Introduction
Direct reduction of oxide Metallic sulfides are commonly distributed as natural minerals and used as important resources for metal production (such as Zn and Cu). However, some elements do not form the stable sulfides but exist as the oxides in nature. For example, Ti, V, Nb and Ta have their stable oxides as the ore of metal production. These oxides are reduced by Al or Mg not by carbon because of their strong thermodynamical stability. In order to get highly pure metals (for example, <0.3 mass%O in Ti), their oxides are once converted to chloride and repeatedly refined by distillation to eliminate their impurities. The pure chlorides are then reduced by Mg to obtain the metallic state. The by-product MgCl2 after the Mg reduction is electrochemically decomposed in a MgCl2 melt to recycle to the reactant Mg. In the refining industries of the oxide-stable elements, the molten salt electrolysis of chloride is indispensable and the efficient electrolysis has been developed, for example, in Kroll process [1] for Ti production.
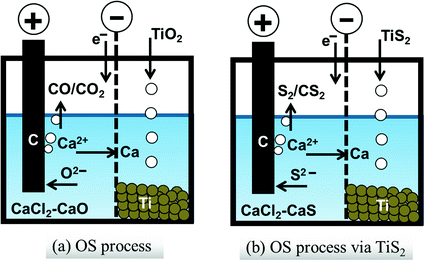
Concepts of a oxide reduction by molten salt electrolysis in CaCl2 (OS process) and b sulfide reduction to obtain the metallic Ti particles. Oxygen and sulfur anion react with carbon anode to form a CO/CO2 and b S2/CS2, respectively
Merit of reduction of sulfide In contrast, the solubilities of sulfur in metallic Ti, V and Nb are very small [10], and the serious effects of residual sulfur was not known in pure Ti. Sulfur locates just below oxygen in the periodic table, and many similarities may be found in thermochemical properties. If the reduction of oxide can be replaced by the reduction of sulfide , a higher quality of metal production can be expected from the sulfide . The thermochemical stability of oxides does not allow the reduction of hydrogen and carbon, and the strong reductant such as Ca is required. Therefore, the electrochemical technology of direct oxide reduction [5–7] can be applied to the reduction of sulfides, as shown in Fig. 1b.







Concept of oxide sulfidation by CS2 gas and sulfide reduction to obtain the metal. The exhausted CS2 gas in the second step can be used in the first step
Purpose The purpose of this work is to show the applicability of our proposal consisting of two steps: sulfidation of TiO2 and reduction of TiS2. The conversion of TiO2 to TiS2 was shortly confirmed here. The successive reduction of TiS2 to Ti was mainly tested by two methods, either by calciothermic reduction or electrochemical reaction in the CaCl2 melt. The details were separately reported in [17, 18].
Experimental

Experimental setup for calciothermic reduction in stainless steel crucible (a), and electrochemical reduction in dense MgO crucible (b), using CaCl2 melt in Ar gas
Constant voltage of 3.0 V was applied between the two electrodes at 1173 K, and terminated after a certain amount of electricity was supplied. The solidified salt was removed by wet-chemical way and dried in vacuum. For sulfuration, about 1.5 g of TiO2 (rutile) powder was filled in Al2O3 boat, which was heated at 1073 K in Ar. The feeding Ar gas was firstly blown into the CS2 liquid kept at 273 K. After optimizing Ar gas flow rate, the gas mixture with a constant concentration of CS2 was fed to the furnace . After heating for a few hours, the sample was cooled in Ar. The sulfur and oxygen content were determined using LECO-CS600 and TC600 analyzers, and the phases and structure were characterized by X-ray diffraction (XRD) measurements and scanning electron microscopy (SEM ).
Results and Discussion
Calciothermic Reduction

Sulfur and oxygen concentration after Ca reduction using CaCl2 melt. The analytical error was within the size of makers. Molar ratio of Ca against TiS2, r, was taken to normalize the deviation of charge. r = 2 means that the stoichiometric amount of Ca reacts with the charged amount of TiS2. Phase identification by XRD was inserted

Electrochemical Reduction

Sulfur and oxygen concentration after electrochemical reduction applying 3.0 V at 1173 K using 0.5 mol%CaS–CaCl2 melt [17]. Supplied charge Q is normalized by the stoichiometric amount of charge Q0 for reduction. Q/Q0 = 1 means that the stoichiometric amount of charge to reduce the charged amount of TiS2
When CaS was added, the current during electrolysis increased and the electrolysis time necessary for TiS2 reduction became shorter [17, 18]. For example, this time can decrease to a quarter of that at 0.5 mol% CaS. However, the residual sulfur concentration in the samples also increased, and the concentration of oxygen remained as high as 1 mass%O.

SEM image of the samples before and after electrolysis of TiS2
Sulfuration of TiO2
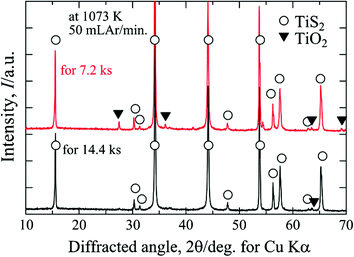
XRD patterns of the samples converted from TiO2 powder. The power on alumina boat was exposed under constant flow of CS2 and Ar gas mixture at 1073 K for 7.2 and 14.4 ks. The black specimens were taken from the upper part of powder at the central part of the boat

SEM image of the sample converted from TiO2 powder. The power on alumina boat was exposed under constant flow of CS2 and Ar gas mixture at 1073 K for 14.4 ks. A crystal growth with hexagonal symmetry is a characteristic for TiS2 crystal
Thus the optimal conditions for sulfidation still remain as future’s work, but the new concept of sulfide usage was well established from our experimental evidences. The pursuit of higher quality titanium via sulfide will be studied soon.
Conclusion
The commercial TiO2 with rutile structure was favorably sulfidated to TiS2 at 1073 K by CS2 gas carried by Ar. In addition, we examined the reduction of TiS2 both by metallic calcium in molten CaCl2 and by electrolysis in CaCl2–CaS molten salt . The residual concentrations of sulfur and oxygen in the obtained samples were analyzed, and the phase constitutions were identified by XRD . With the use of calciothermic reduction , a single phase of metallic titanium was obtained with 0.03 mass%S. With the use of electrolysis, a very low impurity such as 0.01 mass%S was obtained when the supplied charge was Q = 4 Q0. A single phase of metallic titanium was obtained when the supplied charge Q was greater than 2 Q0. The concentration of oxygen decreased to 0.5 mass%O with calciothermic reduction and to 1.0 mass%O with electrolysis. The further addition of CaS in the melt can decrease the electrolysis time for reduction .
We believe that our experimental results significantly contribute to improve the proposal to obtain titanium via TiS2.
Acknowledgements
This work was partly supported by the “Innovative Structural Materials Project (ISMA, Future Pioneering Projects)” and KOBE STEEL, LTD. under contact No. 14100139-b, and by JSPS KAKENHI (Grant Number 17H0343407).