7.1 Introduction
The accident at the Fukushima Daiichi nuclear power plant (FDNPP) in March 2011 released a significant amount of radiocesium (Cs), which caused serious and long-term radioactive contamination of the land around the power plant. It is essential to understand the state and dynamics of radiocesium in the environment to consider its influence on life, agriculture, the decontamination processes, etc. in Fukushima at present and in the future. More than 6 years after the accident, our knowledge of radiation and radiocesium in Fukushima has been increased owing to the work of a number of research groups in Japan. For instance, measurements of the depth profile of radiation in the soil revealed that most radiocesium remains at a shallow depth and it hardly moves downward with time (e.g., Honda et al. 2015), which suggests that radiocesium is being trapped rigidly in specific materials such as clay minerals in the soil. Many researchers have suggested, mainly based on laboratory experiments, that micaceous minerals such as illite and vermiculite are important for the sorption and retention of Cs in the soil (Comans et al. 1991; Cornell 1993; Evans et al. 1983; Francis and Brinkley 1976; Poinssot et al. 1999; Zachara et al. 2002). For instance, Cornell (1993) summarized the potential adsorption sites for Cs in the micaceous minerals as follows: (1) cation exchange sites on the surface, (2) layer edge sites, (3) frayed edge sites (FES), and (4) internal interlayer sites. Among them, it has been suggested that FES, which are formed around the edges of platy micaceous crystals by weathering, strongly and selectively adsorb Cs (Brouwer et al. 1983; McKinley et al. 2004; Nakao et al. 2008). However, it was not certain whether such micaceous minerals really retain radiocesium in the soil around Fukushima. By analyzing the contaminated soils in Fukushima, we first reported that WB or partially vermiculitized biotite, originating from granitic rocks which constitute the geology of this area, is a dominant sorbent of radiocesium (Mukai et al. 2014). Next, we demonstrated by laboratory experiments that WB sorbed Cs more efficiently than other clay minerals from solutions if the Cs concentration in the mineral was dilute, as in the actual soil in Fukushima (Mukai et al. 2016a).
This paper reviews our recent research with respect to WB in Fukushima, including its structure and Cs-sorption/desorption properties, and discusses the role of WB in the radioactive contamination in Fukushima. Details of the experiments to obtain the results presented here are described in the original papers already published (Mukai et al. 2014, 2016a, b; Kikuchi et al. 2015; Motai et al. 2016).
7.2 Speciation of the Radioactive Particles in the Soil of Fukushima
Although the air dose rate in the areas around FDNPP is quite high due to radiocesium in the soil, the actual concentration of radiocesium in the soil is generally too low to specify its location, even if using recent advanced micro-analytical techniques such as X-ray microanalysis with synchrotron radiation. At present, only autoradiography, which is capable of detecting radiation with a certain (but not enough) spatial resolution, is a practical method for finding radioactive particles in the soil. Autoradiography using imaging plates (IPs), which are reusable and detect radioactive rays efficiently and proportionally to the intensity of radiation, has been applied frequently to find the distribution of radiation or radiocesium in various samples including soils, plant tissues, feathers of birds, etc. (e.g., Nakanishi 2016). IP autoradiography of the samples from Fukushima often showed an inhomogeneous distribution of radiation in the samples, represented by a number of “bright spots” in the IP images obtained by placing the IPs in contact with the samples for a certain time. However, results or reports that identified the materials forming the bright spots were few.

(a) Readout image from an imaging plate (IP, Fuji Film FDL-UR-V) with a grid pattern formed using a laser marker, and with radioactive soil particles dispersed on the IP and exposed for around 1 week in a dark box. (b) Magnified readout image including a “bright spot” with the image of the grid pattern. (c) The position of the IP corresponding to the image in (b). (d) The soil particle corresponding to the bright spot in (b). Probably the particle is weathered biotite, considering its platy morphology. (Mukai et al. 2014)
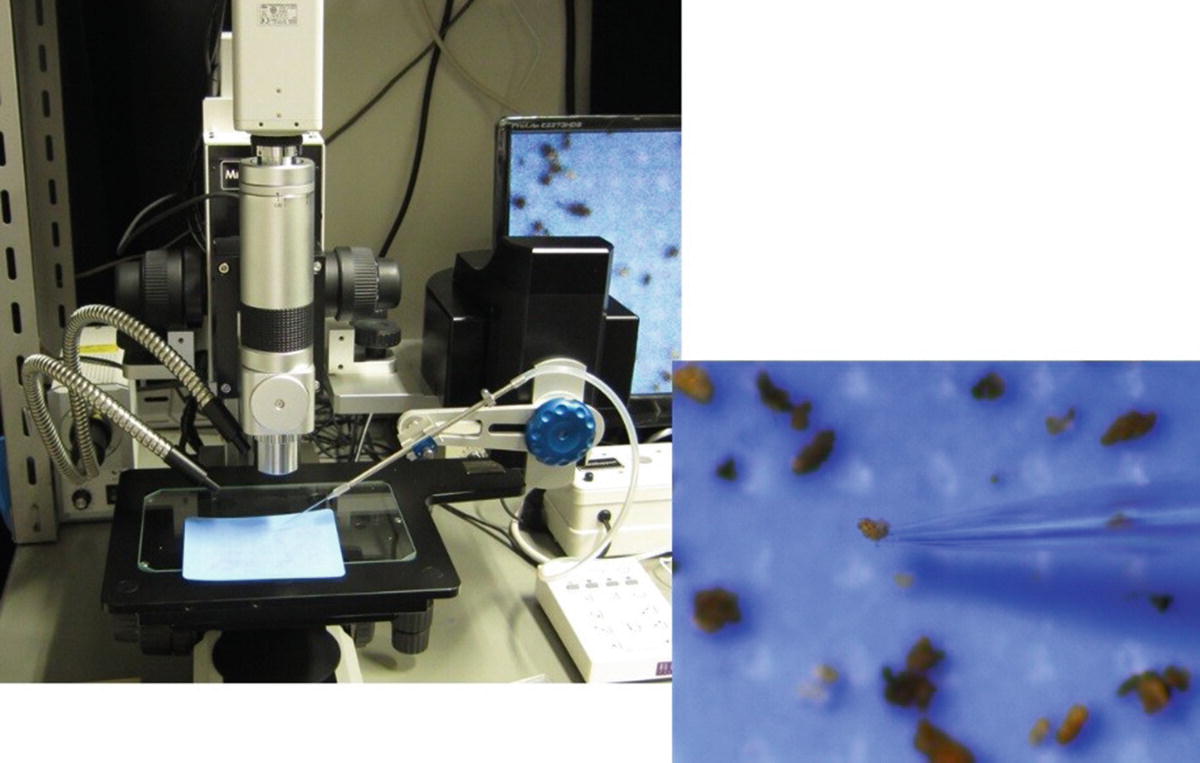
(Left) High-magnification stereomicroscope with a micro-manipulator, used to pick up the soil particles dispersed on IPs. Vacuum tweezers (an evacuated capillary tube) are attached to the micro-manipulator. (Right) A soil particle picked up by the capillary tube

SEM images (upper) and EDS spectra (lower) from the whole particle for typical radioactive soil particles found by IP autoradiography. (a) Aggregate of fine mineral particulates. (b) Particle consisting of organic matter and a small amount of minerals. (c) Weathered biotite with a platy shape. (Mukai et al. 2014)
7.3 Mineralogical Characterization of Weathered Biotite (WB)
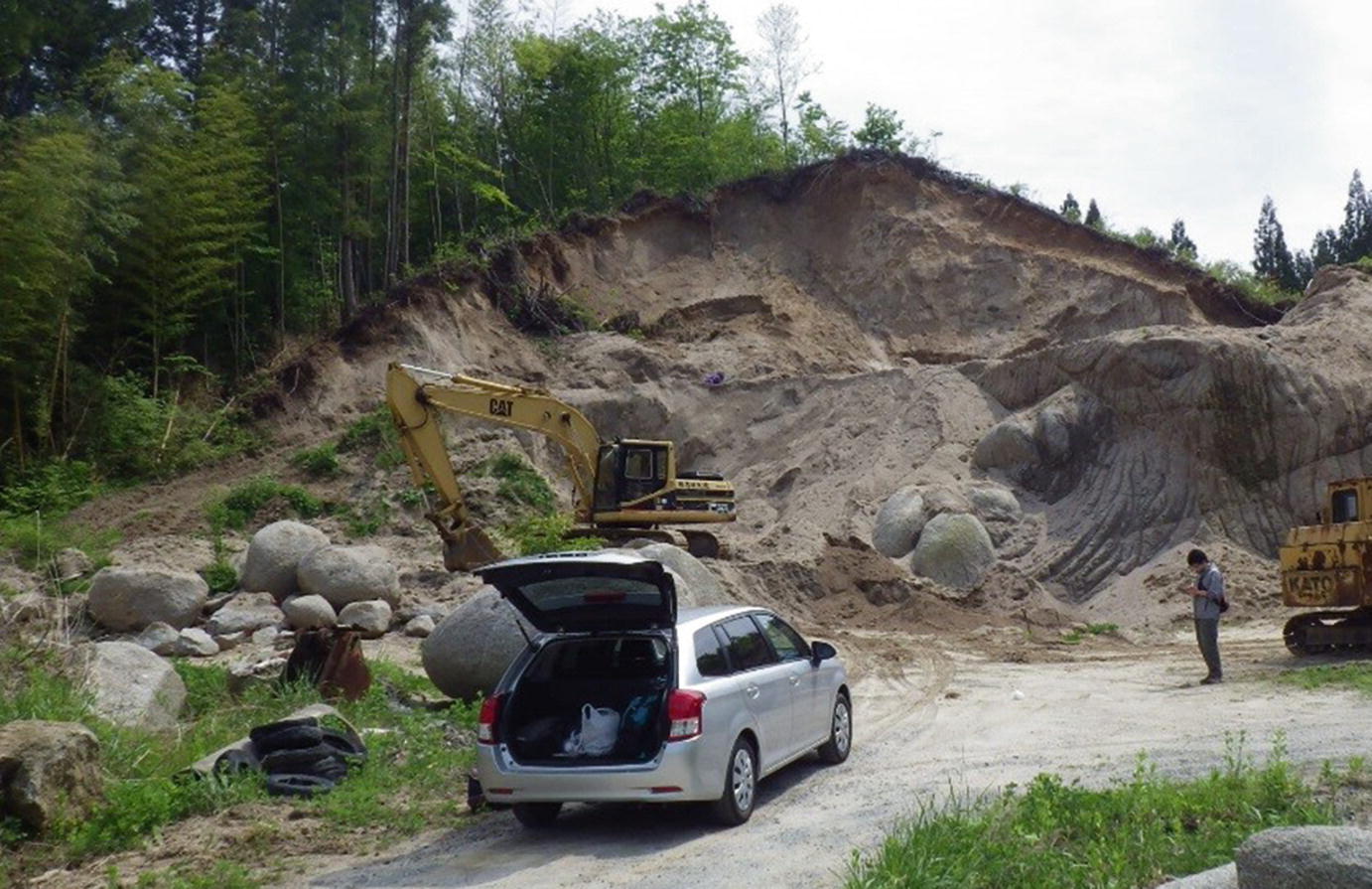
Weathered granitic soils or “Masado” consisting of hills in Fukushima (Ono-Town, Tamura-county, Fukushima Prefecture). The rounded rocks are original granite buried in the soil
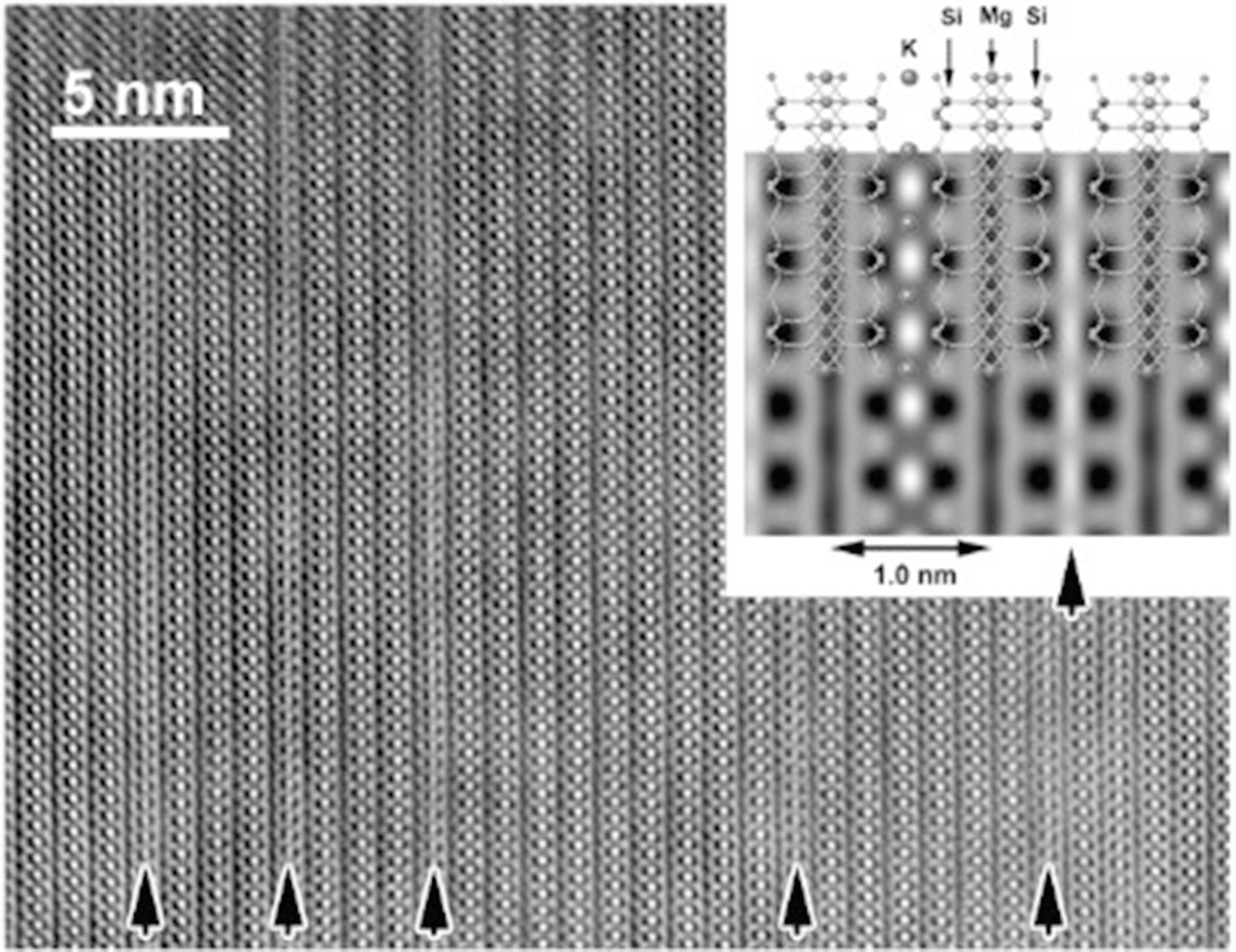
High-resolution transmission electron microscope (HRTEM) image of biotite-vermiculite interstratification in the weathered biotite collected from Fukushima. The arrowed interlayer regions where the contrast of potassium is missing were originally hydrated but collapsed by dehydration in the vacuum in TEM. The inset at the top-right is the simulated contrast for the biotite structure with the potassium (K)-occupied (left) and K-missing (right) interlayers
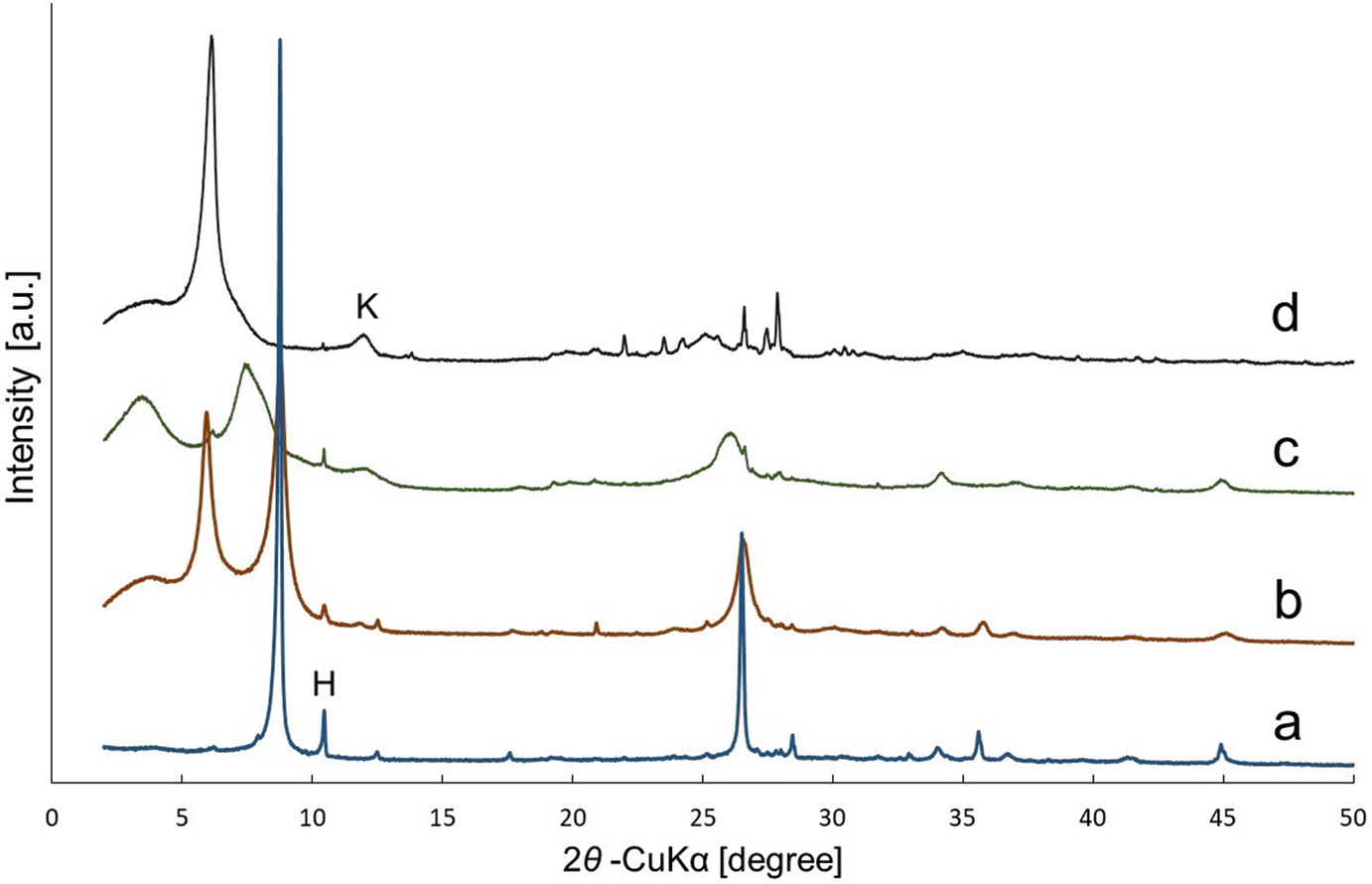
Oriented XRD patterns of various “weathered biotite” specimens in Fukushima. (a) Almost fresh or original biotite taken from granite. (b) Interstratification of the biotite and vermiculite layers. The biotite layers are dominant and the two types of layers are rather segregated. (c) Finely-mixed interstratification of the biotite and vermiculite layers. (d) Interstratification of the two types of layers and the vermiculite layers are dominant. The broad peak marked with “K” is kaolinite or halloysite, and the sharp peak marked with “H” is hornblende. (Kikuchi et al. 2015)

High-angle annular dark-field (HAADF) image of weathered biotite to which cesium was sorbed experimentally, recorded using a scanning transmission electron microscope (STEM). (Kikuchi et al. 2015)

(a) SEM image of radioactive WB cut into four fragments by FIB, and a readout IP image from the four fragments, after separating them from each other. (b) SEM image of the fragment “B”, cut into four fragments, and readout IP image of the four fragments, after separating them from each other. (Mukai et al. 2016b)

(a) SEM image of a radioactive WB from which the cross-sectional thin section for TEM was fabricated by FIB, from the area indicated with the elongated square around the center. (b) Cross-sectional TEM image of the WB in (a), showing a laminated structure with many cleavages and spaces. (Mukai et al. 2014)
7.3.1 Sorption and Desorption Behavior of Cs to WB
Based on the analyses of the radioactive soil particles in the field as described above, it is suggested that WB is an important material sorbing radiocesium in the soil, influencing the dynamics and fate of radiation in the soil around Fukushima. Hence, we investigated the Cs-sorption/desorption properties of this mineral in the laboratory. For instance, a question to be answered is why WB was frequently found as radioactive soil particles in Fukushima? Was it owing to the abundance of WB in Fukushima or its superior Cs-sorption ability? A study by NIMS (the URL is provided in the Reference section) which surveyed the Cs-sorption ability of various clay minerals after the accident indicated that WB (vermiculite in the study) does not predominantly sorb Cs, in contrast to other micaceous clay minerals like illite and smectite. However, these data were obtained using a solution with a cesium concentration as low as sub-ppm (~10−5 mol L−1). It is natural to expect that the Cs-sorption ability of minerals is dependent on the concentration of cesium in the solution and/or the solid-solution ratio because there are various sorption sites in the minerals. The actual concentration of radiocesium in the rain which caused the radioactive contamination of the soil in Fukushima is considered to be very low. For instance, the amount of rainfall in Iitate village, a seriously contaminated area in Fukushima, over a few weeks after the nuclear accident was around 10 mm according to the records of the Japan Meteorological Agency (the URL is provided in the Reference section). On the other hand, the amount of 137Cs per unit area deposited on Iitate village was ~106 Bq/m2, according to a report by JAEA (the URL is provided in the Reference section). From these values, the concentration of radiocesium in the rain should have been of the order of 10 ppt (10−10 mol L−1). To discuss the contamination event affecting the Fukushima soil, sorption experiments should be conducted with such a low concentration. However, this is close to or below the detection limit of the most sensitive analytical instruments. This problem is solved if radiocesium itself is used as the cesium source and the sorption/desorption amount is estimated by measuring the radiation of the sorbed radioisotope, as used in previous studies (Poinssot et al. 1999; Ohnuki and Kozai 2013). Moreover, we evaluated the sorption amounts of 137Cs in the minerals by measuring the radiation in the individual mineral particles quantitatively using IP autoradiography, instead of counting gamma-rays. An advantage of using IP autoradiography is that we can investigate a reaction between a solution and multi-minerals. Such reaction can reproduce more practically the event in which radiocesium in the rain was sorbed to the soil composed of several mineral species.
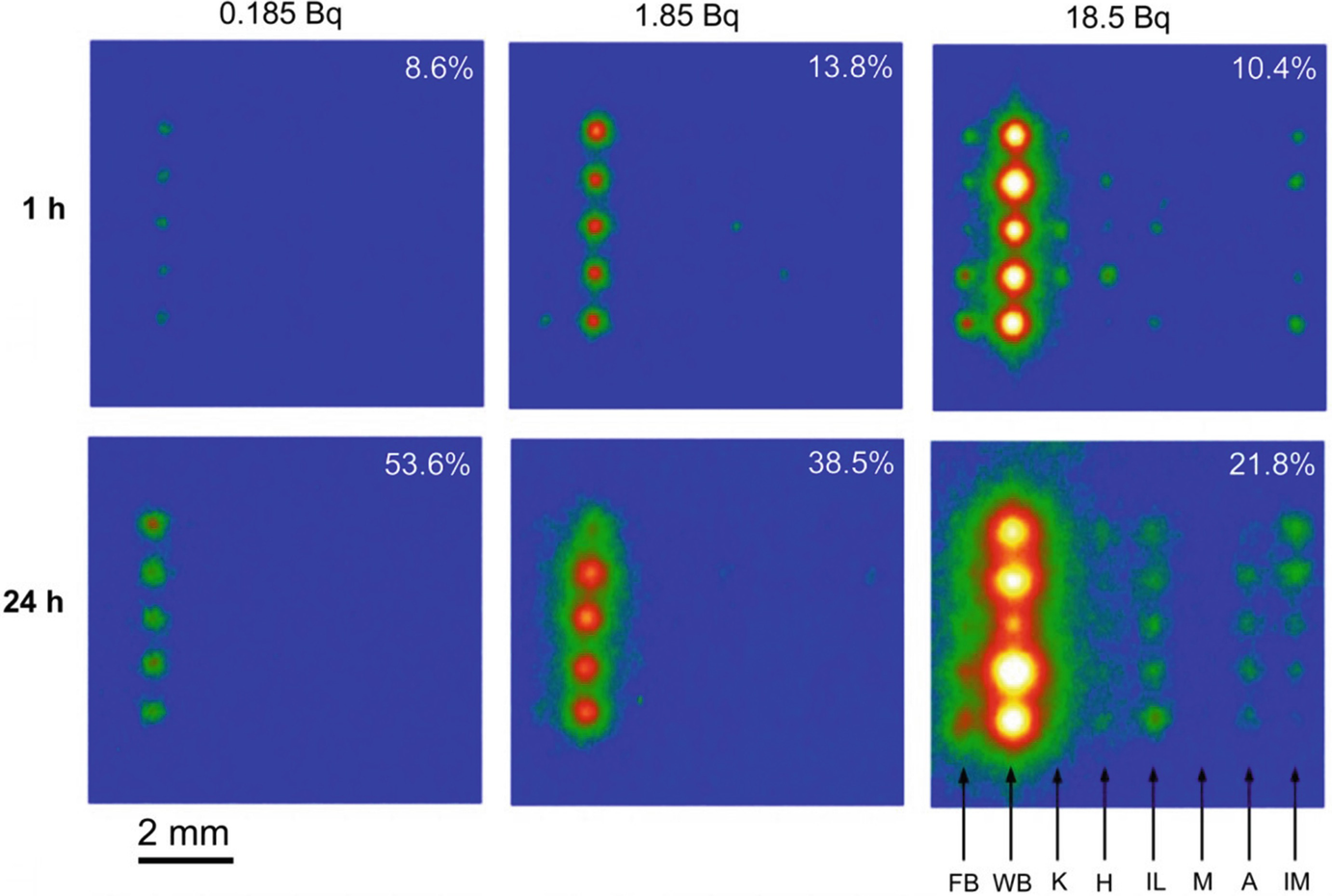
A matrix of the readout images of IPs exposed by the substrates with various mineral particles (five particles for each species) sorbed radiocesium from the solutions. The radioactivity input to the solution and reaction time are at the top and left, respectively. The figure at the top-right of each image is the percentage of radioactivity (or 137Cs) sorbed to the whole mineral particles, estimated from the IP signal. The abbreviations at the bottom-right mean FB: fresh biotite, WB: weathered biotite, K: kaolinite, H: halloysite. IL: illite, M: montmorillonite, A: allophan, IM: imogolite. (Mukai et al. 2016a)

A matrix of the readout images of IPs exposed by the substrates with four mineral particles of WB (top) and SWa-1 (bottom), reacted with 1.85 Bq 137Cs solution for various immersion times which are shown at the top. Notice that only one mineral species was placed on the substrates and the mineral particles were different for each run. (Mukai et al. 2016a)
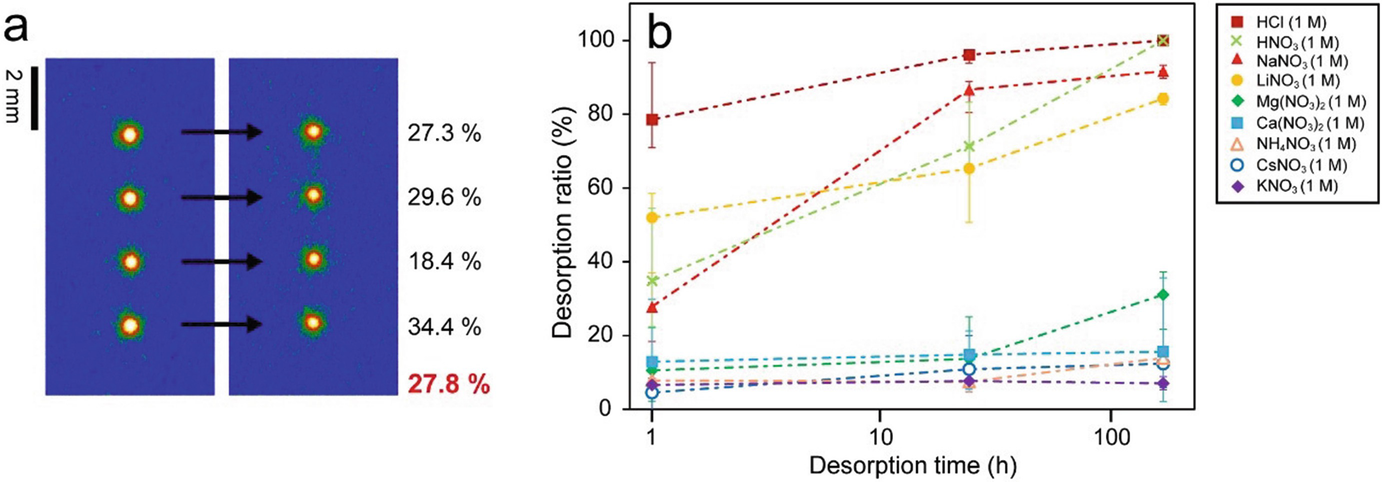
(a) An example of the desorption experiments for the weathered biotite (WB). The readout IP images on the left and right were taken from WB particles before and after immersion, respectively, in an electrolyte solution (1 M NaNO3 in this case) for a certain time. The figure represents the decrease ratio of the integrated IP intensity around the spots for each particle, and that at the bottom represents the average and is used as the “desorption ratio (%)” in (b). (b) Desorption ratios of 137Cs from WB for various solutions and immersion times. For each sample, 137Cs was sorbed to four particles of WB from a 50 μl solution with 2.5 Bq for 24 h. WB particles sorbing 137Cs were immersed in the electrolyte solutions 50 μl. Error bars represent the minimum and maximum desorption ratios in four particles as shown in (a). (Mukai et al. 2018)
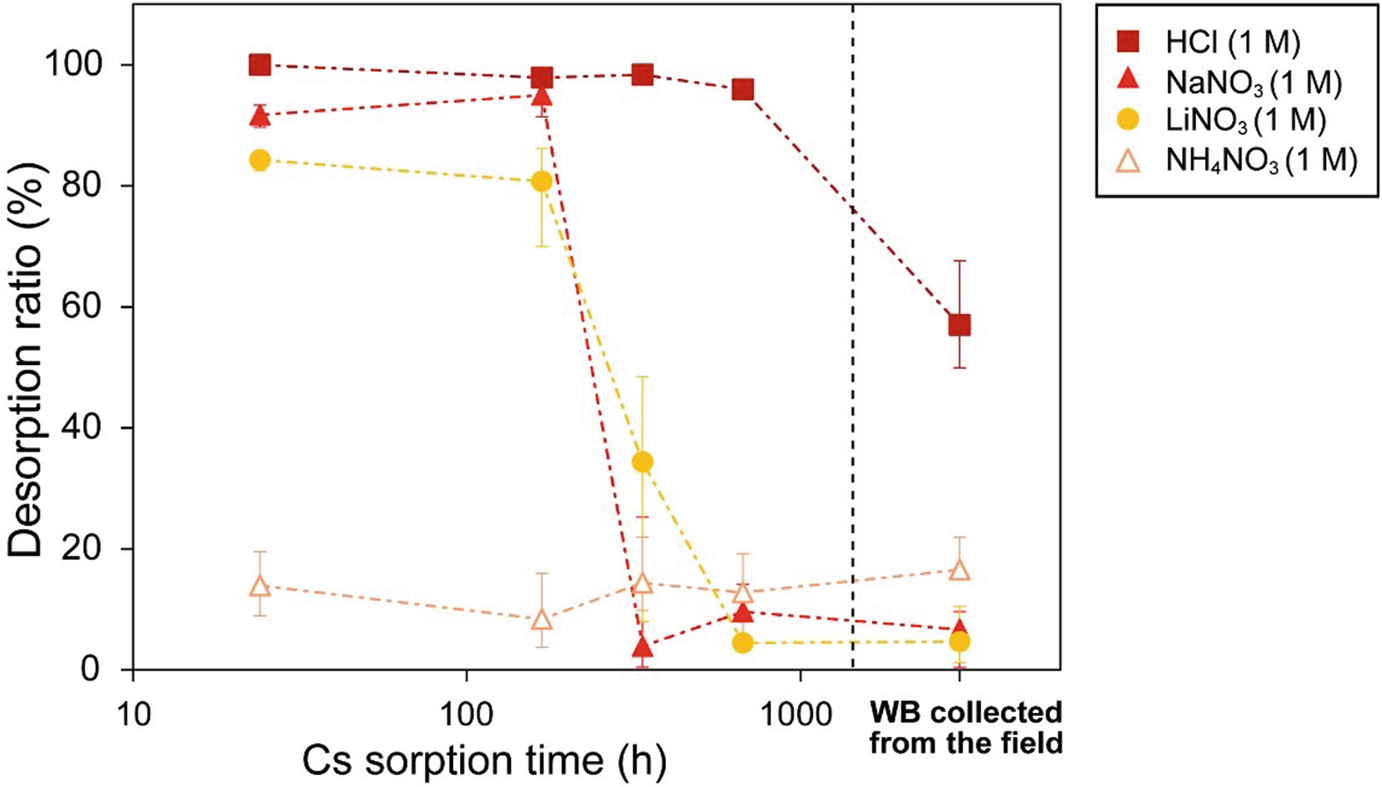
Desorption ratios of WB as a function of 137Cs-sorption time (24 h, 168 h, 336 h, and 672 h), and the desorption ratio of radioactive WB collected from the field at Fukushima. Desorption time was 168 h. (Mukai et al. 2018)
These distinct properties of WB can explain several aspects of the radioactive contamination in Fukushima. For instance, most of the radiation that fell on the ground was trapped in a very shallow depth of the soil and has hardly moved into deeper soils (Honda et al. 2015). The distribution coefficients of radiocesium between sediments and river water are far larger (rich in sediment) in Fukushima than in Chernobyl (Konoplev et al. 2016). The transfer factors of radiation (radiocesium) from the soil to plants have decreased rapidly since the accident (e.g., Takeda et al. 2013). Probably these observations in the field in Fukushima are related to the high sorption and fixation ability of WB.
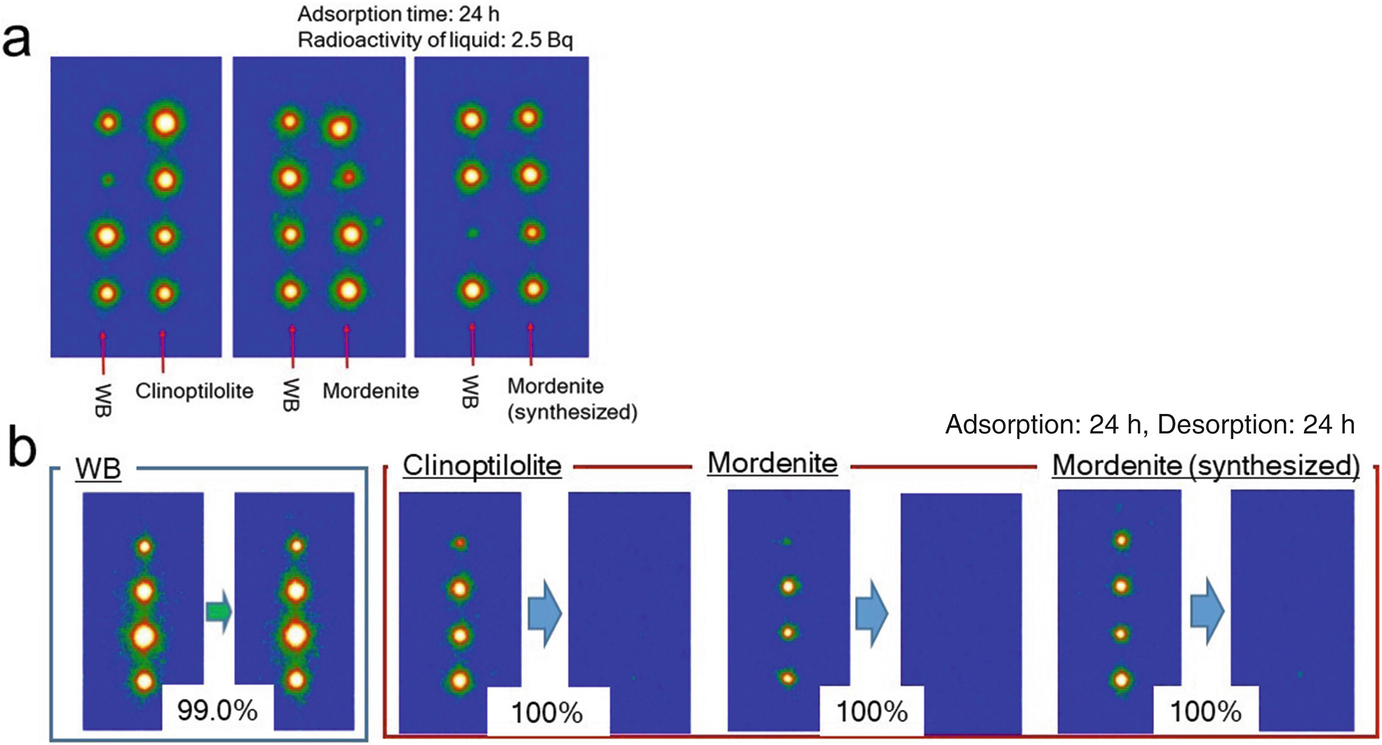
(a) Comparison of the Cs-sorption abilities between WB and several zeolites. The experimental procedure was the same as those in Fig. 7.10. (b) Desorption properties of WB and several zeolites. The solution for desorption was CsCl (1 M)
7.4 Conclusions
Radiocesium released from FDNPP has fallen on the ground where, fortunately or unfortunately, WB was abundant in the soil, and a large portion of radiocesium is now expected to be fixed in this mineral. In such areas, the dynamics of radiocesium should be considered on the basis of the character and properties of WB. Although our knowledge of WB has been increased considerably by the research carried out during the last few years, several substantial questions remain unanswered. For instance, the actual location(s) of dilute Cs in the WB structure, and the atomistic mechanism of “aging” as shown in Fig. 7.13, etc. are not clear yet. For future studies, these unknowns should be elucidated using fundamental research. Such research is still necessary because the radiation of 137Cs will not rapidly decay for several decades and because similar disasters may occur in the future.
Acknowledgements
The authors are grateful to Dr. S. Motai and Ms. E. Fujii for their collaboration in the research, Dr. T. Hatta and Dr. H. Yamada for donating the radioactively contaminated soils from Fukushima, Prof. Y. Watanabe for donating the zeolite specimens, Dr. A. Hirose, Prof. K. Tanoi, and Prof. TM. Nakanishi for the assistance with the sorption/desorption experiments using RI. This study was financially assisted by a Grant-in-Aid for Science Research (15H04222, 15H02149 and 24340133) by JSPS, Japan. This study was also supported through contracted research with the Japan Atomic Energy Agency (JAEA) for Fukushima environment recovery, entitled “Study on Cs adsorption and desorption process on clay minerals”.

Open Access This chapter is licensed under the terms of the Creative Commons Attribution 4.0 International License (http://creativecommons.org/licenses/by/4.0/), which permits use, sharing, adaptation, distribution and reproduction in any medium or format, as long as you give appropriate credit to the original author(s) and the source, provide a link to the Creative Commons license and indicate if changes were made.
The images or other third party material in this chapter are included in the chapter's Creative Commons license, unless indicated otherwise in a credit line to the material. If material is not included in the chapter's Creative Commons license and your intended use is not permitted by statutory regulation or exceeds the permitted use, you will need to obtain permission directly from the copyright holder.