Introduction
An access to high-MgO raw materials for nickel smelting directed Outokumpu in the late 1980s to development of a new primary smelting technology for nickel matte , based on the flash smelting concept. The fundamental novel idea was the expansion of slag volume generated in the smelting step, by oxidation the feed mixture to much lower iron concentrations in the produced matte than the conventional practice [1]. This involved a new flow sheet, closing internal circulation of the slag and matte between the converting and slag cleaning thus improving the metal value recoveries, in particular that of cobalt , and lowering environmental impact of the nickel matte smelting . The elimination of converters and the entire converting step had several side effects to the industrial operation, including e.g. lower fugitive emissions and smaller CAPEX [2]. It also required modifications in the refinery flow sheet [3]. The current operational practices will be described elsewhere in this Conference [4].
Fundamentals of nickel smelting are much less scrutinised than those of copper smelting [2–5]. In 1995 only limited information existed, about the fundamentals of matte-slag equilibria , when the direct nickel matte smelting was taken into industrial use, and the data on properties of trace elements at low iron concentrations in the matte below 15 wt% Fe were non-existent. In high-iron mattes, the previous focus had been in nickel losses to slag and recoveries of selected trace elements, typically cobalt [6].
The knowledge in early ‘90 s upon nickel mattes in the converting was largely based on a review of Kellogg [7] and the prior experimental data. Font et al. [8] and Henao et al. [9] presented new experimental data on the slag -matte-gas equilibria in MgO crucibles and about selected trace elements. The scope was in conventional matte making and its conditions. Certain details on trace elements in nickel matte converting related to platinum group element distributions were studied [10]. For helping to understand the coupled phenomena in converting, process dynamics modelling has also been used [11, 12].
This presentation gives an overview on the recent studies of the slag -matte equilibria carried out with a novel experimental technique, allowing accurate observations about phase equilibria , their assays and the distributions of minority elements deporting them between matte to be recovered and slag where many elements will be discarded at low concentrations to various slag products.
Experimental
The experimental technique was based on gas equilibration of small matte-slag samples on a solid substrate in flowing CO -CO 2-SO2-Ar mixtures of controlled compositions. The experimental conditions were selected so that sulphur dioxide pressure in the furnace in all conditions was P(SO2) = 0.1 atm. This was a modification of a technique used earlier in many geochemical applications [13], and adopted by Jak et al. [14] for metallurgical slags and slag -metal equilibria . The experimental set up as well as the techniques used for confirming the state of equilibrium reached in the experiments of this work have been presented earlier in detail in the literature [15–17].
The experimental breakthrough in the analytical techniques for the trace elements was the use of laser ablation-inductively coupled plasma-mass spectrometry (LA-ICP-MS) directly from the polished sections, without separating the different phases of samples prior to the phase composition analyses. Combined with the electron microprobe X-ray analysis (EPMA) it allowed accurate chemical analyses in the whole range from several wt% to sub-ppm concentrations. The technique used also involved a statistical evaluation of the composition of each phase so that 8–10 points were measured on well-quenched domains of the sample and in addition to the average composition, its standard deviation will also be reported [15]. Those were the first metallurgical slag samples analysed with LA-ICP-MS technique and a lot of effort was put on the possible systematic errors arising from samples, from different composition domains than the geological specimens studied earlier [18].

A comparison of EPMA and LA-ICP-MS data of cobalt concentration in iron silicate slags at nickel matte saturation containing about 0.5 wt% Co in different iron concentrations of the sulphide matte
![$$ \left[ {\text{FeS}} \right]_{\text{matte}} + 1/2{\text{O}}_{2} \left( {\text{g}} \right) = \left( {\text{FeO}} \right)_{\text{slag}} + {\text{ 1/2S}}_{2} \left( {\text{g}} \right). $$](../images/468727_1_En_25_Chapter/468727_1_En_25_Chapter_TeX_Equ1.png)

Results

Experimental slag composition of silica saturation at 1400°C superimposed on a calculated isothermal section of the system by MTDATA and Mtox database, vers. 8.2 [19]; CPX = clinopyroxene, HAL = halite (wüstite, MgO), OLI = olivine, OX_LIQ = slag , TRI = tridymite

Liquidus contour projection as a function of SiO2 concentration with MgO as parameter: silica solubility increases along with MgO-concentration; the calculated boundaries at MgO = 0 (–) and 9 wt% (―) are based on Mtox database (vers. 8.2) and MTDATA software

Sulphur solubility in DON slags at iron concentrations of the matte from 3 to 12 wt%; the slags with a similar MgO-level at 1400°C have been plotted on the same graph and no impact of the [Ni]:[Cu] ratio of nickel matte can be seen (open symbols [Ni]:[Cu] = 2, closed [Ni]:[Cu] = 4)
![$$ {\text{L}}^{{{\text{m}}/{\text{s}}}} \left( {\text{Me}} \right) \equiv \left[ {{\text{wt}} - \% {\text{Me}}} \right]_{\text{matte}} /\left( {{\text{wt}} - \% {\text{Me}}} \right)_{\text{slag}} $$](../images/468727_1_En_25_Chapter/468727_1_En_25_Chapter_TeX_Equ3.png)
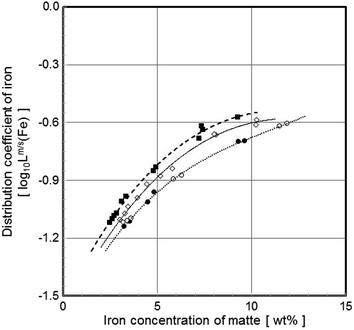
Distribution coefficient of iron between matte and slag as a function of iron concentration at 1400°C with MgO-concentration of the slag as parameter (open symbols [Ni]:[Cu] = 4, closed 2; ⃞ 8–9%, ⃟ 4 and ⃝ 0% MgO)

Distribution coefficient of nickel and copper between nickel matte and slag at 1400°C with different MgO concentrations in the slag (11–12 wt%, 6–7 wt% and 0 wt%: ●, ● and ○; [Ni]:[Cu] = 2)

The effect of [Ni]:[Cu]-ratio of matte on the distribution coefficient of nickel at (MgO) = 0 (a) and 8 wt% (b) at 1400°C, as a function of iron concentration of the matte (open symbols [Ni]:[Cu] = 4, closed 2)
MgO additions to the slag favour the distribution of copper and nickel to the sulphide matte. The effect is small but clear in all studied concentrations of magnesia . The distribution coefficient is affected slightly also by the used boundary condition of this study, when (Fe):(SiO2) ratio of the slag decreases with increasing MgO, as pointed out earlier, e.g., by Takeda [21] and Strengell et al. [16].
As suggested earlier (e.g., Teague et al. [6]) the reason of more favourable distributions between matte/metal and slag is the increase of the activity coefficient of less basic oxides, by formation of stronger MgO-SiO2 bonds in the slag when magnesia is added.
The distribution coefficients of the trace elements cobalt and gold between the nickel -copper mattes as a function of its iron concentration and slag are shown in Figs. 9 and 10, respectively. The nickel -to-copper ratio of nickel sulphide matte in these studies was [Ni]:[Cu] = 4 (w/w). As can be concluded from Fig. 10, the solubility of palladium from the nickel mattes containing 1 wt% Pd in the iron silicate slag is very low (< 1 ppm).

The effect of MgO concentration in slag on the distribution of cobalt between nickel matte and iron silicate slag at 1400 °C in silica saturation; (wt% MgO) = 0, 4 and 8 and [Ni]:[Cu] = 4
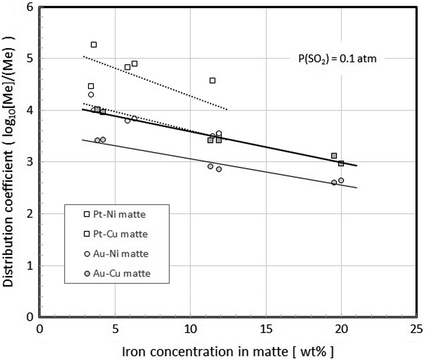
A comparison of matte-slag distribution coefficients for gold and platinum in copper and nickel matte -slag systems at 1350 °C and 1400 °C, respectively; [Ni]:[Cu] = 4 in the studied nickel sulphide matte

The effect of iron concentration in nickel sulphide matte and MgO concentration in the iron silicate slag on the distribution coefficient of gold at 1400 °C in silica saturation; [Ni]:[Cu] = 4, P(SO2) = 0.1 atm
Conclusions
Due to the absence of data on nickel -copper -iron mattes in low iron concentrations, below 15 wt% [Fe] and in the operational window of DON process, an experimental program was carried out for measuring base metal and trace element distributions. The variables used were iron concentration of the matte, its nickel -to-copper ratio, magnesia concentration of the slag and temperature . Magnesia of the slag in these conditions, in a fixed atmosphere, has a clear impact to iron concentration of matte, as a feedback from iron activity of the slag [17].
The common substance in nickel sulphide concentrates’ gangue, MgO [24, 25], improves favourably recoveries of the base metals to the sulphide matte. A particularly large impact of MgO was found to be on those elements, which are weak oxide formers, as typically the precious and some platinum group metals .
A comparison of the current observations at silica saturation with the computational phase diagram Fe-O-MgO-SiO2 based on Mtox database [19] indicates a good agreement. This suggests that the assessed data of Mtox database reproduces reliable phase property data for the industrial nickel matte smelting slags in DON smelting conditions in the flash smelting furnace (FSF) [26].
Font et al. [8] presented distribution data for selected trace elements in MgO crucibles at 1300 °C (i.e. in olivine saturation). They compared their observations as a function of P(SO2), with mattes of different [Ni]:[Cu] ratios and iron concentrations, including the limiting ‘binary’ matte systems Cu2S-FeS and Ni3S2-FeS. Their results indicate the effects of prevailing P(SO2) on the dissolution of the base metals into iron silicate slags. The impact of MgO on the distributions is not visible, due to olivine saturation where silica concentration of the slag still is a free variable, as indicated in Fig. 1, and was less accurately controlled in those experiments.
![$$ \left[ {\text{S}} \right]_{\text{matte}} + {\text{O}}_{2} \left( {\text{g}} \right) = {\text{SO}}_{2} \left( {\text{g}} \right), $$](../images/468727_1_En_25_Chapter/468727_1_En_25_Chapter_TeX_Equ4.png)
The results by Font et al. [8] imply that the matte-slag distribution coefficients for As and Sb increase when iron concentration of copper -nickel matte decrease, and the highest values for As, Bi and Sb were always obtained in copper -free mattes. This pattern, based on the present experimental observations, is more complicated if the effect of magnesia on the slag assay and its silica will be taken into account. There seems to be no data concerning the non-saturated magnesia -bearing iron silicates.
Acknowledgements
The authors are indebted to Boliden Harjavalta Oy for its dedication and SIMP program by Tekes and Dimecc Oy for funding this extensive study.