9.1 Introduction
Since their discovery in 1995 (Sakaguchi et al. 1995), regulatory T cells (Treg) have been established as an anti-inflammatory T cell population which attenuates and modulates immune responses on multiple levels including initiation, progression, and termination (Campbell and Koch 2011). Over the past decades intensive research on this new T cell subtype led to deeper understanding of the intrinsic mechanisms of the immune system to contain and control inflammatory reactions. Graft-versus-host disease (GvHD) developing after allogeneic hematopoietic cell transplantation (allo-HCT) belongs to a variety of diseases, in which the immune system runs amok and attacks the own body in an autoreactive manner (Ferrara et al. 2009). As soon as the immense potential of Treg to downregulate excessive autoimmune responses became evident, multiple translational approaches have been pursued in order to unravel the suppressive capability of Treg in GvHD and be able to control or even prevent this fatal complication in the clinical setting. Ultimately, researchers and clinicians want to find a way to establish long-lasting tolerance within the graft without diminishing the beneficial graft-versus-tumor/graft-versus-leukemia (GvT/GvL) effect (Schneidawind et al. 2013).
The following chapter will give an overview of Treg characterization and functional abilities in order to explain thereafter their diverse influence in the pathogenesis of GvHD which is mainly studied in preclinical models and conclude with a review over completed and current clinical trials.
9.2 Characterization of Treg
In 1995, Sakaguchi and colleagues observed a population of cluster of differentiation 4 positive (CD4+) T cells which even in homeostatic conditions expressed high levels of CD25, the interleukin-2 receptor (IL-2R) α-chain (Sakaguchi et al. 1995). A further characterization by depletion experiments revealed their suppressive function to prevent autoimmune symptoms. Two decades later, this suppressive T cell population became known as regulatory T cells (Treg). The cells are characterized by the expression of the transcription factor Forkhead-Box-Protein P3 (FoxP3), which is not only important for the development yet also for continued suppressive functionality of Treg (Fontenot et al. 2005). Mutations within the FoxP3 locus lead to severe autoimmunity, and the genetic dysfunction of the transcription factor is associated with the fatal IPEX (immunodysregulation polyendocrinopathy enteropathy X-linked) syndrome (Bennett et al. 2001; Brunkow et al. 2001; Wildin et al. 2001). Furthermore, they strongly express the high-affinity IL-2R α-chain which developed to be a well-established Treg marker indicating the importance of IL-2. Indeed, this cytokine is essential for Treg homeostasis, survival, and function (Malek 2008; Setoguchi et al. 2005) also shown by the phenotype of CD25 deficiency in human disease (Sharfe et al. 1997). Naturally occurring Treg (nTreg) undergo the same developmental path like conventional T cells (Tcon) migrating from the bone marrow into the thymus where the lineage commitment occurs. nTreg recognize self-antigens but are not undergoing negative selection. Instead they upregulate FoxP3 and form a suppressive T cell population guarding the lymphatics and tissues from excessive immune responses (Sakaguchi et al. 2006). Nevertheless, CD4+ naïve T cells are able to develop to so-called induced Treg (iTreg) in the periphery when they are activated in a suppressive environment with the presence of transforming growth factor β (TGF-β) and IL-2 (Fantini et al. 2004; Bluestone and Abbas 2003). iTreg can also be developed in vitro by T cell receptor (TCR) stimulation together with TGF-β and IL-2 which leaves them as an attractive tool in clinical use to suppress unwanted immune responses (Fantini et al. 2004; Chen et al. 2003). Additionally, Treg can be divided depending on their location and homeostatic signaling requirements into two subsets, central and effector Treg (Smigiel et al. 2014).
Moreover, besides the classic CD4+ Treg, also the CD8+ counterpart which proves to partake in immune modulation of GvHD (Hahn et al. 2005; Beres et al. 2012) is characterized as well as several subpopulations of Treg such as Tr1 (Roncarolo et al. 2014) or Th3 (Weiner 2001) cells. Recent studies suggest that the initial bias of an immune response shapes the developing Treg which are armed with the proper features such as homing receptors to suppress Th1-, Th2-, or Th17-specific immune reactions (Koch et al. 2009; Zheng et al. 2009; Chaudhry et al. 2009). Yet, a recent study could show that this represents no stable commitment to one lineage but rather a dynamic process in order to maintain homeostatic conditions (Yu et al. 2015).
In order to fulfil their function in suppression of excessive immune responses or aid in the contraction phase after elimination of an infection, Treg have to be able to enter various tissues including lymphoid and nonlymphoid tissues. Highly functional Treg are characterized by expression of CD62L, a lymph node homing molecule which enables them to reach the secondary lymphoid organs where the T cell priming takes place (Venturi et al. 2007). Tissue-specific homing receptors such as C-C chemokine receptor type 5 (CCR5) (Yurchenko et al. 2006), CCR8 (Soler et al. 2006), or CCR9 (Guo et al. 2008) lead the way to the target organs since they are specific for the location of inflammation which needs to be suppressed and are imprinted on the Treg during their activation.
The specific mechanisms in which Treg suppress immune responses are versatile and will be discussed further on with a focus on the suppression of acute GvHD.
9.3 Immunmodulation by Treg in Preclinical Models of GvHD
A study from 2007 could prove that the progressive loss of Treg in the course of GvHD leads to continued disease pathology and conversion of acute to chronic GvHD (Chen et al. 2007). Early Treg depletion studies during allo-HCT indicated an essential role of these cells for tolerance to alloantigen and a highly deteriorated GvHD when Treg were lost (Taylor et al. 2001). Sawamukai et al. further investigated the donor Treg composition and found that nTreg as well as iTreg and both T cell subsets, CD8+ and CD4+ Treg, partake in the protection from GvHD (Sawamukai et al. 2012). Nevertheless, not only donor Treg contained in the graft attenuate alloimmune responses, but also recipient-type Treg which survive the conditioning regimen were able to dampen disease development (Anderson et al. 2004; Bayer et al. 2009).
Based on the finding that donor Treg play a major role in the regulation of alloimmunity, in the following years, several murine adoptive transfer studies were performed in parallel in order to fathom the possibility of improving GvHD by cellular therapy (Cohen et al. 2002; Hoffmann et al. 2002; Taylor et al. 2002; Edinger et al. 2003; Jones et al. 2003; Trenado et al. 2003).
By adding Treg to the transplant, either freshly isolated from a donor (Hoffmann et al. 2002) or expanded ex vivo before transplantation (Taylor et al. 2002), the development of acute GvHD could be prevented. In addition, Jones et al. proved the efficacy of Treg co-transplantation also in a minor mismatch model since in the clinical setting major mismatch transplantations are virtually never performed any more (Jones et al. 2003). Furthermore, first approaches were conducted with the selection and expansion of allo-specific Treg by coculture with recipient antigen-presenting cells (APCs) (Trenado et al. 2003). Despite the unsatisfactory results compared to unspecific Treg, this approach is further evaluated in order to generate Treg specific against major and minor histocompatibility antigens without diminishing the GvL effect (Veerapathran et al. 2011).
Studying the kinetics of allogeneic T cell activation, expansion, and effector phase, Treg were proven to be essential in the early phases after bone marrow transplantation (BMT) in order to efficiently suppress T cell expansion and GvHD development (Edinger et al. 2003). Transplantation of Treg at later time points severely diminished their efficacy to prevent GvHD (Nguyen et al. 2007). Moreover, the transplantation of Treg prior to Tcon allowed for a transfer of smaller numbers since Treg strongly expand after BMT in vivo. Treg only represent 5–10% of all peripheral CD4+ T cells which leaves isolation of large numbers of this cell subset challenging (Sakaguchi et al. 2006). One way to increase the number of transplantable cells is ex vivo expansion with a classic protocol of IL-2 administration together with CD3/CD28 stimulation (Taylor et al. 2002; Hoffmann et al. 2004; Earle et al. 2005). Nevertheless, the contamination of the starting cell pool with Tcon has to be kept in mind since it could lead to an unpreferential expansion of effector T cells (Battaglia et al. 2005).
Another possibility to overcome low Treg numbers is the in vitro induction and expansion of iTreg by generation from Tcon as mentioned above. Initial studies demonstrated a serious problem emerging when using in vitro generated Treg (Fantini et al. 2004; Beres et al. 2011). The Treg quickly lost FoxP3 expression and adopted an inflammatory T cell phenotype failing to prevent GvHD. FoxP3 expression is epigenetically controlled by hypomethylation of a conserved region in the foxp3 promoter. In contrast to nTreg, iTreg remain only partially demethylated leading to a progressive loss of FoxP3 expression upon restimulation and absence of suppressive TGF-β (Floess et al. 2007). Novel approaches including hypomethylating agents such as decitabine in the protocol show promising results concerning a sustained FoxP3 expression (Choi et al. 2010).
Nevertheless, clinical approaches which include in vitro handling of human cells always require elaborate cell culture techniques to ensure safety which leaves them at substantial costs. An alternative is provided by studies attempting the in vivo expansion of nTreg. Blockade of the IL-6 signaling pathway increased Treg frequency with simultaneous reduction of effector T cells in a murine allo-HCT model (Chen et al. 2009). Furthermore, studies with CD28 antibody (Kitazawa et al. 2009) as well as CD1d ligand (Duramad et al. 2011) show promising results for the in vivo expansion of Treg and the resulting attenuation of GvHD in preclinical models which yields hope for possible implementation in the clinic. A recent publication shows that administration of an anti-CD45RB monoclonal antibody resulted in increased Treg-APC interaction which led to enhanced Treg proliferation (Camirand et al. 2014).
Several groups reported that rapamycin in combination with IL-2 application was efficient in the expansion of T cells with a regulatory phenotype (Shin et al. 2011; Zhang et al. 2013) which led to extensive research investigating the practical range and the mechanism behind these observations. Since rapamycin exclusively expands Treg by simultaneously inhibiting other T cell subsets (Battaglia et al. 2005, 2006; Tresoldi et al. 2011), the drug represents a powerful tool to expand Treg for further use for adoptive transfer (Battaglia et al. 2012). In a murine model of acute GvHD, the transplantation of Treg and rapamycin treatment showed a synergistic effect by improving disease outcome and preserving long-term tolerance by highly active Treg (Zeiser et al. 2008). Furthermore, the authors could show that, in response to IL-2 and alloantigen stimulation, Treg upregulated signal transducer and activator of transcription 5 (STAT5) by simultaneous high expression of phosphatase and tensin homolog (PTEN), a negative regulator of the phosphatidylinositol-3-kinase/protein kinase B/mammalian target of rapamycin (PI3K/Akt/mTOR) pathway. In order to understand the mechanism behind the differential responses of Treg and Tcon to rapamycin treatment, Sauer et al. brought evidence for the antagonizing cross talk of TCR signaling and subsequent activation of the PI3K/Akt/mTOR cascade and FoxP3 expression (Sauer et al. 2008). This leads to the conclusion that Treg are not impacted by immune modulation by rapamycin since their signal for survival and function passes through the IL-2R without activating the PI3K/Akt/mTOR pathway (Malek and Castro 2010). In this T cell subset, the signaling cascade is interrupted through high expression of the phosphatase PTEN (Bensinger et al. 2004). The block leaves signaling through the JAK-STAT pathway intact leading to the known hypoproliferative phenotype because of the impaired response to IL-2 signaling compared to Tcon (Walsh et al. 2006).
9.4 Mechanism of Immunosuppression by Treg
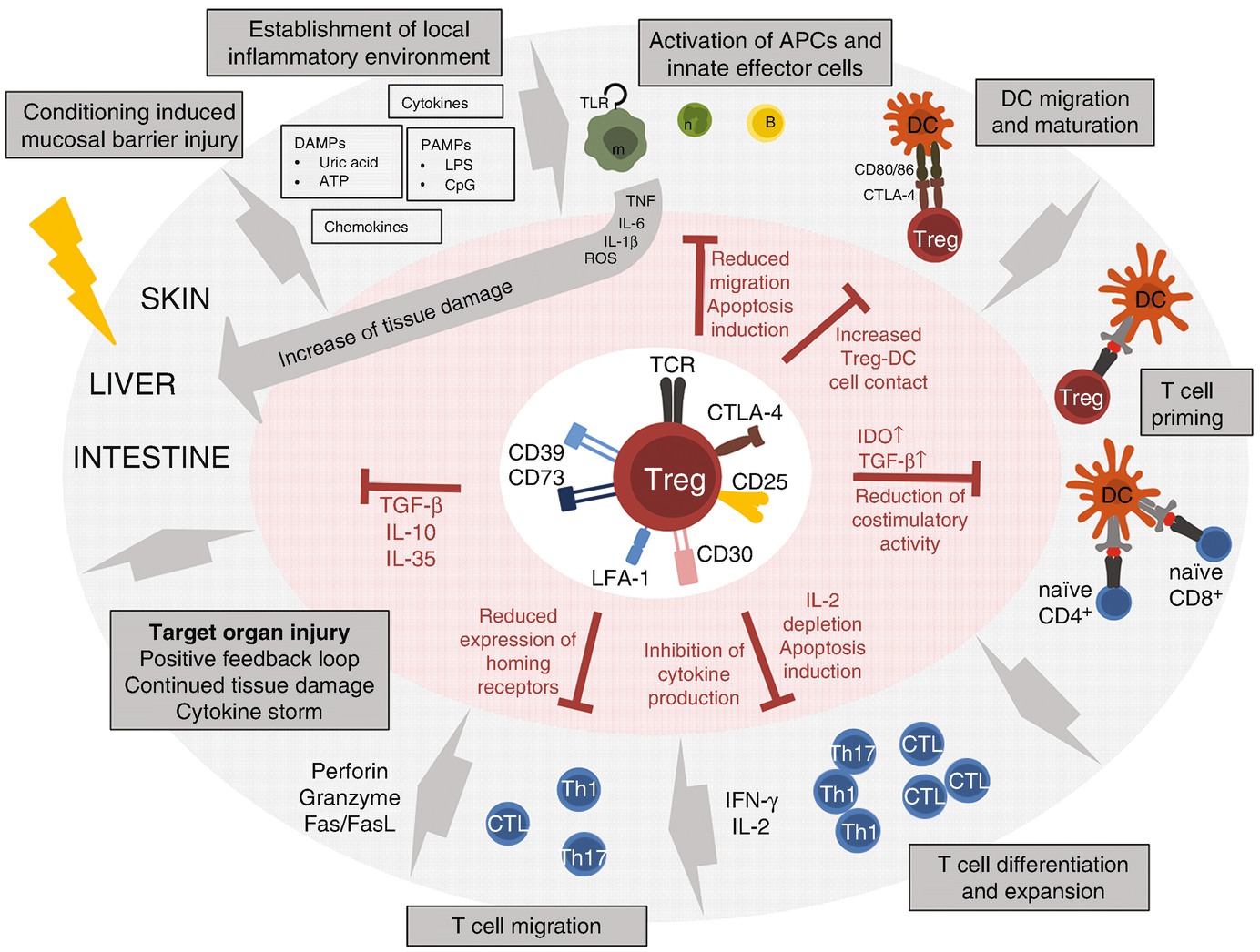
The conditioning regimen such as irradiation or chemotherapy which is necessary in the recipient of allo-HCT to create space for the new immune system and eliminate the malignant cells, the underlying disease, or previous infections leads to host tissue damage in particular in the gut. The resulting release of DAMPs and PAMPs as well as cytokines and chemokines by the local cells leads to an establishment of the inflammatory environment and the activation of local immune cells by their pattern recognition receptors (PRRs). Macrophages and neutrophils in turn secrete inflammatory cytokines such as TNF or IL-6 which enhance the immune activation and aggravate the existing tissue damage. DCs maturing in the periphery migrate to the secondary lymphoid organs where they present host-specific antigens and prime naïve allogeneic T cells which represents the central reaction in GvHD pathogenesis. The activated T cells proliferate and secrete cytokines such as interferon γ (IFN-γ) and IL-2 which contribute to the development of a Th1-biased response. Ultimately, T cells migrate to the target tissues where they destroy cells from the liver, skin, and gastrointestinal tract by mechanisms involving perforin, granzymes, and Fas/FasL. The resulting cell death leads to the continued release of danger signals which perpetuates the GvHD reaction and induces the characteristic cytokine storm. Treg cells are able to interfere at multiple levels within this process in order to attenuate GvHD (references are cited in the main text). (1) Maturation and AG presentation of APC: Treg are able to impair the maturation, migration, and effector function of innate immune cells. Furthermore, they induce apoptosis in B cells and neutrophils. Treg reduce the costimulatory activity of DCs by means of CTLA-4 binding and destabilize the contact of effector T cells with DCs. (2) Inhibition of effector T cells: Treg constrain proliferation by means of IL-2 depletion. Moreover they limit cytokine production and survival by inducing a cytokine-deprived milieu and decrease the expression of homing receptors leading to impaired migration of the effector cells. (3) Effects on local inflamed tissue: Treg secrete a variety of anti-inflammatory cytokines including TGF-β, IL-10, and IL-35 which dampen inflammation
Generally, Treg are anergic, therefore do not proliferate upon antigen stimulation, yet need TCR stimulation for activation. Since Treg are selected in the thymus by their increased autoreactive potential, they are prone for self-recognition (Hsieh et al. 2004) and only a small stimulus suffices for activation (Takahashi et al. 1998). Thereupon, Treg exhibit suppressive activity regardless of antigen specificity which gives reason for hope in treatment of allo-HCT as Treg/Tcon histocompatibility seems not to be essential for suppression (Thornton and Shevach 2000). Nevertheless, recent findings indicate that sustained TCR signaling is required for Treg suppressive function (Levine et al. 2014).
In the initial phase of acute GvHD, when the conditioning regimen, irradiation or chemotherapy, leads to local tissue damage, pathogens and so-called pathogen-associated molecular patterns (PAMPs) cross the damaged epithelial barriers of the skin and gastrointestinal tract (Fig. 9.1). Cytokines, chemokines, adhesion molecules, and danger signals (danger-associated molecular patterns, DAMPs) are expressed which ultimately induce the maturation of host dendritic cells (DCs) and the priming of alloreactive T cells (Zeiser et al. 2011; Hill and Ferrara 2000). In order for the Treg to prevent APC and Tcon activation, they have to enter the draining lymph nodes by means of CD62L expression since it has been proven that GvHD is only efficiently suppressed by CD62Lhi Treg (Taylor et al. 2004; Ermann et al. 2005). Furthermore, expression of homing receptors such as CCR5 mentioned above is essential for the migration in the target tissues in the later stages of GvHD.
Reducing the costimulatory activity and ability of APCs represents the first level of suppression of T cell activation managed by Treg. Already early after Treg discovery, Cederbom and colleagues could show decreased CD80 and CD86 expression on DCs (Cederbom et al. 2000). In 2006, two groups could prove with two-photon microscopy that Treg have long sustained contact with DCs while inhibiting prolonged binding of Tcon with their APCs in the secondary lymphoid organs (Tadokoro et al. 2006; Tang et al. 2006). One major receptor responsible for inhibition of immune activation by Treg is cytotoxic T-lymphocyte-associated protein 4 (CTLA-4), a high-affinity receptor for CD80 and CD86. Its essential role became apparent in receptor-deficient mice developing severe lymphoproliferative disorders (Tivol et al. 1995). Since a direct inhibitory signaling pathway emanating from CTLA-4 engagement remains still controversial, common hypotheses include that one mechanism of DC inhibition originates from competitive binding of CD80/86 by means of higher affinity of CLTA-4 compared to CD28 (Salomon and Bluestone 2001). This theory is supported by data that show an upregulation of lymphocyte function-associated antigen 1 (LFA-1) by CTLA-4 which may result in even increased sustained cell-cell contact between Treg and APC (Schneider et al. 2005). Recent findings suggest a second mechanism in which by a process called trans-endocytosis CTLA-4 binds and removes CD80/86 from the DC surface by internalization and degradation within the Treg leading to a profound and sustained DC suppression (Qureshi et al. 2011). Moreover, CTLA-4 induces indoleamine dioxygenase (IDO) production in DCs thereby initiating tryptophan metabolism (Grohmann et al. 2002). Tryptophan depletion strongly inhibits T cell proliferation and cytokine production (Fallarino et al. 2003), and IDO expression may also lead to a tolerogenic phenotype of the IDO-expressing cells themselves (Mellor and Munn 2004). IDO represents a major suppression-inducing molecule since it also stimulates the new development of Treg by high TGF-β production of DCs as well as the strong activation of existing Treg and the inhibition of reprogramming of Treg into inflammatory T cells (Chung et al. 2009; Yan et al. 2010; Munn 2011).
Lymphocyte-activation gene 3 (LAG-3) represents another Treg receptor which binds major histocompatibility complex (MHC) class II and may mediate suppression of Tcon and APC activation (Huang et al. 2004; Liang et al. 2008). Moreover, LAG-3 is also expressed by Tcon which leaves these cells more susceptible to regulation by Treg (Durham et al. 2014). Further inhibitory surface molecules of Treg include CD30 which has been shown to be essential during early Treg protection from GvHD (Zeiser et al. 2007), as well as CD39 and CD73 which degrade the danger signal extracellular adenosine triphosphate (ATP) to the immunomodulatory molecule adenosine leading to metabolic inhibition of effector T cells (Deaglio et al. 2007; Han et al. 2013).
Besides the receptors involved in Treg activation and enhancement of suppressor function, multiple publications describe glucocorticoid-induced tumor necrosis factor-related protein (GITR) expressed on Treg as a negative regulator which upon stimulation diminishes Treg suppression (Shimizu et al. 2002), while the role of Toll-like receptors (TLRs) such as TLR8 or TLR4 in dampening or inducing Treg responses seems to depend on the type of TLR (Peng et al. 2005; Caramalho et al. 2003).
Nevertheless, Treg also exhibit direct killing mechanisms by means of perforin release for DC killing (Boissonnas et al. 2010) as well as granzyme A and B in order to induce apoptosis in target cells such as CD4+ and CD8+ T cells, monocytes, and DCs (Zeiser et al. 2007; Grossman et al. 2004a, b).
Besides inhibition of DCs, several groups investigated Treg-suppressive capability of B cells regarding the interference with activation, class switch, and antibody production as well as apoptosis induction by perforin and granzymes, the programmed cell death protein 1 (PD-1)/PD-L1 interaction, or the Fas/FasL pathway (Zhao et al. 2006; Lim et al. 2005; Gotot et al. 2012; Xu et al. 2016; Janssens et al. 2003). B cell inhibition is worth consideration in the context of GvHD since B cells are able to act as APCs for effector cells and play a major role in chronic GvHD.
Recent data prove the importance of early neutrophil activation and migration into target organs for the pathogenesis of acute GvHD (Schwab et al. 2014) which leaves inhibition of this cell compartment by Treg during GvHD of serious interest. Treg cells are not only able to reduce migratory potential and induce apoptosis in neutrophils (Richards et al. 2010; Lewkowicz et al. 2006), yet a recent study found that Treg could even promote immunosuppressive features in neutrophils resulting in their contribution to immune modulation (Lewkowicz et al. 2013). Regarding other innate immune cell compartments, Treg are also able to inhibit the cytotoxic capacity of natural killer (NK) cells in a cell-cell contact-dependent mechanism (Trzonkowski et al. 2004). Moreover, Treg were shown to directly downregulate monocyte and macrophage activation leading to reduced cytokine secretion and stimulatory molecule expression (Taams et al. 2005) as well as induce apoptosis in monocytes by means of Fas/FasL interaction (Venet et al. 2006).
Besides the vast possibilities of constraining APC activation by Treg, several studies describe mechanisms of Tcon inhibition including the impaired expression of homing receptors, adhesion molecules, and chemokine receptors leading to reduced migration (Mavin et al. 2012; Sarween et al. 2004) as well as impaired proliferation (Edinger et al. 2003; Trenado et al. 2003) and cytokine production (Bergerot et al. 1999). The consistent expression of the high-affinity IL-2 receptor chain CD25 is not only required for Treg survival and function, yet is also thought to deplete IL-2 access to Tcon resulting in apoptosis induction by means of cytokine deprivation (Pandiyan et al. 2007). Furthermore, Treg can not only interact with DCs via CTLA-4 but also with Tcon thereby inhibiting T cell priming (Fig. 9.1) (Matheu et al. 2015). Several studies show the interference of Treg with CD8+ cytotoxic activity, which is either cell contact mediated or originates from a TGF-β-dependent mechanism (Trzonkowski et al. 2004; Mempel et al. 2006).
TGF-β represents a major factor of tolerance induction since its presence without further inflammatory stimuli such as IL-6 leads to FoxP3 expression and the generation of iTreg in the periphery (Chen et al. 2003). Moreover, TGF-β enhances the expansion and suppressive activity of Treg (Huber et al. 2004) while simultaneously suppressing effector T cell proliferation and function and immunoglobulin production of B cells (Chen et al. 2005; Green et al. 2003; Nakamura et al. 2001). Lastly, Treg interfere with DC maturation in a TGF-β-dependent manner (Misra et al. 2004).
IL-10 represents another important effector molecule of Treg during GvHD since IL-10 mediates immunosuppression particularly at epithelial barriers with contact to the environment such as the lungs or the skin representing target organs in GvHD (Rubtsov et al. 2008). Several studies indicate the important role of IL-10 secretion likely by donor Treg in the regulation of GvHD induction (Hoffmann et al. 2002; Tawara et al. 2012). These data are supported by a study which found a correlation between polymorphisms in the IL10 promoter and GvHD (Lin et al. 2003).
Investigations by the group around Dario Vignali revealed IL-35 as an important suppressive cytokine produced and secreted by Treg (Collison et al. 2007). On the one hand IL-35 suppresses proliferation of effector T cells which is partly contact dependent since the secretion of IL-35 is increased upon Treg-Tcon cell contact (Collison et al. 2009). Furthermore, IL-35 drives the conversion of Tcon to induced Treg (iTr35) whose regulatory activity is mainly driven by IL-35 (Collison et al. 2010).
Altogether, the data presented here draw a complex picture of Treg cell inhibition at multiple levels of an immune response including direct cell contact-mediated effects as well as indirect mechanisms of soluble factor secretion and metabolic inhibition of the target cells.
9.5 Impact of Treg on Antitumor Immunity
Multiple studies particularly in solid tumors delineate the role of Treg in the establishment of tumor tolerance and escape from immune surveillance with Treg infiltrating the tumor tissue and inhibiting an effective T cell response against the cancer cells. Curiel et al. could show in ovarian cancer patients that human Treg specifically inhibit antitumor activity of T cells and contribute to tumor progression. Their accumulation in the tumor mass was supported by CCL22 production by the cancer cells themselves inducing an immune-privileged site; therefore the authors suggest the modulation of Treg function in order to tackle cancer immune escape (Curiel et al. 2004). Besides direct inhibition of T cell immunity, Treg are able to condition intratumoral APCs which could then contribute to tumor tolerance (Bauer et al. 2014). Similar findings were made for gastrointestinal malignancies (Sasada et al. 2003); metastatic melanoma (Vence et al. 2007); breast (Gobert et al. 2009), lung (Tao et al. 2012), and pancreatic cancer (Hiraoka et al. 2006); hepatocellular carcinoma (Fu et al. 2007); classic Hodgkin lymphoma (Schreck et al. 2009); and B cell non-Hodgkin lymphoma (Yang et al. 2006) as well as acute myelogenous leukemia (Szczepanski et al. 2009). These observations led to intensive studies of interrupting Treg tolerance to increase antitumor immunity by using CTLA-4 blockade (Phan et al. 2003), anti-GITR monoclonal antibody (Ko et al. 2005), or antiangiogenic treatment (Adotevi et al. 2010).
Since an expansion of Treg during allo-HCT represents an interesting option in order to prevent GvHD, the urgent question emerged if increased Treg counts result in diminishment of the greatly appreciated (GvT/GvL) effect. Already early publications showed that engraftment and long-term tolerance were facilitated in the presence of donor Treg (Hanash and Levy 2005; Joffre et al. 2004) while preserving GvL mechanisms (Edinger et al. 2003; Jones et al. 2003; Trenado et al. 2003).
Reasons for the different outcomes of Treg expansion after allo-HCT regarding relapse lie in the unique setting after BMT concerning alloreactivity and tumor cell counts. The number of tumor cells is greatly reduced after the conditioning regimen facilitating their elimination by the transplanted immune system. The diverse mechanisms of Treg suppression of allogeneic immune responses discussed in the chapter above mostly limit the extent of T cell activation, their proliferation, and migration yet without impairing their individual cytotoxic capacity (Edinger et al. 2003). Since the count of tumor cells after BMT is rather low, the transplanted Tcon are able to eliminate the minimal cancer residue without an excessive allogeneic immune response. Furthermore, novel approaches reach to induce alloantigen-specific Treg which efficiently prevent GvHD without a possible impairment of GvL effects (Veerapathran et al. 2011; Semple et al. 2011; Li et al. 2015).
Moreover, the immune reactivity of the cancer cells is not limited to specific tumor antigens which may be already downregulated in later stages of disease but include classic self-antigens which are recognized by the alloreactive T cells of the graft leaving them more immunogenic (Kloosterman et al. 1995; Reddy et al. 2005). Donor lymphocyte infusions in case of a relapse utilize this effect.
Furthermore, the Treg efficacy is a space-limited process where Treg have to enter similar tissues as effector T cells such as the epithelial GvHD target organs. At later time points after BMT, Treg migrate to the target organs in order to suppress GvHD. Emerging leukemic cells reside in the secondary lymphoid organs or the bone marrow which hosts low Treg numbers (Booth et al. 2010) where they can be eliminated by effector T cells (Nguyen et al. 2007). Supporting this hypothesis, Kim et al. restrained T cells within the secondary lymphoid organs resulting in efficient GvL without GvHD development (Kim et al. 2003).
9.6 Clinical Application of Treg Transfer
The transfer of a tolerogenic cell population such as Treg, mesenchymal stroma cells, or Tr1 cells which persist in the body could ideally lead to long-term tolerance without the need for additional pharmacological interventions. This concept has been recently applied when Treg were transferred in the prophylactic setting to patients that had undergone allo-HCT.
The first study on Treg transfer was performed in 28 patients with high-risk hematologic malignancies who underwent human leukocyte antigen (HLA)-haploidentical HCT and showed that Treg prevented GvHD and promoted immune reconstitution (Di Ianni et al. 2011). The authors reported that Treg prevented GvHD in the absence of any posttransplantation immunosuppression and enhanced lymphoid reconstitution compared to historical controls without any evidence for an increased relapse rate (Di Ianni et al. 2011). Another group studied the safety profile of umbilical cord blood (UCB)-derived Treg in 23 patients (Brunstein et al. 2011). After infusion, UCB Treg could be detected for 14 days, indicating that the infused cells survived the proinflammatory cytokine milieu in patients post-HCT. The authors compared their patients with identically treated 108 historical controls without Treg and found a reduced incidence of grade II–IV acute GvHD (43% vs 61%, P = 0.05) with no deleterious effect on risks of infection (Brunstein et al. 2011). The same group reported later on the transfer of UCB-derived Treg that had been expanded in cultures stimulated with K562 cells modified to express the high-affinity Fc receptor (CD64) and CD86. The authors reported that the incidence of grade II–IV acute GvHD at 100 days was 9% in the UCB-Treg group compared to 45% in control patients (Brunstein et al. 2016).
Besides those preemptive Treg transfer studies, more evidence indicates a central role for Treg in prevention of GvHD post allo-HCT. Patients who received the antibody mogamulizumab for their adult T cell acute lymphoblastic leukemia (T-ALL) experienced prolonged suppression of normal Treg (Ishida et al. 2012). Importantly, pretransplant use of mogamulizumab in those T-ALL patients that later underwent allo-HCT was connected to severe acute GvHD, which is consistent with a suppressive role of Treg against GvHD (Inoue et al. 2016).
Since it was shown that Treg require IL-2 post allo-HCT to expand and survive (Zeiser et al. 2006), multiple studies later investigated the effects of IL-2 on Treg expansion in patients and GvHD (Koreth et al. 2011, 2016). In a recent phase 2 study, 35 adult patients with steroid-refractory chronic GvHD received daily IL-2 (1 × 106 IU/m2/d) for 12 weeks (Koreth et al. 2016). The authors reported that 20/33 evaluable patients (61%) had clinical responses at multiple chronic GvHD sites such as the liver, skin, and gastrointestinal tract (Koreth et al. 2016). An important predictor of response seemed to be initiation of IL-2 early after transplantation, suggesting that later established chronic GvHD is harder to modify by IL-2 treatment.
Furthermore, investigators used human donor Treg that were cultivated for 7–12 days and then given to patients suffering from chronic GvHD (Nishimori et al. 2012). The authors reported that two of five patients showed a clinical response with improvement of chronic GvHD symptoms and three patients showed stable symptoms for up to 21 months (Theil et al. 2015). Further clinical trials are in progress and planned to extend these studies also to the treatment of acute GvHD by adoptive Treg transfer.
Summarized, the findings support the attractive option of Treg expansion in patients undergoing allo-HCT in order to suppress the development of GvHD with simultaneous preservation of the appreciated anti-infectious immunity and GvL effect which in turn allows for reduced intensity conditioning regimens. First clinical trials are promising but need confirmation in larger prospective randomized trials.
9.7 Expert Point of View and Future Directions
Since their discovery, Treg have risen rapidly to a well investigated cell population with various possibilities of application. Profound characterization of Treg markers enables the specific enrichment of this regulatory cell compartment which makes them an interesting tool for clinical application. Furthermore, due to their unique signaling pathways such as their specific response to IL-2 treatment and rapamycin, protocols for the efficient expansion have been developed and are already applied successfully. Since classic standard prophylaxis and treatment options for GvHD do not interfere and even possibly enhance Treg function, including rapamycin, glucocorticosteroids, and FTY720, the combined therapy could even provide a synergistic effect, while recent studies indicate that therapy with calcineurin inhibitors has an adverse effect on Treg concerning proliferation and function and MMF application is still discussed controversially (Wu et al. 2012; Scotta et al. 2016; Demirkiran et al. 2009; Lim et al. 2010). First clinical trials show promising results concerning acute as well as chronic GvHD disease outcome both by in vivo as well as in vitro expansion of Treg. Nevertheless, intense research has to be continued in order to further improve the experimental protocols such as the optimal time point of adoptive transfer and investigate the best option for Treg expansion regarding efficacy and safety. Furthermore, the various mechanisms of Treg suppression have to be delineated to better understand their role in the clinical picture of GvHD.
A clinically valuable approach may be the use of third-party Treg and was investigated by Pierini et al. (2015). The researchers suggested this method as more practical since the availability and access of Treg in certain allo-HCT settings such as grafts from cord blood or an unrelated donor may be limited. Furthermore, the Treg could be easily stored and readily applied when most needed. Yet, due to the observed reduced survival of third-party Treg, this technique needs more profound studies. Another urgent question to be clarified is the characterization of common antigens inducing a GvHD reaction. With this knowledge, allo-specific Treg could be generated which would limit their suppressive activity to alloreactive Tcon. Lastly, certain patient cohorts may be more susceptible to Treg application, and in these, adoptive Treg transfer may be found to be favorable depending on their type of disease pathology.
Concluding, clinical application of Treg for prophylaxis and treatment of GvHD is an upcoming treatment option which not only prevents GvHD by simultaneously preserving the GvL effect but leads to profound long-term immunotolerance instead of medically induced immunosuppression which ceases upon discontinuation of treatment.