A Note Concerning the Usage of Species Names in This Chapter
For many decades, the genus name Cordyceps was used when referring to any and all of the insect-inhabiting ascomycetes fungi used in medicine or associated with this class of fungi. Specifically, the name Cordyceps sinensis was used extensively to describe both the naturally occurring wild-collected insect larva and fungi complex and the cultivated anamorph (asexual mycelial form) varieties. Today, the term “Cordyceps” is still in nearly universal use by the public, when referring to all forms of dietary supplements manufactured from this class of fungi, regardless of the actual genus and species used. However, the huge grouping including these fungi is basically a mega genus, containing many hundreds of species. No one is even certain of the exact number of species included in his group. To try to clarify this taxonomy (naming) nightmare, in 2007 a complete and comprehensive phylogenetic study of the Cordyceps group was performed and published under the title Phylogenetic Classification of Cordyceps and the Clavicipitaceous Fungi by Gi-Ho Sung and associates (Sung et al. 2007). In this landmark study, they were able to break the Cordyceps group into different genera and subgenera based upon their molecular ID, by using modern methods of multigene DNA sequencing rather than depending on the traditional morphology-based identification. One of the major results of this work was to reclassify Cordyceps species into a number of different genera: with most species falling into the genera Cordyceps, Metacordyceps, Elaphocordyceps, and Ophiocordyceps, as well as a few species falling into other genera and subgenera, based primarily on their DNA but also on the spore characteristics. However, this renaming of popularly used species has also led to quite a bit of confusion when dealing with the commercial products on the market today but also when looking at the research done on this fascinating group of fungi over the last four or five decades. In order to help clarify this and to make the naming issue a bit more understandable for those readers who are not professional mycology taxonomists, several names have been used in this chapter for these fungi: The term Cordyceps sensu lato, which in Latin means the entire or overall grouping of Cordyceps-type fungi, is used where appropriate, to refer to overall characteristics of the Cordyceps group of insect-inhabiting fungi. When the name Cordyceps sinensis was used in the research articles referred to in this chapter, that earlier name has been converted in this chapter to the currently correct name, Ophiocordyceps sinensis. When the species discussed in the research was not clearly identified, or when an unidentified anamorph species was used, the term “Cordyceps” is used here. When other species are mentioned, those correct species names are used, such as the name Cordyceps militaris, which is a species used commonly in commercial trade today. The author’s intent was to clarify the names used while accurately reporting the data represented in the research articles referred to. It is my sincere hope we have accomplished this.
3.1 Introduction
Imagine a mushroom that only grows from the head of a buried caterpillar high in the rugged mountains of Tibet, only above 3000 m elevation. This is one of the most remote, inaccessible, and hostile environments on the planet, one not normally associated with an environment suitable for mushroom hunting. This is the natural habitat of the famed Cordyceps mushroom. In use for many centuries as a precious medicine in Chinese and Tibetan medicine practices, Cordyceps is and always has been one of the rarest and most mysterious of mushrooms. It was long thought to be a nearly mythical creature that was able to change from a plant in the summer into a worm in the winter and back again when the snow cleared and the weather warmed. The famous Tibetan Cordyceps, which today is properly named Ophiocordyceps sinensis, is an ascomycetes mushroom that grows only on the dead subterranean larva of several species of moths, known as Himalayan ghost moths (around 30 species of the genus Thitarodes) (Fig. 3.1a–e). There are a number of species of this group of entomogenous (insect-inhabiting) fungi used in medicine today, which overall group is known broadly as Cordyceps sensu lato – and this group of fungi is typified by the most well-known and historically important species, Ophiocordyceps sinensis. This name has only been in use since about 2007 and was formerly known as Cordyceps sinensis, which is still the most commonly used name in medicine and by most practitioners and consumers other than professional mycologists. These fungal species have been and still are important medicines in the traditional medical practices of Asia. They are also species of great economic importance in the regions where they grow. O. sinensis is known regionally by many names dōng chóng xià căo (冬虫夏草) in Chinese, the Chinese caterpillar fungus in English, tochukaso in Japanese (translation into Japanese of the Chinese characters 冬虫夏草), known as yartsa gunbu (
) in Tibetan, and yarsagumba (यार्चागुन्बू) in Nepali. The term Cordyceps sensu lato (Cordyceps s.lat.) refers to and includes the entire broader grouping of ascomycetes fungi found growing on insect larvae and mature insects and in some cases found growing on the underground sporocarps of truffles of the genus Elaphomyces. Most of the species referred to as Cordyceps s.lat. belong to one of four genera: Cordyceps, Ophiocordyceps, Elaphocordyceps, and Metacordyceps. While Cordyceps s.lat. has been medicinally used for centuries, in-depth research into this group of fungi is all fairly recent. It was not until as late as 2007 that a full study was made of the phylogeny of Cordyceps s.lat., showing the relationship between the various genera and species (Sung et al. 2007). By far, the most well-known and most economically important species, as well as the most well-studied species of this group of fungi, is Ophiocordyceps sinensis, which has a long history of use as a rare and exotic medicinal fungus of great importance in TCM. While it is unclear from the written record exactly how long this has been used medicinally, we know that it has been regarded as one of the cornerstones of Tibetan and Chinese medicine for a very long time, at least since the fifteenth century (Boesi and Cardi 2005). O. sinensis is a medicinal herb that has long been believed to have a wide range of medicinal effects (Bensky et al. 2004; Zhu et al. 1998), many of which modern science has confirmed to be true. Up until very recently, the use of O. sinensis was restricted almost exclusively to the richer population in very limited areas of Asia due to its cost and rarity, but within the last 20 or 30 years, this medicinal fungus has become well known in Western countries. With all the claims of the wide-ranging therapeutic effects of O. sinensis, much research effort has been devoted to this group of fungi in recent years. Starting around 1980, modern scientific methods have been applied to investigating the wide range of claimed therapeutic effects in an attempt to validate what TCM practitioners have observed for centuries (Holliday and Cleaver 2008). While O. sinensis was the earliest used and is the most well known of the medicinal fungi of the group Cordyceps s.lat., in recent years many other fungal species from this broad group have been brought into medicinal usage, mainly through commercial cultivation, as other species of Cordyceps s.lat. are far easier to grow in laboratory conditions than O. sinensis. As science and technology move ahead, we are bound to see more and more Cordyceps products brought into the herbal medicine market and perhaps used as pharmaceutical raw material, so while this chapter will focus mainly on O. sinensis, the other fungi of the broader grouping Cordyceps s.lat. will be discussed as well.

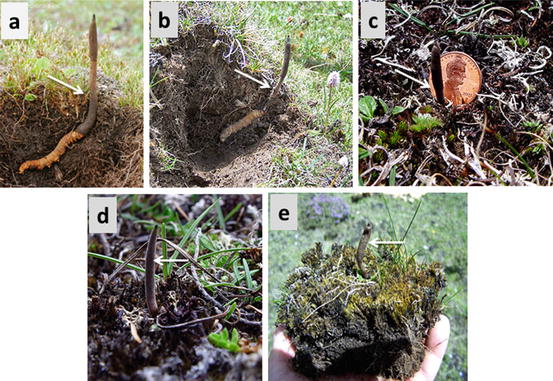
Fig. 3.1
(a) Ophiocordyceps sinensis at 5600 m elevation, Tibet. (b) Ophiocordyceps sinensis at 4800 m elevation – Tibet. (c) Ophiocordyceps sinensis with American penny for size reference. (d) Ophiocordyceps sinensis found growing at 6000 m Serkim La pass, Tibet, June 2006. (e) Tibetan Ophiocordyceps sinensis from Mila, Tibet, 2006 (Arrows showing the Cordyceps)
3.2 Ophiocordyceps sinensis: The Prototypical Cordyceps Species
O. sinensis is the species used for centuries in TCM and the one named in all the earlier texts. This is not simply a mushroom, but rather it is a complex consisting of the mushroom and the attached insect larvae, both of which are consumed together for their medicinal properties (Fig. 3.2). In fact, the mushroom portion alone is not considered to have any medicinal properties by itself; rather the caterpillar portion is the part that contains the majority of active ingredients. Recent studies by this author have confirmed this to be true, because while the mummified larvae may still look like a caterpillar, in fact, the only part of the original larvae remaining is the outermost chitinous shell, with the rest of the caterpillar having been consumed and replaced with mycelium from the fungus. This outer chitinous larval shell then acts as a reservoir, which contains all the fungal extracellular metabolites. These are the compounds that the fungus excretes out through the cell wall as it grows, in order to digest its food source in place, to act as transport molecules to bring the nutrients back across the cell wall, to stun or kill bacteria and other fungi (the antibiotic compounds), and to give itself an advantage in the food chain, and undoubtedly perform other functions as well. While it is true that the cell wall and cytoplasm of the fungus do contain some medicinal properties, in fungal metabolism, it is the extracellular compounds that contain the majority of the biologically active compounds. If we consider the classic fungal fermentation process – beer or wine production – it is easy to understand why this is the case. In making wine, for example, a substrate (fruit juice) is first sterilized and then inoculated with the target fungal species (the yeast Saccharomyces cerveza in the case of wine), and over the next few weeks as the fungus bioconverts the substrate into fungal tissue, the biological compounds of interest, things like the color, the flavor, and the alcohol, are not retained within the cell boundary, but rather they are excreted into the environment, into the liquid substrate which the fungus is growing in. The resulting wine is this mixture of the extracellular compounds and the remaining substrate. The wine is not the cell mass that collects at the bottom of the tank. This concept of extracellular activity is true for all fungi, Cordyceps included. Since fungal cells have no mouth with which to ingest their nutrients for growth, nearly all of their interactions with the surrounding environment take place by passing through the cell wall, with certain compounds traveling out from the cell and other nutrients passing the other way back into the cell. Understanding this concept has led to great advances in the cultivation process for medicinally important fungi today. Some medicinal mushrooms are cultivated in a liquid media, where the cells are grown in a liquid media, either to capture the compounds within the cells or to extract target compounds from the residual liquid. Other medicinal mushrooms are cultivated in a solid media, termed solid-state fermentation, where the entire suite of growth compounds and secondary metabolites are captured together by harvesting the entire mass of fungal tissue and residual substrate mass together. When grown in this manner, the commercial products are termed “Full Spectrum Mycoproducts” as they contain everything that was produced through the entire fungal life cycle. While the liquid fermentation process is great for producing specific isolated compounds, liquid fermentation cannot match the full profile of chemical complexity such as is found in the entire O. sinensis mushroom and insect complex. Solid-state fermentation can much more closely match the entire chemical complexity, often producing nearly an identical analytical signature as the wild variety, since both the cellular and the extracellular compounds produced by the fungus throughout the entire growth cycle are captured and harvested together (Fig. 3.3).

Fig. 3.2
Two types of Ophiocordyceps sinensis from Tibet

Fig. 3.3
HPLC comparison of chemistry of solid fermentation of O. sinensis and liquid fermentation of H. sinensis
3.3 Historical Use of O. sinensis and Other Cordyceps spp.
The first written record believed to likely refer to O. sinensis comes from China in the year AD 620, at the time of the Tang Dynasty (Bensky et al. 2004). However, this record is not entirely clear and does not positively refer to Cordyceps, although many researchers believe that to be the case. The first written record that can be confirmed as a reference to the use of O. sinensis in medicine appeared in the Tibetan text Man ngag bye ba ring bsrel (Oral Instructions on a Myriad of Medicines) written in the fifteenth century by Zurkhar Nyamnyi Dorje [1439–1475]. The first confirmed written reference of its use in TCM comes from Wang Ang’s 1694 compendium of materia medica, Ben Cao Bei Yao (Winkler 2008), and it was again mentioned in Wu Yiluo’s Ben Cao Cong Xin (New Compilation of Materia Medica) written in the year 1757, during the Qing Dynasty (Zhu et al. 1998; Halpern 2007). These early writings all speak of a creature whose annual existence included a transformation from animal to plant during the summer and then again from a plant back into an animal during the winter (Bensky et al. 2004). This belief in the transformation leads to the common Tibetan name, yartsa gunbu, which translates literally as “winter worm, summer grass.” This is also the literal translation of the common Chinese name, dōng chóng xià căo.
The species referred to in these early texts were all O. sinensis, as this is the only endemic Asian species of insect-inhabiting fungus that was known to be traditionally used in Asian medicine and one of only two species of Cordyceps with any known historical or traditional medicinal usage. The only other Cordyceps species known with a history of traditional use is Cordyceps robertsii, a species closely related to O. sinensis (Sung et al. 2007) and found only in New Zealand and traditionally used for tattooing. C. robertsii (Fig. 3.4) has been used by the Maori people to make the tattoo ink favored by traditional people in New Zealand, by burning the fungus and caterpillar complex to a fine ash and mixing the ash with oil or water. It was also used to prevent infection in the fresh tattoo by mashing the fresh fruit body/caterpillar complex and mixing with water and then applying this paste over the site of the fresh tattoo (Relph 1991; also the result of author’s discussion with various New Zealand Maori historians and elders). It is interesting to note that O. sinensis and C. robertsii are the closest relatives on the phylogenetic tree as laid out in the phylogenetic study of Cordyceps s.lat. by Sung et al. (2007). Humans tend to be very good observers of nature, and when separate peoples in different parts of the world are found to use closely related mushroom species for similar or exact purposes, it supports the idea that there is some fact underlying the belief.
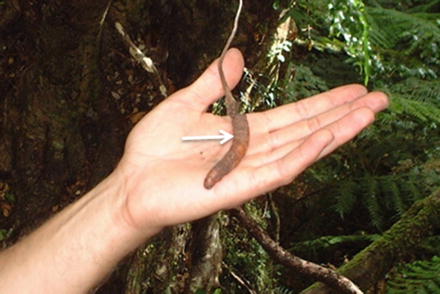
Fig. 3.4
Cordyceps robertsii – known in New Zealand as “awheto”
3.4 The Collection and Economic Value of Wild O. sinensis
In the wild, O. sinensis has a very small natural range. The natural habitat is restricted to high altitudes on the Himalayan Plateau above an elevation of approximately 3000 m. The primary collecting region is the Naqu district of Tibet, with an annual harvest reported at 7000 kg (Chen et al. 2000). The total O. sinensis harvest for the entire Tibet Autonomous Region (TAR) is estimated to be about 50 tons annually, with a total harvest of about 60 tons worldwide (Boesi and Cardi 2009; Winkler 2008). It is also collected in smaller volumes throughout the rest of Tibet, in the Gansu, Qinghai, Sichuan, and Yunnan provinces of China, in the countries of Nepal and Bhutan, and a small region in northernmost India in Sikkim state. The value of wild-collected O. sinensis is literally higher than gold. The price in Beijing in recent years has been as high as $112,000 USD per kilogram (Lo et al. 2013). The annual harvest in Tibet, which takes place over just a 4- to 6-week period in May and June, is estimated to add approximately $225 million USD to the GDP of the TAR, accounting for fully 40% of the total rural cash income of Tibet. The collection of O. sinensis is understandably very popular throughout the TAR, where the average Tibetan nomad family earns the largest portion of its annual income from the collection of O. sinensis, representing up to 80–90% of the total annual income for many rural nomadic families (Winkler 2010). While the importance of the O. sinensis harvest to the economy of the TAR cannot be overstated, it is unknown how sustainable this harvest will prove to be, due to environmental destruction from overharvesting and the dearth of regulations controlling the harvest in a sustainable manner. This fantastic financial growth in TAR triggered by Cordyceps has led to other environmental problems as well, even outside of TAR itself. In Tibetan culture, there is a long tradition that tiger skins represent personal wealth and power. Since the trade in Cordyceps is an entirely cash-based economy, the ready availability of large quantities of unreported and untaxed cash from the trade has dramatically increased demand in TAR for tiger skins, which are being smuggled into TAR from India, despite strong international restrictions on such trade. This increased demand for tiger skins in Tibet is leading to a serious reduction in populations of tigers in India, perhaps presaging their eventual extinction (Moyl 2009; Wright 2012).
Due to the elevation, the remote location, the small geographical area where it is found and the subsequent difficulty in harvesting O. sinensis, it is understandable why it has always been one of the most expensive medicinal substances known. Historically, its high price meant its use was restricted almost exclusively the Emperor’s court and others of the ranking Chinese nobility. Its high price tended to place it beyond the reach of the average Chinese and Tibetan population. Despite its cost and rarity, the unprecedented list of medicinal uses for O. sinensis made it a valued staple of TCM. In modern years with the increasing economic status and a growing middle class in Asia, O. sinensis has become more widely used. It is known today by most people in Asia and considered to be a favored medicine. It is no longer just in Asia that it is widely prescribed by TCM practitioners but more so throughout the world today. Since the opening of China to trade with the west in 1972, TCM was brought to the attention of people in the Western countries. This growing interest in TCM in the West has brought O. sinensis to the attention of people in more affluent areas, such as North America and Europe. In a study published in 2013, the annual harvest was valued between 5 billion and 11 billion US dollars (Shrestha and Bawa 2013). This growing popularity throughout the world has resulted in increased demand and increasing supply, both of which have created multiple problems in recent years: the ever-increasing price has meant the number of collectors is increasing each year, which is resulting in the species being dramatically overharvested, with more than a 50% reduction in the quantity harvested, just between the years 2009 and 2011 (Shrestha and Bawa 2013). This overharvesting is considered to be the main reason for the declining yields every year. This is why the future remains uncertain as to the continuing availability of wild-collected O. sinensis. These reduced yields and inflated prices have led to many counterfeit and adulterated products reaching the consumer in recent years (Holliday and Cleaver 2008), although the development and application of new analytical techniques and a more discerning consumer are reducing these number of false Cordyceps products as time goes on.
3.5 Cordyceps Diversity and Artificial Cultivation
There are more than 600 species of Cordyceps s.lat. known to science, and many more species are known to exist that have not been described to science or named yet. In fact, there are Cordyceps species found on all continents except Antarctica and in most of the various climatic zones and habitats. This author alone has found more than 1000 types of Cordyceps in the Tingo Maria Valley in Peru, most of which are assumed to be new, as yet undescribed species. Tingo Maria Valley was used as an experimental area by the US Drug Enforcement Administration to aerially apply spores from a plant pathogen fungus, the species Fusarium oxysporum, in an effort to eradicate the coca crop and reduce Peru’s involvement in the international cocaine trade. It is unknown whether this experiment was a success in reducing the number of coca bushes, but one thing became clear within a short time after application of the spores: the F. oxysporum spores certainly increased the number of Cordyceps fruiting, with a multitude of species growing in virtually every family of insects and arachnids of the Tingo Maria Valley (Fig. 3.5a–e).
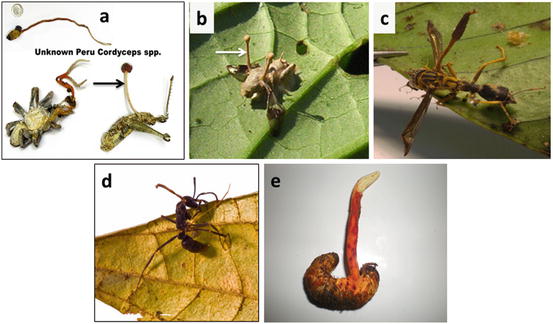
Fig. 3.5
(a) Cordyceps spp. from Tingo Maria Valley, Peru. (b) Another unknown species from Peru. (c) Cordyceps fruit body growing from a wasp in Tingo Maria Valley, Peru. (d) A Peruvian ant species of Cordyceps. (e) The largest Cordyceps species found in Peru so far – up to 400 mm in length
The sheer number of specimens collected there has limited the description and naming of these specimens, although the work is ongoing and will be for years to come. These Cordyceps species all occur parasitically or commensally (perhaps in some cases symbiotically, as with termites in Africa) with a wide range of insect and arachnid hosts (Holliday and Cleaver 2008; Halpern 2007; Sung et al. 2007), with Elaphocordyceps species inhabiting truffles of the genus Elaphomycetes. Due to the ever-increasing rarity and high prices of wild-collected O. sinensis, much effort has been made to artificially cultivate O. sinensis or related species, many of which have similar chemical makeup and medicinal properties. In fact, O. sinensis has proven to be very difficult to cultivate in artificial conditions, perhaps due to the vast range of associated fungal species that are known to coinhabit the insect host from which the O. sinensis fruiting body erupts. Using modern high-throughput DNA sequencing, more than 600 species of fungi belonging to at least 65 genera have been found to coinhabit wild O. sinensis (Xia et al. 2016). It is unknown how these different fungi interact with the O. sinensis or with the insect host. Many researchers believe there is a symbiotic relationship between some or all of these fungi, and the cocultivation of many different species may be required to replicate the complex conditions required for fruiting the O. sinensis fungus. It also appears from this author’s work in cultivating O. sinensis and other Cordyceps species that several different species need to be cocultivated together in order to replicate the complex chemical signature of naturally occurring O. sinensis. In other words, even though the known medicinal species is O. sinensis, from a consideration of the active medicinal compounds present, things are not as simple as they seem. The myriad species complex found in the natural O. sinensis each adds their own unique compounds to the mix, and no single one of the species represents all of the known medicinal or chemical properties when they are cultivated alone as single species. With the escalating price of natural O. sinensis, as early as the mid-1980s, the majority of Cordyceps available in the world’s marketplace was artificially cultivated (Mizuno 1999). In 1985, the first officially approved drug derived from O. sinensis cultivation was brought to market in China, under the name Jin Shui Bao. Although today it is known through DNA sequencing that Jin Shui Bao is not actually a Cordyceps species, certainly not O. sinensis, rather it is another of the commensal ascomycetes species found cohabiting O. sinensis, a species known as Paecilomyces hepiali. This species of fungus is fairly easy to cultivate and is usually passed off in the dietary supplement market under the name Cordyceps sinensis CS-4. When this species was first isolated from wild O. sinensis in the early 1980s, it was assumed to be the anamorph of O. sinensis. Later as DNA sequencing and molecular identification techniques became more widely applied, it was found to be P. hepiali rather than the O. sinensis anamorph as had been assumed (Halpern 2007). This has led to much confusion, as the original research from the 1980s refer to this as Cordyceps sinensis, so much of the medicinal properties thought to be from that species are now known to be from P. hepiali instead. Even though this misidentification has now been corrected, many commercial companies still sell this under the name “Cordyceps CS-4.” Many companies now produce artificially cultivated Cordyceps products, both from the mycelium and also from the fruit bodies of a few Cordyceps species, most notably Cordyceps militaris, a species that fruits readily in culture. Cordyceps militaris is usually passed off today as a direct analog of natural O. sinensis, especially in Asia, even though it is a different genus and species with no traditional medicinal usage. There are a few large biotech companies today, such as Aloha Medicinals in the United States and Chung Jing Biotechnology in Taiwan, which have invested huge sums into research in methods for commercially cultivating Cordyceps, with the result that today those companies specializing in cultivating Cordyceps produce herbal supplements with a virtually identical chemical profile to wild-collected O. sinensis. However, the increase in suppliers has also given rise to variations in purity and quality, creating a situation in which there are a large number of counterfeit and adulterated products being sold (Halpern 2007). However, that situation is changing as time progresses, as there have been new methods recently introduced for assaying the quality and purity of Cordyceps products, mainly by looking at the unique nucleosides found only in Cordyceps (Holliday et al. 2004; Yu et al. 2006; Li et al. 2006). The large variations in quality found in Cordyceps products cultivated under different methods and culture conditions by different companies lead many consumers to believe wild-collected O. sinensis is medicinally more potent than the cultivated type. But with continuing advances in our understanding of both the chemistry and the mechanisms of action for Cordyceps’ medicinal activity, along with the great advances we have seen in biotechnology practices since the beginning of the twenty-first century, companies today are able to cultivate Cordyceps under modified culture parameters and by using specially selected substrates, which are chosen specifically for the optimized production of the targeted medicinal compounds sought (Cleaver et al. 2008). This is leading to an ever-increasing quality of commercial Cordyceps products available on the market. With habitat destruction and overharvesting, wild O. sinensis is becoming more and more scarce and faces the real risk of becoming extinct in the near future, but fortunately, the cultivated variety is becoming ever closer to the potency and wide-ranging effects of the original wild type. As research continues to unfold the secrets of Cordyceps, it is allowing cultivators new targets to aim for, and new levels of quality and consistency are evolving in the commercial Cordyceps market every day.
One issue that has commercial cultivators looking for answers today is the fact that because O. sinensis cannot be readily fruited in culture, the commercial cultivation industry is limited to fermentation technology of the anamorphic stage only – the asexual mycelial growth. This mycelium material is commercially produced and marketed as a substitute for the much more expensive wild-collected variety. This situation is fine in most respects, as the mycelial products can have an almost identical analytical signature to the wild-collected O. sinensis fruit body and insect host complex. And because commercial cultivation is typically performed at large scale, the products are far more affordable and available to a much larger market sector than wild-collected types. At least this quality issue is true when the products are cultivated by the larger biotech firms. Unfortunately, the high price and wide demand for these products have led some unscrupulous manufacturers to put products on the market that sometimes do not contain any Cordyceps at all. This tends to be more common in Asian products and low-cost products sold in Africa, rather than in European or American products.
Over the last four decades, there have been 22 different species from 13 different genera identified as the anamorph, or asexual stage, of O. sinensis in the scientific literature. In 2001, a group from China led by Yue-Qin Chen published a paper purporting to resolve this question, showing their research group had determined through modern DNA sequencing methods Hirsutella sinensis be the true anamorph for O. sinensis (Chen et al. 2001). According to decisions made at the Nomenclature Section meeting of the International Botanical Congress in Melbourne, Australia, in July 2011, these new naming regulations allowed each fungus to have only one name (Hawksworth 2011). These regulations took effect on January 1, 2013, and since then the anamorph name Hirsutella sinensis was dropped by the International Code of Nomenclature for algae, fungi, and plants (formerly called the International Code of Botanical Nomenclature) (McNeill and Turland 2011). The name Hirsutella sinensis is no longer used, and the currently correct naming for both the anamorph and teleomorph stages of O. sinensis are now both the same – O. sinensis.
It would seem that with the identification of the true anamorph for O. sinensis, it would be relatively simple for cultivators to produce products identical to the wild-type fungus. But this has definitely proven not to be the case. As mentioned earlier, there are many fungi cohabiting and living commensally in the insect host that forms one-half of the O. sinensis fungal/insect complex. In fact, when examined closely, the fruiting body and larval remains most closely resemble a colony organism, much the way a coral reef is a colony – made up of many very diverse species. Many to most of these separate species are dependent on some other species for their very survival, and they all unquestionably add their own unique secondary metabolites into the complex. With more than 600 individual species of fungi shown to coinhabit the Thitarodes larvae and O. sinensis fungal complex, we can assume all of these fungi add something to the complex chemistry found in the wild O. sinensis. It is interesting that when Hirsutella sinensis is cultivated alone as a single species, the resulting chemistry, the analytical profile, does not look very much like O. sinensis at all (Fig. 3.3). With such a dissimilar chemical profile, the cultivated H. sinensis does not have the same medicinal properties as the wild fungus. The approach that has been taken by some of the more sophisticated biotech cultivators of O. sinensis substitutes today is that rather than strictly matching the DNA of the wild type, they match the O. sinensis chemical profile as closely as possible, thereby replicating the entire complex analytical signature and the medicinal properties of wild-collected O. sinensis. This often requires the blending of several cultivated anamorph species, usually including both Hirsutella spp. and Tolypocladium spp., and sometimes adding Paecilomyces spp. into the mix. This approach, along with carefully chosen substrates and culture conditions (Cleaver et al. 2008), results in very high-quality commercial Cordyceps products, which have the same characteristics, chemical profile, and medicinal activity as the wild-collected O. sinensis, at a mere fraction of the price.
Several other species of Cordyceps are being cultivated today as alternatives to O. sinensis, including large quantities of Cordyceps militaris. While C. militaris has no historical usage in traditional medicine, it shares many of the chemical constituents of O. sinensis and is a species that is much easier and cheaper to cultivate, making it a ready substitute for natural Cordyceps. Today, C. militaris has become a readily available substitute for O. sinensis and has many of the same properties and medicinal qualities (Das et al. 2010). Cordyceps japonica (Park et al. 2002) and Cordyceps cicadae (Hsu et al. 2015) fall into this same category and are currently being marketed as cultivated substitutes for O. sinensis.
3.6 Traditional Medicinal Usage of Cordyceps
The range of therapeutic uses claimed for Cordyceps is quite broad, although many of them have yet to be investigated using the modern gold standard of double-blind, placebo-controlled clinical trials. O. sinensis has been used in TCM to treat quite a wide range of medical conditions, including respiration and pulmonary diseases, cardiovascular diseases, renal and liver disease, hypo-sexuality, hyperlipidemia, and many age-related disorders. It is also widely used for boosting weak immune systems and other immune disorders and as an adjunct to the modern cancer treatments of chemotherapy, radiation, and surgery (Bensky et al. 2004). O. sinensis is believed by many, particularly those people living in its place of origin, Tibet, to be a remedy for altitude sickness and all manners of general weakness and fatigue. It is often used as a general tonic for increased energy or taken while recovering from any serious illness to give strength, increased vigor, and help speed recovery. O. sinensis also has a strong reputation as a treatment for impotence and is often referred to in Asia as “Himalayan Viagra.” The treatment of impotence is perhaps the single largest market for wild-collected O. sinensis today, with an annual market estimated over a billion US dollars in China alone. There is some basis for this belief, as early clinical trials showed O. sinensis to increase sexual function in both men and women (Zhu et al. 1998). However, these trials were poorly designed with only a small patient base and would not stand up to today’s more rigorous clinical trial standards. O. sinensis is often prescribed in TCM for the elderly to ease general aches and pains. TCM practitioners also recommend the regular use of O. sinensis to strengthen the body’s immune system and resistance to illness, such as colds and flu, and to generally improve the well-being and health of the patient. O. sinensis has historically been used as almost a catchall medication, seemingly prescribed for so many different conditions that it seems like the medical “magic bullet,” good for whatever ails the patient. Modern science has proven some of these uses to be valid and is still looking for evidence for some other traditional uses.
3.7 The Bioactive Chemistry of Cordyceps
O. sinensis and other Cordyceps, although they are not normally considered as food, contain a broad range of nutrient compounds. Cordyceps contains all 18 of the essential amino acids. The content of amino acids tends to be quite high, generally in the range of 5–10%. The highest contents are glutamate, arginine, aspartic acid, tryptophan, and tyrosine (Zhou et al. 2009). Also found are vitamins E and K and the water-soluble B vitamins, including B1, B2, and B12. In addition, Cordyceps contains many mono-, di-, and oligosaccharide sugars and contains very unique complex polysaccharides of both the alpha-bound and beta-bound conformation, even some polysaccharides with both beta- and alpha-bonds found in the same molecule, containing both five and six carbon sugars, which make them true heteropolysaccharides, proteins, sterols, many different nucleosides, including some unique nucleosides found nowhere else in nature, and a wide range of minerals, perhaps reflecting the rich mountain soil in which it grows, including K, Na, Ca, Mg, Fe, Cu, Mn, Zn, Pi, Se, Al, Si, Ni, Sr, Ti, Cr, Ga, V, and Zr (Holliday and Cleaver 2008; Mizuno 1999).
3.7.1 Polysaccharides
O. sinensis contains a large concentration and variety of polysaccharides, which can be in the range of 10–20% of the total weight of wild-collected specimens and typically up to 50% or even higher in Cordyceps specially cultivated by solid-state fermentation methods to enhance the production of these unique polysaccharides. The structural cell wall polysaccharides and the exopolysaccharides excreted into the culture medium or into the environment in wild specimens are considered to be the main medicinally active classes of compounds from Cordyceps. These polysaccharides are present in the fruiting bodies, the mummified larva, and the mycelium of both solid fermentation and submerged cultures, and the exopolysaccharides are found in the culture broth of liquid cultivated and excreted into the substrate in solid-state fermentation (Zhou et al. 2009). Four different beta-D glucan exopolysaccharide structures exopolysaccharides from C. militaris with different molecular masses ranging from 50 to 2260 kDa were found in C. militaris cultivated by liquid fermentation (Kim et al. 2003). In the case of O. sinensis, most of the heteropolysaccharides contained mannose, galactose, and glucose, with mannose at higher levels and with smaller amounts of the five carbon sugars arabinose, rhamnose, and xylose, respectively. The average molecular mass of O. sinensis polysaccharides varies between 7 and 200 kDa, while the C. militaris polysaccharides consisted mostly of glucose, galactose, and mannose with only traces of rhamnose and xylose and have an average molecular weight of approximately 60 kDa (Zhong et al. 2009). The polysaccharides of Cordyceps are very difficult to analyze and elucidate due to their complex tertiary structure, which is primarily a triple right-hand helix conformation with differing lengths and ratios of pentose and hexose side chains and only recently have analytical methods been developed to accurately purify and assay the various polysaccharide fractions (Danielson et al. 2010; Megazyme 2008). The attempt to fully elucidate the polysaccharides from Cordyceps is an ongoing research area being pursued by many scientists around the world. Much has yet to be learned regarding the tertiary structures of these polysaccharides, their binding to particular receptor sites on the cells, and the subsequent biological actions triggered in the body.
3.7.2 Proteins and Other Nitrogenous Compounds
Cordyceps contain proteins, peptides, polypeptides, polyamines, all the essential amino acids, and a number of common and uncommon cyclic dipeptides, including cyclo-[Gly-Pro], cyclo-[Leu-Pro], cyclo-[Val- Pro], cyclo-[Ala-Leu], cyclo-[Ala-Val], and cyclo-[Thr- Leu]. Cordyceps also contains small amounts of polyamines, such as 1,3-diamino propane, cadaverine, spermidine, spermine, and putrescine (Mizuno 1999).
Many free nucleosides have been found in Cordyceps, including uridine, several unique deoxyuridines, adenosine, dideoxyadenosine, hydroxyethyl adenosine, cordycepin [3′deoxyadenosine], cordycepin triphosphate, guanidine, deoxyguanidine, and other altered and deoxygenated nucleosides, many of which are found nowhere else in nature (Fig. 3.6) (Yang et al. 2007).
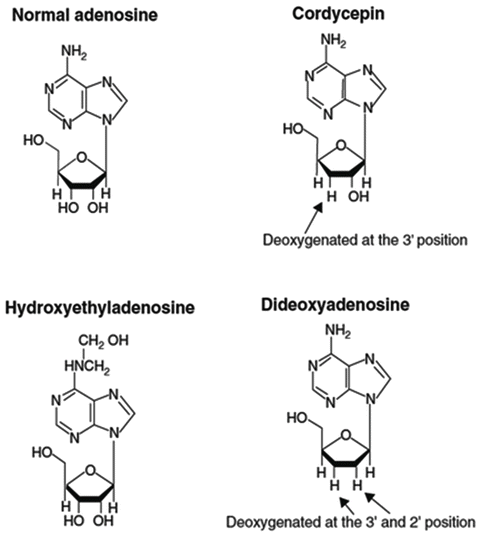
Fig. 3.6
Some of the unique nucleosides found in Cordyceps
Many of the altered nucleosides, such as cordycepin, have been shown to have potent antiviral effects, including effects against the HIV virus (Adotey et al. 2011). Cordycepin is found in several of the Cordyceps species, in lesser quantities in O. sinensis, and larger quantities in C. militaris. Cordycepin has a unique way of halting HIV (and other viral) replication: cordycepin is an inhibitor of ribonucleoside triphosphate (rNTP). Macrophages contain high levels of rNTP, which is used in cells in a variety of ways. HIV uses primarily rNTP to replicate inside macrophages but not in CD4+ cells. By inhibiting the rNTP, cordycepin effectively inhibits the replication of the virus, at least in the macrophages (Kennedy et al. 2010). This is just one of the several mechanisms by which cordycepin is thought to exert its antiviral effects. Sugar-binding proteins named lectins were isolated from C. militaris. N-terminal amino acid sequence differed greatly from other lectins (Yue et al. 2013). Production of the nonribosomal peptides cicapeptins I and II from C. heteropoda was reported. These are compounds unique in their structures that have been found only in one Cordyceps species so far, which display antibacterial and antifungal activity (Krasnoff et al. 2005).
The total amount of amino acids in cultivated Cordyceps products can be up to about 10% by weight. In O. sinensis, the three principal amino acids in both the mycelium-mummified larvae and the fungal fruiting body are glutamic acid, aspartic acid, and arginine, and their contents are in the range of 1.5% up to 2.6% (Hsu et al. 2002). Cordyceps is often used to provide mild sedation, and in one trial, a mixture of 18 synthetic amino acids combined to mimic the amino acid composition in natural O. sinensis showed the same sedative action as natural O. sinensis, confirming this medicinal activity (Zhang et al. 1990).
A number of unique peptides and cyclodipeptides have been found in Cordyceps. One is the cyclodipeptide named cordycedipeptide A, which is a compound isolated from the culture liquid of liquid fermentation-cultivated O. sinensis. This compound showed good antitumor effect, showing cytotoxicity when tried against several cancer cell lines, including L-929, A375, and HeLa cells (Jia et al. 2005; Mang et al. 2015).
Another interesting peptide that was isolated from O. sinensis was named cordymin. Cordymin was shown in animal trials to have a protective effect in the ischemic rat brain by inhibiting inflammation. If this animal data translates to humans, this compound could prove to become a valuable medication for the prevention or limiting of neurological damage caused by cerebral ischemia-reperfusion injury in stroke patients (Wang et al. 2012b).
A unique and atypical amino acid known as myriocin has been isolated from O. sinensis and has been found in several other fungi as well. Myriocin is a potent immunosuppressant agent, up to 100 times more potent than the standard against which other immunosuppressants are measured, cyclosporine. Myriocin, which also has antibiotic properties and is known as antibiotic ISP-1, is a new type of immune inhibitor. It also very potently inhibits the synthesis of sphingosine, which forms a primary part of sphingolipids. These are a type of cell membrane lipid that includes sphingomyelin, the material covering nerve fibers in humans. Myriocin is used today primarily as a research tool to deplete cells of sphingolipids, although it shows potential for other uses in medicine as well (Zhao et al. 2014; Paterson. 2008).
O. sinensis has long been used as an anti-inflammatory medication. The discovery of a group of uniquely altered adenosine-type compounds named cordysinins in Cordyceps has proven this usage to be scientifically valid. Five cordysinins, named cordysinins A–E, have been isolated from the mycelia of O. sinensis. These compounds have been shown to have potent anti-inflammatory activities (Lo et al. 2013; Shrestha et al. 2014).
3.7.3 Sterols
A number of sterol compounds have been found in Cordyceps, including the sterol common to all fungi, ergosterol. Ergosterol is important to human health in that it is the precursor of vitamin D2
. When ergosterol is exposed to ultraviolet light (as in sunlight), a photochemical reaction is triggered that converts ergosterol into vitamin D2, the biologically active form of vitamin D in the human body. Other sterols have been found in Cordyceps as well, including δ-3 ergosterol, ergosterol peroxide, 3-sitosterol, daucosterol, and campesterol (Zhu et al. 1998). Another sterol compound named H1-A was found in O. sinensis that has shown to be effective in the treatment of autoimmune disorders, a great challenge to medicine using the current drugs available today, and an area worthy of further research (Yang et al. 1999).
3.7.4 Other Constituents
Twenty-eight saturated and unsaturated fatty acids with the function of decreasing blood lipids have been isolated from O. sinensis. The unsaturated fatty acid content includes Cl6:1, Cl7:1, Cl8:l, and Cl8:2 (Zhou et al. 2009). Polar compounds of C. sinensis extracts include many alcohols and aldehydes (Bensky et al. 2004). Particularly interesting is the range of polycyclic aromatic hydrocarbons produced by some O. sinensis strains, called PAH compounds, which were found to react with the polypropylene used in common mushroom culture bags, resulting in the production of by-products toxic to O. sinensis and stunting growth as time progresses, limiting the cultivation potential of strains producing these PAHs to glass or metal culture containers (Holliday et al. 2004). Various immune-suppressive compounds have been found in Cordyceps, including cyclosporine from Cordyceps subsessilis, which is most commonly produced through the cultivation of the Cordyceps subsessilus anamorph (Tolypocladium inflatum) (Segelken 2002). Other immune-suppressive compounds have been found in Isaria sinclairii, another entomopathic species that is closely related to Cordyceps (Halpern 2007).
3.7.4.1 Mannitol
O. sinensis contains a high percentage of D-mannitol, up to 10% in wild specimens of O. sinensis and up to 14% in cultivated varieties. D-mannitol is the so-called cordycepic acid in Chinese. The name cordycepic acid was thought to be first used by the mycologist G.H. Cunningham around 1951, referring to a slightly acidic compound in Cordyceps militaris that he assumed was unique to Cordyceps. It was first isolated and purified from O. sinensis in 1957 (Lin and Li 2011). Further research into the chemistry of this compound determined it was not unique to Cordyceps but was nothing other than the common sugar alcohol D-mannitol. As such, the name cordycepic acid has been considered obsolete since the late 1950s. This compound is not unique to Cordyceps, and in fact, D-mannitol is found in much higher concentrations in cranberries than it is in any of the Cordyceps species. However, this term is still widely used in the commercial market as a “verification of authenticity” and a “measure of potency,” with manufacturers claiming their products to contain this or that percentage of cordycepic acid. This is an unsubstantiated marketing claim used by unscrupulous marketers to confuse the nonscientific public into thinking their products must be better than another company’s products because of the “high levels of cordycepic acid” they contain. The term cordycepic acid cannot be found in any modern chemical formularies though, and the correct term D-mannitol should be used in its place. D-mannitol is found in O. sinensis, C. militaris, and many others of the Cordyceps s.lat. group.
3.8 Current Medicinal Uses for Cordyceps s.lat.
While the long history of and widespread use of Cordyceps in TCM has been discussed in the section on Traditional Medicinal Uses above, it is still a favored medicine in TCM today, seemingly gaining popularity year by year as TCM becomes more widely accepted in other areas around the world, far from its birthplace of China. Also, the increased availability through commercial cultivation has allowed its widespread use, such as was never possible in previous times. One of the most significant activities ascribed to almost all the medicinal mushrooms in recent years is their role as immunomodulators, also known as biological response modifiers (BRM) (Zhu et al. 2015; Chen and Seviour 2007; El Enshasy and Hatti-Kaul 2013). Immunomodulation is not the same as immune potentiation or immune suppression, rather it is usually defined as a concurrent bidirectional immune response, with the upregulating of some classes of the immune cell while simultaneously downregulating other cell classes. For example, in clinical trials, it was found in some autoimmune diseases; we find Cordyceps downregulates pro-inflammatory cells while upregulating anti-inflammatory cells (Halpern and Miller 2002; Zhu et al. 1998; Lu 2003). This immunomodulation effect is thought to be primarily the result of the polysaccharide component, which are primarily the cell wall structural components, found in both wild and cultivated varieties of Cordyceps. Many other medicinal activities are ascribed to Cordyceps, such as anticancer, antitumor, antimetastatic, immunomodulatory, antioxidant, anti-inflammatory, insecticidal, antimicrobial, hypolipidemic, treatments for hypolibido in both males and females, hypoglycemic, antiaging, and neuroprotective and renal protective activities (Holliday and Cleaver 2008).
3.8.1 Cancer
One of the major applications of O. sinensis and other of the Cordyceps s.lat. today are their use in cancer treatments as adjuncts to chemotherapy and radiation therapy and to speed recovery from surgery. Quite a bit of research has been done in the area, including both animal and human trials. In one trial with lung cancer patients, a commercial formulation consisting of several species of medicinal mushrooms named Immune Assist™ was used, of which O. sinensis is a major constituent. This formula and compared with an anti-side effect medication called Polyactin A™. In every side effect parameter measured, such as vomiting, hair loss, appetite, sleep time, etc., the medicinal mushroom formula outperformed the drug (Ruwei et al. 2003). In another 1 yearlong trial with this same Immune Assist™ mushroom formula conducted on dogs undergoing chemotherapy for four types of cancer, the mushroom immunomodulator formula showed very good results (Gianotti et al. 2009). The mechanism by which Cordyceps inhibits the growth of various cancer cells is thought to occur by several mechanisms of action: by upregulating the body’s own immune function; by selectively inhibiting RNA synthesis, thereby affecting protein synthesis; perhaps by restricting the growth of new blood vessels (antiangiogenesis); by inducing tumor cell apoptosis (Tuli et al. 2015; Chen et al. 2014; Li et al. 2015); and by the direct cytotoxic effect of cordycepin and other altered nucleosides being taken up by the tumor cells (Zhou et al. 2009).
Inhibiting growth of cancer cells through enhancing of immune function is usually associated with the fungal polysaccharides, especially beta-D-glucans and the unique heteropolysaccharides in Cordyceps. These are specific structures of polysaccharides, which are not found in plants or animals but are found in the cell walls of fungi and some bacteria. Consequently, when orally administered, they are recognized by the intestinal immune system – the gut-associated lymphoid tissue (GALT) – to be pathogen-associated molecular patterns, called PAMPs (Janeway 1992). PAMPs trigger strong immune responses in the gut, similar to what would occur if it was facing a massive fungal infection. This leads to a rapid increase in the number and activity of immune cells, which pass through the intestinal wall and into the bloodstream, where they circulate on a heightened state of alert and are thus available to fight other immune challenges encountered, such as the identification and destruction of aberrant cells. This heightening of nonspecific immune awareness is thought to be one of the main mechanisms by which the fungal polysaccharides work in identifying and downregulating cancer growth.
Studies have shown that there are other ways besides gut immune activation in which beta-D-glucans initiate biological response, such as by directly binding to receptors located on the surface of the immune system effector cells, such as the T cells, B cells, eosinophils, neutrophils, and monocytes, thereby setting up different intercellular activities of the immune system and leading to release of various cytokines, such as tumor necrosis factor (TNF) interleukins and interferons (Battle et al. 1998). Complement receptor type 3 (CR3) receptors, Toll-like receptors (especially TLR-2), and Dectin-1 receptors play an important role in binding and activating responses to fungal beta-D-glucans (Chen and Seviour 2007). It is most likely that other uniquely fungal polysaccharide structures bind to other cell receptor sites, which mechanisms are currently under investigation but have not been fully elucidated yet. The anticancer role of cordycepin and other Cordyceps-derived compounds has been extensively investigated in a variety of cancers, including glioma and cancers of oral, breast, lung, hepatocellular, bladder, colorectal, testicular, prostate, melanoma, and blood cell (Tuli et al. 2015; Chen et al. 2008, 2014; Li et al. 2015; Wu et al. 2007; Lee et al. 2009, 2010, 2011, 2012, 2013a, b, 2014a, b; Choi et al. 2011; Kim et al. 2011; Noh et al. 2010; Nakamura et al. 2006; Shi et al. 2008; Lu et al. 2014; Jen et al. 2010; Pan et al. 2011; Jeong et al. 2011, 2012; Yoshikawa et al. 2008, 2009; Thomadaki et al. 2008; Ko et al. 2013).
A search on Google Scholar (https://scholar.google.com) conducted on March 30, 2017, using the keywords Cordyceps + Cancer returned almost 11,000 scholarly articles on this topic. This number of published articles indicates the scope and breadth of the research being performed into cancer treatment using Cordyceps over the last 30 years. It seems that cancer treatment is one of the traditional uses for Cordyceps, which use has been well established as valid today.
3.8.2 Antiviral
In a recent murine study on infection and mortality of the H1N1 influenza virus, it was shown that an extract of C. militaris had a strong anti-influenza effect (Lee et al. 2014c).
The mechanism of action for the antiviral effect cited in this study was the increase in IL-12 production and an increase in NK cell population. Another 2007 influenza study using an acidic polysaccharide extract from C. militaris also showed potent anti-influenza activity and decreased mortality (Lee et al. 2014c). In another recent study using a protein extracted from Cordyceps sobolifera, potent HIV reverse transcriptase inhibition was noted, opening the possibility for the development of Cordyceps-based anti-HIV medications (Wang et al. 2012b). Two studies using Cordyceps and other mushroom polysaccharides in conjunction with the drug lamivudine on chronic hepatitis B patients had shown that when the mushrooms were added to the standard treatment of lamivudine, it increased treatment efficacy and decreased the time to patient’s conversion to an HBV seronegative (Li 2004; Ruwei et al. 2004). Many other studies on Cordyceps’ antiviral activity have shown Cordyceps to have potential in treating many viral infections and, as the raw material source for new antiviral drug development, validating the long-observed antiviral effects by TCM practitioners.
3.8.3 Fatigue
Of all the traditional uses that Cordyceps is noted for, perhaps the one that is best known is the relief of fatigue. There is an ancient legend told in the Himalayas, relating the way Cordyceps was originally found; it is a legend from a long time ago when the tribes’ people of Tibet and Nepal took their animals into the high mountain pastures for springtime grazing. There they would see goats and yaks grazing on some sort of a small, brown grasslike mushroom, growing from the head of a caterpillar. After eating this strange-looking creature, the animals would become frisky and start chasing the other goats and yaks around with lustful intent. This added vigor must have looked pretty attractive to those tribes’ people, so they started collecting these small mushrooms and eating them as well. They also became frisky, even a bit lustful, or so the story goes (from author’s discussions with local nomad people in Tibet).
Today, most of the wild O. sinensis collected is considered too valuable to be consumed by the rural people in its natural habitat, so all of it tends to get sold off to buyers for transport to Beijing and on to the rest of the world. It seems that modern conveniences such as motorcycles and TVs have become more valued in those collecting regions than the benefits from consuming the fungus itself. But in previous times, the people living in these harsh climate high mountains would consume Cordyceps on a regular basis. It gave them energy and offset the symptoms of altitude sickness. With Cordyceps at their disposal, they were able to trek higher into the mountains and stay there for longer periods of time. We now think that one of the reasons for this energy-boosting effect is this increase of cellular ATP (Siu et al. 2004). In addition to increasing cellular ATP, recent studies have shown that oxygen availability is facilitated from taking Cordyceps (Hirsch et al. 2017), which would also help to explain how Cordyceps assists in these high-altitude jaunts. Cordyceps is in regular use today by most high-altitude mountain climbers, and it is doubtful if Mount Everest would get nearly so many visitors if it were not for the remarkable fatigue-reducing effectiveness of Cordyceps. Cordyceps supplements are also in widespread use by professional athletes in the United States (personal communications between author and several professional NFL football players) and other amateur and professional sports as shown by the popularity of the many Cordyceps-based performance-enhancing supplements on the market today, such as the supplements Performax™ by Aloha Medicinals and Optygen™ by First Endurance Company, as well as hundreds of other Cordyceps-containing sports products as found through any search on the internet for Cordyceps performance enhancement products. One of the simplest and most reliable tests used to determine whether a compound can actually increase energy output or decrease fatigue is the mouse swim test. In this test, two groups of mice are used. One group gets the normal diet and the other group a normal diet with the addition of the test substance, Cordyceps in this case. After a period of time taking the test substance, the two groups of animals are put into a steep-sided container full of water from which they cannot escape. In that way, they are forced to swim. The time to exhaustion is measured for the two groups and compared. If the group receiving the test compound swims longer than the group on the normal diet, then it has been determined that they had increased energy output and/or decreased fatigue than the other group. Many trials of this nature have been conducted using Cordyceps, and invariably they show that the use of Cordyceps significantly increases the time to exhaustion in the test animals. An example of this test is detailed in the 1999 review article by T. Mizuno, titled “Medicinal Effects and Utilization of Cordyceps (Fr.) Link (Ascomycetes) and Isaria Fr. (Mitosporic Fungi) Chinese Caterpillar Fungi” (Mizuno 1999) (Fig. 3.7).
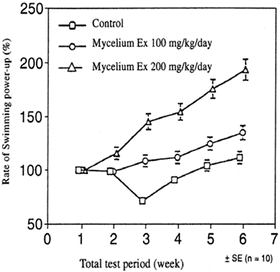
Fig. 3.7
Mouse swim test with O. sinensis extract
3.8.4 Kidney Protection and Repair
Traditional beliefs are that Cordyceps mushrooms are useful for strengthening the kidneys. Given its vast array of uses, it is interesting to note that what is being discovered today is that kidney health, perhaps more than that of any other organ, is a virtual cornerstone of the body’s health. When the kidneys fail, the effects are normally felt via other organs and systems consequently affected. In this way, taking into consideration only its effect on the kidneys, Cordyceps truly was a promoter of overall health and homeostasis. Many other traditional uses for this mushroom can be traced back to proper kidney health. Fatigue, impotence, joint and back pain, and even ringing in the ears are all symptoms of degenerative kidney health. It has been shown that much of Cordyceps kidney-enhancing potential comes from its ability to increase 17-hydroxy-corticosteroid and 17-ketosteroid levels (Zhu et al. 1998).
Chronic renal failure is a serious and all too common disease, often affecting the elderly. In a study with 51 patients suffering chronic renal failure, it was found that the administration of 3–5 g per day of O. sinensis significantly improved both the kidney function and the overall immune function of the patients receiving the Cordyceps, as compared to the control group who did not receive the Cordyceps (Guan et al. 1992). Patients with chronic renal failure or reduced kidney function often suffer from hypertension, proteinuria, and anemia. In a study with such patients, it was found that after 1 month on Cordyceps, a 15% reduction in blood pressure was observed. Urinary protein was also significantly reduced validating the long-held TCM belief that Cordyceps is valuable for treating diseases and weakness of the kidneys.
In another recent study, the mechanism of action for Cordyceps’ kidney-protection mechanisms was studied, gaining insight into how and why Cordyceps works in preventing and reversing kidney damage (Zhong et al. 2012).
Several studies have shown Cordyceps to be especially helpful when used in conjunction with cyclosporine for renal transplant patients, reducing the required dose of cyclosporine and at the same time reducing the cyclosporine-induced kidney damage, toxicity, and side effects (Ding et al. 2010; Wojcikowski et al. 2006).
Listed here are just a few of the many trials that have been conducted on kidney function with Cordyceps, both animal and human, showing Cordyceps to be of particular value in maintaining kidney health and in restoring function to diseased and damaged kidneys. A search on http://scholar.google.com conducted on April 17, 2017, using “Cordyceps AND Kidney” as the keywords, revealed over 5200 scholarly articles, showing the extent to which this use has been researched. From evaluating the results of the many kidney studies, Cordyceps appears to be a low-cost, low-toxicity medicine that is well tolerated and has real value for clinical application in this field.
3.8.5 Lungs
TCM has characterized Cordyceps as a guardian of respiratory health for many hundreds of years. Much of its reputation for protecting the lungs is believed to come from its ability to promote enhanced oxygen utilization efficacy. In environments lacking sufficient oxygen levels, mice treated with Cordyceps were able to survive up to three times longer than those left untreated, demonstrating a more efficient utilization of the available oxygen. This is objective confirmation of Cordyceps long history of use in preventing and treating altitude sickness (Zhu et al. 1998). Such efficacy alludes to the use of Cordyceps as an effective treatment for bronchitis, asthma, and chronic obstructive pulmonary disease (COPD). Extracts of O. sinensis have been shown to inhibit tracheal contractions, especially important for asthma patients in that it allows for increased airflow to the lungs (Hsu et al. 2008). In addition, its anti-inflammatory properties bring further relief to asthma patients, whose airways become obstructed due to an allergic reaction resulting in swelling of the bronchial pathways (Rao et al. 2007). In a clinical trial involving 50 asthma patients, efficacy against symptoms among the group treated with Cordyceps was 81.3%, within an average of 5 days, while among those treated with conventional antihistamines, the rate was only 61.1% and took an average of 9 days for symptoms to subside (Halpern 1999).
There have been many well-designed trials with Cordyceps for improving lung function in humans, using Cordyceps to treat all manner of respiratory illnesses including asthma, COPD, and bronchitis, either alone or as an adjunct to other standard therapies. It has proven useful for all of these conditions (Zhu et al. 1998). Other recent studies have elucidated the mechanisms of action whereby Cordyceps is able to offset the effects of hypoxia – low oxygen levels (Singh et al. 2013). While the use of Cordyceps for enhanced performance in low-oxygen environments has long been observed, for example its use in high-altitude mountain climbing, this is the first time the actual mechanisms responsible for this activity have been explained. It has been observed for centuries by thousands of TCM practitioners that Cordyceps improves respiratory function, and it is now a well-proven and well-accepted scientific fact.
3.8.6 Heart
One of the more profound actions of Cordyceps, both traditionally and in modern practice, is its ability to stabilize the heart beat and correct heart arrhythmias. Cordyceps is one of the first-line medications of choice for this serious condition in China today. While the exact reasons and mechanism of action for Cordyceps excellent reputation in controlling arrhythmias are only partly understood, it was proposed in earlier studies that the anti-arrhythmia action is due to the presence of adenosine (Peleg and Porter 1990). A study conducted in 2013 has shown this to be correct (Yan et al. 2013). Cordyceps often contains a significant quantity of adenosine, deoxyadenosine, and related adenosine-type nucleotides and nucleosides. It has been shown that these compounds have a widespread effect on coronary and cerebral circulation (Toda et al. 1982; Berne 1980). While no single drug or herb is equally effective in all patients, it is rare that a patient’s arrhythmia does not benefit from the addition of Cordyceps to the treatment regimen. Cordyceps is not known to adversely react with any other arrhythmia medication, and with its low toxicity, it seems to be an excellent choice for this condition.
In studies of patients suffering from chronic heart failure, the long-term administration of Cordyceps in combination with conventional treatments, digoxin, hydrochlorothiazide, dopamine, and dobutamine, promoted an increase in the overall quality of life. This included general physical condition, mental health, sexual drive, and cardiac function, compared to the control group (Chen 1995).
3.8.7 Uses Against Male and Female Sexual Dysfunction
Cordyceps has been used for centuries in traditional Chinese medicine to treat male and female sexual dysfunction, such as hypolibidinism and impotence. Preclinical data on the effects of O. sinensis on mice showed sex steroid-like effects. Human clinical trials have demonstrated similarly in the effectiveness of Cordyceps in combating decreased sex drive. The results of one such study showed an increase in 24-h urine 17-ketosteroid, compared to the control group (Zhu et al. 1998). “These results indicated that CS-4 might affect patients’ sexual drive and functions, either via sex hormone systems or by directly acting on the sexual organs, in parallel with the effects on the hypothalamic-pituitary-adrenocortical axis” (quote from reference). The presence of amino acids, vitamins, zinc, and other trace elements found in Cordyceps is hypothesized to account for increased sperm survival rates, as demonstrated in clinical and preclinical studies (Guo 1986). In three separate studies done in China on a total of 756 patients who were reporting decreased sex drive (hypolibidinism), the patients were given either a placebo or O. sinensis at 3 g per day for 40 days. By the completion of the 40-day study, 64.8% of the patients in the Cordyceps groups reported improvement in their sex drive, while only 23.8% showed improvement in the placebo group (Zhu et al. 1998). In these three related studies alone, 492 patients with a noted lack of sex drive found relief from this condition by using Cordyceps. In another study on both elderly men and women with complaints of decreased libido, impotence, and other sexual malfunctions, Cordyceps was given at 3 g per day for 40 days, and several measurements were taken to determine the degree of improvement. Increased sperm survival time, increased sperm count, and decreased number of malformed sperm were noted in the majority of male subjects, as well as more than double the number of patients reporting reversal of their impotence. Improvements in hypoleukorrhagia, menoxenia, and sex drive were noted in the majority of women subjects (Zhu et al. 1998). Cordyceps is clearly indicated as a therapeutic agent in treating hypolibidenism and other sexual malfunction in both men and women.
Currently, as of the spring of 2017, the main usage for O. sinensis in Asia is for decreasing impotence and improving erectile dysfunction (ED). O. sinensis is widely regarded throughout Asia as the “Herbal Viagra” or “Himalayan Viagra.” A search of the research literature does not show any reliable studies proving the ED effect claimed for Cordyceps, but nonetheless, it is widely believed to be effective for this condition, and ED is thought to be one of the main driving forces of the O. sinensis trade today (Winkler 2010).
3.9 Safety and Toxicity
Cordyceps, both wild-collected and cultivated products, have proven to be a very nontoxic herbal substance for something with the obviously wide-ranging physical effects on the body. While no human toxicity studies have been reported, animal models have found an LD50 (median lethal dose) of 27 g/kg when injected IP in mice. Given by mouth to rabbits for months at 80 g/day, no abnormalities were seen from blood tests or in kidney or liver function (Zhu et al. 1998). Cordyceps s.lat. is thought to be a very safe substance with a minimal potential of toxicity.
3.10 Conclusions
Considering O. sinensis’ long history of use, it seems quite likely there is some truth behind the observed medicinal efficacy. In our modern age, we strive to test and understand why and how these natural medicines work. With O. sinensis and other species of the Cordyceps s.lat. group, this challenge has been greater than with many other herbals, primarily due to the enormous cost and scarcity of the wild-collected material. But today, we are in an age of rapidly expanding biotechnological progress. This has provided us with ways to lab produce Cordyceps spp. in large enough volume, and at a low enough cost, that research becomes practical for anyone interested in looking into the characteristics and medicinal efficacy of these unique organisms. As we investigate deeper, we shall learn more and more of Cordyceps secrets, and we might find that this once rare medicinal herb may hold the key to controlling some of our more difficult medical challenges. More research is needed into this and other species of medicinal mushrooms.
References
Adotey G, Quarcoo A, Holliday JC, Fofie S, Saaka B (2011) Effect of immunomodulating and antiviral agent of medicinal mushrooms (immune assist 24/7 TM) on CD4+ T-lymphocyte counts of HIV-infected patients. Int J Med Mushr 13(2):109–113. doi:10.1615/IntJMedMushr.v13.i2.20
Battle J, Ha T, Li C, Della Beffa V, Rice P, Kalbfleisch J, Browder W, Williams D (1998) Ligand binding to the (1→3)-β-D-glucan receptor stimulates NFκB activation, but not apoptosis in U937 cells. Biochem Bioph Res Co 249(2):499–504. doi.org/10.1006/bbrc.1998.9175
Bensky D, Clavey S, Stoger E (2004) In: Chinese herbal medicine: materia medica, 3rd edn. Eastland Press, Seattle, p 770
Berne RM (1980) The role of adenosine in the regulation of coronary blood flow. Circ Res 47(6):807–813
Boesi A, Cardi F (2005) Wildlife and plants in traditional and modern Tibet: conceptions, exploitation and conservation. Soc Ital Sci Nat 34(1/2)
Boesi A, Cardi F (2009) Cordyceps sinensis medicinal fungus: traditional use among Tibetan people, harvesting techniques, and modern uses. Herbal Gram 83:52–63
Chen DG (1995) Effects of Jin Shui Bao capsule on the quality of life of patients with heart failure. J Admin Trad Chin Med 5:40–43
Chen J, Seviour R (2007) Medicinal importance of fungal β-(1→ 3),(1→ 6)-glucans. Mycol Res 111(6):635–652. doi.org/10.1016/j.mycres.2007.02.011
PubMed
Chen S, Yin D, Li L, Zha X, Shuen J, Zhama C (2000) Resources and distribution of Cordyceps sinensis in Naqu Tibet. Zhong yao cai= Zhongyaocai= J Chin Med Mat 23(11):673–675
Chen YQ, Wang N, Qu LH, Li TH, Zhang WM (2001) Determination of the anamorph of Cordyceps sinensis inferred from the analysis of the ribosomal DNA internal transcribed spacers and 5.8 S rDNA. Biochem Syst Ecol 29(6):597–607. http://doi.org/10.1016/S0305-1978(00)00100-9
PubMed
Chen LS, Stellrecht CM, Gandhi V (2008) RNA directed agent, cordycepin, induces cell death in multiple myeloma cells. Brit J Haematol 140(6):682–391. doi:10.1111/j.1365-2141.2007.06955.x
Chen Y, Yang SH, Hueng DY, Syu JP, Liao CC, Wu YC (2014) Cordycepin induces apoptosis of C6 glioma cells through the adenosine 2A receptor-p53-caspase-7-PARP pathway. Chem Biol Interact 216:17–25. http://doi.org/10.1016/j.cbi.2014.03.010
PubMed
Choi S, Lim MH, Kim KM, Jeon BH, Song WO, Kim TW (2011) Cordycepin-induced apoptosis and autophagy in breast cancer cells are independent of the estrogen receptor. Toxicol Appl Pharmacol 257(2):165–173. doi.org/10.1016/j.taap.2011.08.030
PubMed
Cleaver PD, Holliday JC, Powers ML (2008) US Patent 8,008,060, Novel method for growing Cordyceps sinensis on a substrate and novel method for hybridizing different strains of Cordyceps sinensis. Issued August 2008
Danielson ME, Dauth R, Elmasry NA, Langeslay RR, Magee AS, Will PM (2010) Enzymatic method to measure β-1, 3-β-1, 6-glucan content in extracts and formulated products (GEM assay). J Agri Food Chem 58(19):10305–10308. doi:10.1021/jf102003m
Das SK, Masuda M, Sakurai A, Sakakibara M (2010) Medicinal uses of the mushroom Cordyceps militaris: current state and prospects. Fitoterapia 81(8):961–968. http://doi.org/10.1016/j.fitote.2010.07.010
PubMed
Ding C, Tian PX, Xue W, Ding X, Yan H, Pan X, Feng X, Xiang H, Hou J, Tian X (2010) Efficacy of Cordyceps sinensis in long term treatment of renal transplant patients. Front Biosci (Elite Ed) 3:301–307. doi:10.2741/e245
El Enshasy HA, Hatti-Kaul R (2013) Mushroom immunomodulators: unique molecules with unlimited applications. Trends Biotechnol 31(12):668–677. http://doi.org/10.1016/j.tibtech.2013.09.003
PubMed
Gianotti BM, Cleaver MP, Mullins MN, West SY (2009) Preclinical evaluation of concurrent medicinal mushroom-based immune-enhancement supplementation in dogs undergoing chemotherapy for various cancers. Int J Med Mushr 11(2):167–184. doi:10.1615/IntJMedMushr.v11.i2.60
Guan YJ, Hu Z, Hou M (1992) Effect of Cordyceps sinensis on T-lymphocyte subsets in chronic renal failure. Zhongguo Zhong xi yi jie he za zhi Zhongguo Zhongxiyi jiehe zazhi. Chin J Integrat Trad Western Med 12(6):338–339
Guo YZ (1986) Medicinal chemistry, pharmacology and clinical applications of fermented mycelia of Cordyceps sinensis and Jin Shui Bao capsule. J Modern Diag Ther 1:60–65
Halpern GM (1999) Cordyceps: China’s healing mushroom. Avery Publishing Group, New York
Halpern GM (2007) Healing mushrooms. Square One Publishers, New York
Halpern GM, Miller AP (2002) Medicinal mushrooms: ancient remedies for modern ailments. Rowman & Littlefield, Lanham, p 20706
Hawksworth DL (2011) A new dawn for the naming of fungi: impacts of decisions made in Melbourne in July 2011 on the future publication and regulation of fungal names. IMA Fungus 2(2):155–162. https://doi.org/10.5598/imafungus.2011.02.02.06
PubMedPubMedCentral
Hirsch KR, Smith-Ryan AE, Roelofs EJ, Trexler ET, Mock MG (2017) Cordyceps militaris improves tolerance to high-intensity exercise after acute and chronic supplementation. J Diet Suppl 14(1):42–53. http://dx.doi.org/10.1080/19390211.2016.1203386
Holliday JC, Cleaver MP (2008) Medicinal value of the caterpillar fungi species of the genus Cordyceps (Fr.) link (Ascomycetes). A review. Int J Med Mushr 10(3):219–234. doi:10.1615/IntJMedMushr.v10.i3.30
Holliday JC, Cleaver P, Loomis-Powers M, Patel D (2004) Analysis of quality and techniques for hybridization of medicinal fungus Cordyceps sinensis (Berk.) Sacc. (Ascomycetes). Int J Med Mushr 6(2):151–164. doi:10.1615/IntJMedMushr.v6.i2.60
Hsu TH, Shiao LH, Hsieh C, Chang DM (2002) A comparison of the chemical composition and bioactive ingredients of the Chinese medicinal mushroom Dong Chong Xia Cao, its counterfeit and mimic, and fermented mycelium of Cordyceps sinensis. Food Chem 78(4):463–469. http://doi.org/10.1016/S0308-8146(02)00158-9
Hsu CH, Sun HL, Sheu JN, Ku MS, Hu CM, Chan Y, Lue KH (2008) Effects of the immunomodulatory agent Cordyceps militaris on airway inflammation in a mouse asthma model. Pediatr Neonatol 49(5):171–178. https://doi.org/10.1016/S1875-9572(09)60004-8
PubMed
Hsu JH, Jhou BY, Yeh SH, Chen YL, Chen CC (2015) Healthcare functions of Cordyceps cicadae. J Nutr Food Sci 5(6):1–7. doi:10.4172/2155-9600.1000432
Janeway CA (1992) The immune system evolved to discriminate infectious nonself from noninfectious self. Immunol Today 13(1):1–16. doi.org/10.1016/0167-5699(92)90198-G
Jen CY, Lin CY, Huang BM, Leu SF (2010) Cordycepin induced MA-10 mouse Leydig tumor cell apoptosis through caspase-9 pathway. Evid Based Compl Altern Med 2011:1–11. http://dx.doi.org/10.1093/ecam/nen084
Jeong JW, Jin CY, Park C, Hong SH, Kim GY, Jeong YK, Lee JD, Yoo YH, Choi YH (2011) Induction of apoptosis by cordycepin via reactive oxygen species generation in human leukemia cells. Toxicol In Vitro 25(4):817–824. doi.org/10.1016/j.tiv.2011.02.001
PubMed
Jeong JW, Jin CY, Park C, Han MH, Kim GY, Moon SK, Kim CG, Jeong YK, Kim WJ, Lee JD, Choi YH (2012) Inhibition of migration and invasion of LNCaP human prostate carcinoma cells by cordycepin through inactivation of Akt. Int J Oncol 40(5):1697. doi:10.3892/ijo.2012.1332
PubMed
Jia JM, Ma XC, Wu CF, Wu LJ, Hu GS (2005) Cordycedipeptide A, a new cyclodipeptide from the culture liquid of Cordyceps sinensis (Berk.) Sacc. Chem Pharm Bull 53(5):582–583. http://doi.org./10.1248/cpb.53.582PubMed
Kennedy EM, Gavegnano C, Nguyen L, Slater R, Lucas A, Fromentin E, Schinazi RF, Kim B (2010) Ribonucleoside triphosphates as substrate of human immunodeficiency virus type 1 reverse transcriptase in human macrophages. J Biol Chem 285(50):39380–39391. doi:10.1074/jbc.M110.178582
PubMedPubMedCentral
Kim SW, Hwang HJ, Xu CP, Sung JM, Choi JW, Yun JW (2003) Optimization of submerged culture process for the production of mycelial biomass and exo-polysaccharides by Cordyceps militaris C738. J Appl Microbiol 94(1):120–126. doi:10.1046/j.1365-2672.2003.01754.x
PubMed
Kim H, Naura AS, Errami Y, Ju J, Hamid Boulares A (2011) Cordycepin blocks lung injury-associated inflammation and promotes BRCA1-deficient breast cancer cell killing by effectively inhibiting PARP. Mol Med 17(9):893. doi:10.2119/molmed.2011.00032
PubMedPubMedCentral
Ko BS, Lu YJ, Yao WL, Liu TA, Tzean SS, Shen TL, Liou JY (2013) Cordycepin regulates GSK-3β/β-catenin signaling in human leukemia cells. PLoS One 8(9):e76320. doi.org/10.1371/journal.pone.0076320
PubMedPubMedCentral
Krasnoff SB, Reátegui RF, Wagenaar MM, Gloer JB, Gibson DM (2005) Cicadapeptins I and II: new Aib-containing peptides from the entomopathogenic fungus Cordyceps heteropoda. J Nat Prod 68(1):50–55. doi:10.1021/np0497189
PubMed
Lee SJ, Kim SK, Choi WS, Kim WJ, Moon SK (2009) Cordycepin causes p21WAF1-mediated G2/M cell-cycle arrest by regulating c-Jun N-terminal kinase activation in human bladder cancer cells. Arch Biochem Biophys 490(2):103–109. doi.org/10.1016/j.abb.2009.09.001
PubMed
Lee EJ, Kim WJ, Moon SK (2010) Cordycepin suppresses TNF-alpha-induced invasion, migration and matrix metalloproteinase-9 expression in human bladder cancer cells. Phytother Res 24(12):1755–1761PubMed
Lee JH, Hong SM, Yun JY, Myoung H, Kim MJ (2011) Anti-cancer effects of cordycepin on oral squamous cell carcinoma proliferation and apoptosis in vitro. J Cancer Ther 2(02):224. doi:10.4236/jct.2011.22029
Lee HJ, Burger P, Vogel M, Friese K, Brüning A (2012) The nucleoside antagonist cordycepin causes DNA double strand breaks in breast cancer cells. Investig New Drugs 30(5):1917–1925. doi:10.1007/s10637-012-9859-x
Lee HH, Jeong JW, Lee JH, Kim GY, Cheong J, Jeong YK, Yoo YH, Choi YH (2013a) Cordycepin increases sensitivity of Hep3B human hepatocellular carcinoma cells to TRAIL-mediated apoptosis by inactivating the JNK signaling pathway. Oncol Rep 30(3):1257–1264. doi.org/10.3892/ijmm.2012.1173
PubMed
Lee SY, Debnath T, Kim SK, Lim BO (2013b) Anti-cancer effect and apoptosis induction of cordycepin through DR3 pathway in the human colonic cancer cell HT-29. Food Chem Toxicol 60:439–447. doi.org/10.1016/j.fct.2013.07.068
PubMed
Lee HH, Hwang WD, Jeong JW, Park C, Han MH, Hong SH, Jeong YK, Choi YH (2014a) Induction of apoptosis and G2/M cell cycle arrest by cordycepin in human prostate carcinoma LNCap cells. J Life Sci 24(1):92–97. doi:10.5352/JLS.2014.24.1.92
Lee HH, Kim SO, Kim GY, Moon SK, Kim WJ, Jeong YK, Yoo YH, Choi YH (2014b) Involvement of autophagy in cordycepin-induced apoptosis in human prostate carcinoma LNCaP cells. Environ Toxicol Pharmacol 38(1):239–250. doi.org/10.1016/j.etap.2014.06.003
PubMed
Lee HH, Park H, Sung GH, Lee K, Lee T, Lee I, Park MS, Jung YW, Shin YS, Kang H, Cho H (2014c) Anti-influenza effect of Cordyceps militaris through immunomodulation in a DBA/2 mouse model. J Microbiol 52(8):696–701. doi:10.1007/s12275-014-4300-0
PubMed
Li C (2004) Treatment of chronic viral hepatitis B with Jing shui bao and lamivudine: a report of 60 cases [J]. J Anhui Trad Chin Med Coll 2:008
Li SP, Yang FQ, Tsim KW (2006) Quality control of Cordyceps sinensis, a valued traditional Chinese medicine. J Pharma Biomed Analy 41(5):1571–1584. http://doi.org/10.1016/j.jpba.2006.01.046
Li Y, Li R, Zhu S, Zhou R, Wang L, Du J, Wang Y, Zhou B, Mai L (2015) Cordycepin induces apoptosis and autophagy in human neuroblastoma SK-N-SH and BE (2)-M17 cells. Oncol Lett 9(6):2541–2547. doi:10.3892/ol.2015.3066
PubMedPubMedCentral
Lin BQ, Li SP (2011) Cordyceps as an herbal drug. In: Benzie IFF, Wachtel-Galor S (eds) Herbal medicine, biomolecular and clinical aspects, 2nd edn. CRC Press, Boca Raton, pp 73–105
Lo HC, Hsieh C, Lin FY, Hsu TH (2013) A systematic review of the mysterious caterpillar fungus Ophiocordyceps sinensis in Dong Chong Xia Cao (冬蟲夏草 Dōng Chóng Xià Cǎo) and related bioactive ingredients. J Trad Comp Med 3(1):16–32. http://doi.org/10.1016/S2225-4110(16)30164-X
Lu L (2003) Study on effect of Cordyceps sinensis and artemisinin in preventing recurrence of lupus nephritis. Altern Med Rev 8(2):209–210. doi:10.1007/BF02934427
Lu H, Li X, Zhang J, Shi H, Zhu X, He X (2014) Effects of cordycepin on HepG2 and EA. hy926 cells: potential antiproliferative, antimetastatic and anti-angiogenic effects on hepatocellular carcinoma. Oncol Lett 7(5):1556–1562. doi.org/10.3892/ijmm.2012.1173
PubMedPubMedCentral
Mang CY, Liu CP, Liu GM, Jiang B, Lan H, Wu KC, Yan Y, Li HF, Yang MH, Zhao Y (2015) Theoretical searches and spectral computations of preferred conformations of various absolute configurations for a cyclodipeptide, cordycedipeptide A from the culture liquid of Cordyceps sinensis. Spectrochim Acta Part A: Mol Biomol Spectro 136:1401–1408. http://doi.org/10.1016/j.saa.2014.10.028
McNeill J, Turland NJ (2011) Major changes to the code of nomenclature-Melbourne. Taxon 60(5):495–1497
Megazyme International Ireland Limited (2008) Mushroom and yeast beta-glucan assay procedure manual, Megazyme Corp, IDA Business Park, Bray, Co. Wicklow, A98 YV29 Ireland
Mizuno T (1999) Medicinal effects and utilization of Cordyceps (Fr.) link (Ascomycetes) and Isaria Fr.(Mitosporic fungi) Chinese caterpillar fungi. Int J Med Mushr 1(3):251–262. doi:10.1615/IntJMedMushrooms.v1.i3.80
Moyle B (2009) The black market in China for tiger products. Global Crime 10(1–2):124–143. http://dx.doi.org/10.1080/17440570902783921
Nakamura K, Yoshikawa N, Yamaguchi YU, Kagota S, Shinozuka K, Kunitomo M (2006) Antitumor effect of cordycepin (3′-deoxyadenosine) on mouse melanoma and lung carcinoma cells involves adenosine A3 receptor stimulation. Anticancer Res 26(1A):43–47PubMed
Noh EM, Youn HJ, Jung SH, Han JH, Jeong YJ, Chung EY, Jung JY, Kim BS, Lee SH, Lee YR, Kim JS (2010) Cordycepin inhibits TPA-induced matrix metalloproteinase-9 expression by suppressing the MAPK/AP-1 pathway in MCF-7 human breast cancer cells. Int J Mol Med 25(2):255–260. doi:10.3892/ijmm_00000338
PubMed
Pan BS, Lin C, Huang BM (2011) The effect of cordycepin on steroidogenesis and apoptosis in MA-10 mouse Leydig tumor cells. Evid Based Com Alter Med 2011:1–14. http://dx.doi.org/10.1155/2011/750468
Park HW, Jun DY, Kim YH (2002) Apoptotic activity of insect pathogenic fungus Paecilomyces japonica toward human acute leukemia Jurkat T cells is associated with mitochondria-dependent caspase-3 activation regulated by bcl-2. J Microbiol Biotechnol 12(6):950–956
Paterson RRM (2008) Cordyceps – a traditional Chinese medicine and another fungal therapeutic biofactory? Phytochemistry 69(7):1469–1495. http://doi.org/10.1016/j.phytochem.2008.01.027
PubMed
Pelleg A, Porter RS (1990) The pharmacology of adenosine. Pharmacother: J Hum Pharmacol Drug Ther 10(3):157–174
Rao YK, Fang SH, Tzeng YM (2007) Evaluation of the anti-inflammatory and anti-proliferation tumoral cells activities of Antrodia camphorata, Cordyceps sinensis, and Cinnamomum osmophloeum bark extracts. J Ethnopharmacol 114(1):78–85. http://doi.org/10.1016/j.jep.2007.07.028
PubMed
Relph D (1991) New Zealand geographic, 012, Oct-Dec 1991. Accessed at https://www.nzgeo.com/stories/caterpillar-killer/. 29 Mar 2017
Ruwei W, Yiyuan X, Peijun J, Wang X, Holliday J (2003) Clinical trial of immune-assist™ as an adjunct for chemo- and radiation therapy. http://www.ia-micron.com/IA_Mic_Cancer_Trial3.pdf. Accessed 29 Mar 2017
Ruwei W, Jianjun X, Hongpeng Z, Songhua L, Jin Y, Wang Y, Holliday J (2004) Phase 1 clinical trial on treatment of chronic Hepatitis B using immune assist brand dietary supplement as an adjunct with Lamivudine [Epivir], http://www.ia-micron.com/hepbstudy.pdf. Accessed 30 Mar 2017
Segelken R (2002) Cyclosporin mold’s “sexual state”found in New York forest Cornell students’ discovery could target additional sources of nature-based pharmaceuticals. Cornell Univ. Sci. News
Shi P, Huang Z, Tan X, Chen G (2008) Proteomic detection of changes in protein expression induced by cordycepin in human hepatocellular carcinoma BEL-7402 cells. Methods Find Exp Clin Pharmacol 30(5):347–353. doi:10.1358/mf.2008.30.5.1186085
PubMed
Shrestha UB, Bawa KS (2013) Trade, harvest, and conservation of caterpillar fungus (Ophiocordyceps sinensis) in the Himalayas. Biol Conserv 159:514–520. http://doi.org/10.1016/j.biocon.2012.10.032
Shrestha S, Shrestha AK, Park JH, Lee DY, Cho JG, Shrestha B, Baek NI (2014) Review on pharmacologically active metabolites from Yarsagumba (Ophiocordyceps sinensis), an epitome of Himalayan elixir. Nepal J Sci Tech 14(2):49–58. http://dx.doi.org/10.3126/njst.v14i2.10415
Singh M, Tulsawani R, Koganti P, Chauhan A, Manickam M, Misra K (2013) Cordyceps sinensis increases hypoxia tolerance by inducing heme oxygenase-1 and metallothionein via Nrf2 activation in human lung epithelial cells. Biomed Res Int 2013:1–13. http://dx.doi.org/10.1155/2013/569206
Siu KM, Mak DH, Chiu PY, Poon MK, Du Y, Ko KM (2004) Pharmacological basis of ‘Yin-nourishing’ and ‘Yang-invigorating’ actions of Cordyceps, a Chinese tonifying herb. Life Sci 76(4):385–395. http://doi.org/10.1016/j.lfs.2004.07.014
PubMed
Sung GH, Hywel-Jones NL, Sung JM, Luangsa-ard JJ, Shrestha B, Spatafora JW (2007) Phylogenetic classification of Cordyceps and the clavicipitaceous fungi. Stud Mycol 57:5–59. doi:10.3114/sim.2007.57.01
PubMedPubMedCentral
Thomadaki H, Tsiapalis CM, Scorilas A (2008) The effect of the polyadenylation inhibitor cordycepin on human molt-4 and Daudi leukaemia and lymphoma cell lines. Cancer Chemother Pharmacol 61(4):703–711. doi:10.1007/s00280-007-0533-5
PubMed
Toda N, Okunishi H, Taniyama K, Miyazaki M (1982) Responses to adenine nucleotides and related compounds of isolated dog cerebral, coronary and mesenteric arteries. J Vasc Res 19(5):226–236
Tuli HS, Kumar G, Sandhu SS, Sharma AK, Kashyap D (2015) Apoptotic effect of cordycepin on A549 human lung cancer cell line. Turkish J Biol 39(2):306–311
Wang J, Liu YM, Cao W, Yao KW, Liu ZQ, Guo JY (2012a) Anti-inflammation and antioxidant effect of cordymin, a peptide purified from the medicinal mushroom Cordyceps sinensis, in middle cerebral artery occlusion-induced focal cerebral ischemia in rats. Metab Brain Dis 27(2):159–165. doi:10.1007/s11011-012-9282-1
Wang SX, Liu Y, Zhang GQ, Zhao S, Xu F, Geng XL, Wang HX (2012b) Cordysobin, a novel alkaline serine protease with HIV-1 reverse transcriptase inhibitory activity from the medicinal mushroom Cordyceps sobolifera. J Biosci Bioeng 113(1):42–47. http://doi.org/10.1016/j.jbiosc.2011.09.005
PubMed
Winkler D (2008) Yartsa Gunbu (Cordyceps sinensis) and the fungal commodification of Tibet’s rural economy. Econ Bot 62(3):291–305. doi:10.1007/s12231-008-9038-3
Winkler D (2010) Cordyceps sinensis: a precious parasitic fungus infecting Tibet. Field Mycol 11(2):60–67. https://doi.org/10.1016/j.fldmyc.2010.04.009
Wojcikowski K, Johnson DW, Gobe G (2006) Herbs or natural substances as complementary therapies for chronic kidney disease: ideas for future studies. J Lab Clinical Med 147(4):160–166. http://doi.org/10.1016/j.lab.2005.11.011
Wright B (2012) Indian tiger is Tibet’s second skin. Conservation India. http://www.conservationindia.org/articles/indian-tiger-is-tibets-second-skin. Accessed 20 Apr 2017
Wu WC, Hsiao JR, Lian YY, Lin CY, Huang BM (2007) The apoptotic effect of cordycepin on human OEC-M1 oral cancer cell line. Cancer Chemother Pharmacol 60(1):103–111. doi:10.1007/s00280-006-0354-y
PubMed
Xia F, Chen X, Guo MY, Bai XH, Liu Y, Shen GR, Li YL, Lin J, Zhou XW (2016) High-throughput sequencing-based analysis of endogenetic fungal communities inhabiting the Chinese Cordyceps reveals unexpectedly high fungal diversity. Sci Rep 6:33437. doi:10.1038/srep33437
PubMedPubMedCentral
Yan XF, Zhang ZM, Yao HY, Guan Y, Zhu JP, Zhang LH, Jia YL, Wang RW (2013) Cardiovascular protection and antioxidant activity of the extracts from the mycelia of Cordyceps sinensis act partially via adenosine receptors. Phytother Res 27(11):597–1604
Yang LY, Chen A, Kuo YC, Lin CY (1999) Efficacy of a pure compound H1-A extracted from Cordyceps sinensis on autoimmune disease of MRL lpr/lpr mice. J Lab Clin Med 134(5):492–500. http://doi.org/10.1016/S0022-2143(99)90171-3
PubMed
Yang FQ, Guan J, Li SP (2007) Fast simultaneous determination of 14 nucleosides and nucleobases in cultured Cordyceps using ultra-performance liquid chromatography. Talanta 73(2):269–273. http://doi.org/10.1016/j.talanta.2007.03.034
PubMed
Yoshikawa N, Yamada S, Takeuchi C, Kagota S, Shinozuka K, Kunitomo M, Nakamura K (2008) Cordycepin (3′-deoxyadenosine) inhibits the growth of B16-BL6 mouse melanoma cells through the stimulation of adenosine A3 receptor followed by glycogen synthase kinase-3β activation and cyclin D1 suppression. Naunyn Schmiedeberg’s Arch Pharmacol 377(4–6):591–595. doi:10.1007/s00210-007-0218-y
Yoshikawa N, Kunitomo M, Kagota S, Shinozuka K, Nakamura K (2009) Inhibitory effect of cordycepin on hematogenic metastasis of B16-F1 mouse melanoma cells accelerated by adenosine-5′-diphosphate. Anticancer Res 29(10):3857–3860PubMed
Yu L, Zhao J, Li SP, Fan H, Hong M, Wang YT, Zhu Q (2006) Quality evaluation of Cordyceps through simultaneous determination of eleven nucleosides and bases by RP-HPLC. J Sep Sci 29(7):953–958. doi:10.1002/jssc.200600007
PubMed
Yue K, Ye M, Zhou Z, Sun W, Lin X (2013) The genus Cordyceps: a chemical and pharmacological review. J Pharm Pharmacol 65:474–493. doi:10.1111/j.2042-7158.2012.01601.x
Zhang SS, Zhang DS, Zhu TJ, Chen XY (1990) A pharmacological analysis of the amino acid components of Cordyceps sinensis Sacc. Yao xue xue bao= Acta Pharm Sin 26(5):326–330
Zhao J, Xie J, Wang LY, Li SP (2014) Advanced development in chemical analysis of Cordyceps. J Pharma Biomed Anal 87:271–289. http://doi.org/10.1016/j.jpba.2013.04.025
Zhong S, Pan H, Fan L, Lv G, Wu Y, Parmeswaran B, Pandey A, Soccol CR (2009) Advances in research of polysaccharides in Cordyceps species. Differences 13:14
Zhong F, Liu X, Zhou Q, Hao X, Lu Y, Guo S, Wang W, Lin D, Chen N (2012) 1H NMR spectroscopy analysis of metabolites in the kidneys provides new insight into pathophysiological mechanisms: applications for treatment with Cordyceps sinensis. Nephrol Dial Transplant 27(2):556–565PubMed
Zhou X, Gong Z, Su Y, Lin J, Tang K (2009) Cordyceps fungi: natural products, pharmacological functions and developmental products. J Pharm Pharmacol 61(3):279–291. doi:10.1211/jpp/61.03.0002
PubMed
Zhu JS, Halpern G, Jones K (1998) The scientific rediscovery of an ancient Chinese herbal medicine: Cordyceps sinensis. J Altern Complem Med [Part 1] 4 (3): 289–303 [part 2] 4 (4): 429–457
Zhu F, Du B, Bian Z, Xu B (2015) Beta-glucans from edible and medicinal mushrooms: characteristics, physicochemical and biological activities. J Food Compos Anal 41:165–173. http://doi.org/10.1016/j.jfca.2015.01.019