and anoxic conditions
Post Stability Testing Characterization
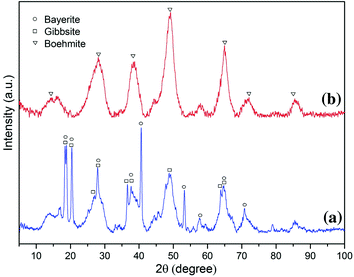
XRD patterns of NaOH derived-aluminum hydroxyl gel after 167 days of stability testing under a oxic condition and b chemically generated anoxic condition
Process Flow Diagram for Industrial Application

Conceptual process flow diagram for scorodite stabilization with aluminum hydroxyl gel
Conclusions
Aluminum hydroxyl gels were proposed to extend the stability of scorodite under wider pH and redox potential range conditions. Aluminum hydroxyl gels were blended with atmospherically produced scorodite at a low gel/scorodite ratio (Al:As = 0.1). After sufficient ageing under ambient conditions, a very thin Al-gel coating was present on the surface of scorodite particles along with the agglomerate depositions. The stability testing revealed that the encapsulation of scorodite particles with aluminum gels appears to be effective in suppressing the release of arsenic under wider pH and redox potential range conditions. Under anoxic condition, only 4.2 mg/L of arsenic released from the aluminum hydroxyl gel/scorodite system equilibrated at pH = 8.3, Eh = 210 mV that is 33 times lower than the solubility of unprotected scorodite. After being exposed to 167 days of stability testing under oxic and anoxic conditions, the aluminum gel was shown to form mineralized Al(OH)3/AlOOH phases that provide robust protection to scorodite. The results of the present work show aluminum hydroxyl gels to be a promising option in stabilizing iron arsenate solids under wider pH and redox potential range conditions and deserve further development for large-scale and industrial application.
Acknowledgements
The support of NSERC and industrial partners is gratefully acknowledged. Similarly, the contributions of several past members of our group in this work is proudly acknowledged.