There is chaos, there is circumstance, and suddenly there is the whole foundation. There is the background noise, then a noise in the midst of that background noise, and suddenly there’s the whole song.
MICHEL SERRES, GENESIS
There is an embarrassing vagueness about the very beginning.
STEVEN WEINBERG, THE FIRST THREE MINUTES
The century and a half that followed Kant’s uncelebrated Natural History was a time of almost total multiversal dormancy. This dormancy was both marked and reaffirmed by the publication in 1796 of Pierre Laplace’s Exposition du système du monde, which ordered the Kantian chaos into what became known as the “nebular hypothesis,” according to which a hot, rotating nebula produces a sun and its planets.1 What the next generations took this to mean, although Laplace (1749–1827) himself waffled on the matter,2 was that the far-off nebulae were at worst just clouds of gas and at best “potential star-clusters” of “inchoate suns.”3 Laplace’s cosmology became “the standard Victorian model of the universe,” to such an extent that one hundred years later the historian of astronomy Agnes Clerke would write that “the question whether nebulae are external galaxies hardly any longer needs discussion…. No competent thinker … can now, it is safe to say, maintain any single nebula to be a star system of coordinate rank with the Milky Way.” Rather, she insisted, all the visible objects in the night sky, “stellar and nebular … belong to one mighty aggregation, and stand in ordered mutual relations within the limits of one all-embracing scheme,” which is to say the Milky Way itself.4 With very few exceptions (including, remarkably, Edgar Allan Poe),5 this “galactocentric” viewpoint held well into the 1920s, so that when Albert Einstein (1879–1955) published his theory of general relativity in 1916, he believed that the universe consisted of nothing but the Milky Way—a single “island” surrounded by an endless void. He also believed that the universe was eternal. On the largest cosmological scales, then, the twentieth century began more or less where Aristotle had left off: with a single, static world that had existed forever. Then the 1920s hit, and the whole model was suddenly blown apart.
The uniqueness of the Milky Way was dramatically dismantled in 1924, when the New York Times announced that Edwin Hubble (1889–1953) and his assistant Vesto Slipher had determined “the spiral nebulae, which appear in the heavens as whirling clouds,” to be galaxies in their own right. Kant, we will recall, named these structures “world-systems”; Hubble tended to call them “island universes.”6 Hubble had confirmed Kant’s guess by observing luminous stars in the Andromeda and Triangulum (or Messier 33) galaxies, but he also extended his conclusions by analogy to all the other nebulae in the cosmos, which seemed to him even then to number in the “hundreds of thousands.”7 Although it would take until the mid-1990s for the world to see these hundreds of thousands of galaxies—which turned out to be billions of galaxies (figure 5.1)—Hubble’s discovery immediately increased the size of the known universe and the number of worlds within it by a factor of half a million, give or take a few hundred thousand.8 This cosmos, to borrow an Irigarayan turn of phrase, suddenly seemed not to be one.9
As for cosmic eternity, it also began to look implausible in the light of Hubble’s discovery. As he determined in the years that followed, these newfound galaxies were not remaining in place; rather, almost all of them were moving away from one another. More precisely, the space between them was growing and pushing them apart.10 To be sure, this was a strange proposition to early-twentieth-century ears. As a depressed young Alvy Singer explains to a therapist in Woody Allen’s Annie Hall, “The universe is everything.”11 So, by definition, there is nothing “out there” for the receding galaxies to recede into. What Alvy realizes, what prevents him from doing his homework (“What’s the point?” he asks his mother), is that something is pushing the whole world “out” into nothing at all. In more familiar terms, and as Alvy intones miserably, “the universe is expanding.”
Although Hubble observed this phenomenon directly, the possibility of an expanding universe had been opened a decade earlier by Einstein’s theory of general relativity, which had shocked the world in 1916 by revolutionizing Newtonian gravity.12 According to general relativity, space and time do not constitute the impassive, “absolute” background that Newton had assumed; rather, they compose a dynamic, neo-Leibnizian “space-time” that can grow, shrink, bend, and warp in relation to matter and energy.13 Prior to Hubble’s observations, then, Einstein’s own theory suggested that spacetime might be either expanding or contracting. Parts of the universe—even the whole universe itself—might be either racing outward or retreating inward thanks to the warping effects of gravity.
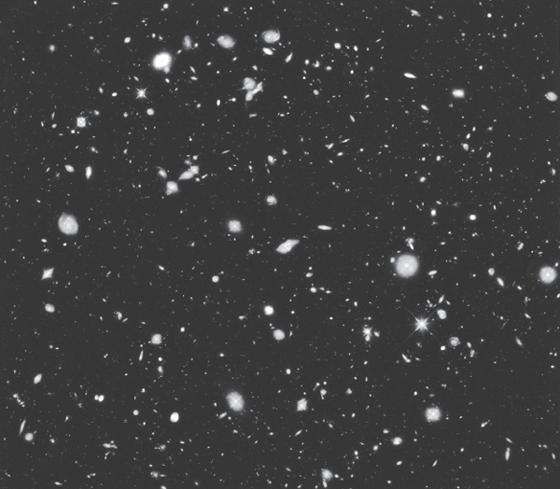
Einstein’s own calculations notwithstanding, this possibility was notoriously difficult for him to abide. One Newtonian belief he retained was that the universe must be static. Something must be regulating the elasticity of space-time, otherwise gravity, which exerts an attractive force on matter, would draw the whole world into a fiery collapse. At this point, we have seen numerous thinkers struggle with this problem. It is clear that matter acts attractively under the influence of gravity, but not at all clear what acts repulsively to keep matter distributed through the cosmos. Both Descartes and Newton ended up relying on God, not only to create matter, but also to provide the outward push that scatters it through the universe. Kant, by contrast, found his repulsive force not in God, but in the Epicurean “swerve,” or clinamen. Einstein, faced with a similar difficulty, appealed neither to God nor to the swerve, instead positing a negative pressure, which he called the “cosmological constant” and designated by the Greek letter lambda (Λ), to offer an equal and opposite push to gravity’s pull.14 With gravity’s attraction and lambda’s repulsion in perfect proportion, Einstein was able (theoretically at least) to keep the universe from stretching out or caving in.
Upon hearing the news of Hubble’s discovery—that space-time was not standing still, but instead expanding “outward”—Einstein immediately retracted his cosmological constant, calling it his “biggest blunder.”15 In the meantime, the work of a young Belgian physicist and priest named Georges Lemaître (1894–1966) was vindicated. Beginning in 1927, Lemaître had posited an expanding universe based on Einstein’s equations, provoking Einstein to tell him, “[Y]our math is correct, but your physics is abominable.”16 The Russian mathematician Alexander Friedmann (1888–1925) had reached a similar conclusion five years earlier in his groundbreaking solutions to Einstein’s gravitational equations, but he died before Hubble’s discovery could confirm his findings.17 As for Lemaître, he took courage from Hubble’s fortuitous observations, going on to suggest that if the universe is expanding now, perhaps it has always been expanding—from the beginning of things. Perhaps if we could play time backward, we would see galaxies getting closer and closer together, the space between them contracting, until we would finally reach a moment when the whole universe would have been crammed into one point. The scientist-priest, soon to be made monsignor, concluded: the whole universe, in all its multiplicity, seems to have burst forth from one tiny ball of nuclear fluid, which he called the “Primeval Atom.”18
This story is so familiar to us now that it is hard to imagine that it was ever controversial. But the middle decades of the twentieth century witnessed impassioned debates not just over the particulars of this strange new cosmogony, but also over the possibility of a scientific cosmogony in the first place. From the dawn of the seventeenth century through the dawn of the twentieth, observational astronomers had refrained from speculating about the origins of the universe, leaving such meditations to philosophers and theologians. Clerke summarized this restraint in 1905, saying, “[W]ith the infinite possibilities beyond, science has no concern.”19 By Einstein’s time, then, the very notion that the universe could have begun at all had become scientifically distasteful; as Steven Weinberg puts it, “[A]n aura of the disreputable always surrounded such research.”20 After all, if the world had a beginning, then what was there before the world? And how did the “before” give way to the “after”? The problem is that if we assume that the universe had a beginning, we are almost forced to appeal to some kind of supernatural force to get it going. Hence, the Victorian scientific consensus that the universe must have existed from eternity: if the world does not have a beginning, then we do not have to worry about what might have begun it.
The rise of the big bang hypothesis thus staged a return of the mythological at the heart of modern science. To the horror of many midcentury physicists, positing a beginning to the universe seemed to open their discipline constitutively to something beyond it. The most contested site of this opening was the big bang’s beginning itself: t = 0, when general relativity predicts that the whole universe would have been squeezed into a “point” of no size. Containing everything in nothing, the temperature and density of this point would be infinite, and, unfortunately, most laws of physics break down at infinity. Physicists call this “pathological circumstance,” when the values hit infinity and the calculations jam, a “singularity.”21
Although cosmologists spent the rest of the twentieth century debating the nature and necessity of such a singularity,22 the floodgates to philosophy and, more troublingly, religion were most definitely open. In particular, many Christian theologians of the mid-twentieth century quickly saw in the big bang’s infinite starting point a confirmation of orthodox doctrine.23 As early as 1952, for example, Pope Pius XII declared to the Pontifical Academy of Science that “present day science, with one sweep back across the centuries, has succeeded in bearing witness to the august instance of the primordial Fiat Lux [let there be light], when along with matter, there burst forth from nothing a sea of light and radiation, and the elements split and churned and formed into millions of galaxies.”24
And the big bang hypothesis, in its standard form at least, does seem to stage an uncanny recapitulation of Christian creation theology. Just as the church has taught, the universe is not eternal but had a temporal beginning. Just as the church has taught, it was born in a sudden flash of light. And just as the church has taught, the whole thing seems to have come out of nothing at all.25
Scholars of varying theological commitments agree that the doctrine of “creation out of nothing” is not exactly biblical; rather, it emerged during the second and third centuries C.E. as early church scholars vied for dominance over the so-called Gnostics, and the teaching was adopted centuries later into a number of strands of Jewish and Muslim theologies.26 Nevertheless, the resemblance between modern science and what physicists considered a “biblical” mythos was so strong and so disturbing that it prompted the renegade British astronomer Fred Hoyle (1915–2001) to look for a different story to tell—preferably one that did not “aid and abet” religion.27 As far as Hoyle was concerned, the chief danger of positing an absolute beginning to the universe was that “a ‘something’ outside physics can then be introduced at t = 0,” and an infinite “something” beyond the reach of physics is almost always called “God.”28 It was Hoyle, in fact, who called his opponent the “big bang” in the first place, intending to ridicule the theory he ended up baptizing. As an alternative and with the collaboration of the mathematicians Thomas Gold and Herman Bondi, Hoyle posited the “steady-state” model, according to which the universe has been cooled and extended from eternity, continually producing new matter to fill the expanding intergalactic void.29 Insofar as this new matter would also be generated out of nothing, this model did not sidestep the Christian narrative altogether, but the key for Hoyle and his colleagues was that if we extrapolate backward from a steady-state universe, the cosmic density comes nowhere near infinity, and there is never a need for an imponderable, incalculable, godlike singularity at the beginning. There is, in fact, no beginning at all.
Unfortunately for these alternative cosmologists, their model was ruled out when radio astronomers Arno Penzias (b. 1933) and Robert Wilson (1927–2002) accidentally discovered the Cosmic Microwave Background (CMB) in 1965 (figure 5.2).30 Known as “the surface of last scattering,” the CMB is a sphere of radiation left over from the big bang—more precisely, from 380,000 years after the bang, when electrons and protons had cooled sufficiently to form hydrogen atoms, allowing light effectively to “decouple” from matter and roam the universe. As such, the CMB provides a thermal record of the aftermath of the primordial blast,31 establishing that the world as we know it cannot have been eternal. Rather, all of it had a beginning—and the same hot-as-hell beginning—in a burst of light, in time. As American astronomer Robert Jastrow sees it, the big bang’s midcentury victory therefore seemed to confirm the “biblical view of the origin of the world.” Jastrow infamously goes on to attest that “for the scientist who has lived by his faith in the power of reason, the story ends like a bad dream. He has scaled the mountains of ignorance, he is about the conquer the highest peak; as he pulls himself over the final rock, he is greeted by a band of theologians who have been there for centuries.”32 It’s enough to drive a scientist mad.
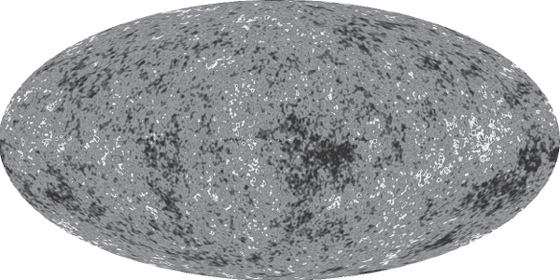
As we have seen, the first point of contested connection between modern and “biblical” cosmology lies in the notion of an initial singularity: a timeless, immaterial, and infinite power that fires forth space and time out of nothing at all.33 The persistent resemblance of the big bang’s infinite creative force to the Abrahamic “God of power and might” prompted physicists over the decades to try to find an alternative model—preferably one that might rid itself of this “singularity” and its weighty theological baggage. With Hoyle’s alternative off the table, some physicists therefore posited an “oscillating” universe as a new way around the singularity.34 According to this model, the amount of matter in the universe is large enough to allow gravity to counteract the initial outward thrust, drawing the universe back after a few billion years into an increasingly smaller, denser state—until it collapses into what some have called a “big crunch” and others a “gnab gib” (“big bang” backward). At the moment of tightest compression, the cosmos “bounces” and explodes again, producing another rush of radiation and elementary particles, another batch of galaxies that race outward before they are drawn back in, and the cycle repeats eternally.
By making “The Big Bang” a mere moment in a succession of other bangs and crunches (a succession sometimes referred to as the “big brunch”), the oscillating model avoided the unsavory notion that anything—let alone the universe—might be created out of nothing. As the astrophysicist John Gribbin wrote in 1976, “The biggest problem with the Big Bang theory of the universe is philosophical—perhaps even theological—what was there before the bang? This problem alone was sufficient to give a great initial impetus to the Steady State theory; but with that theory now sadly in conflict with the observations, the best way round this initial difficulty is provided by a model in which the universe expands from a singularity, collapses back again, and repeats the cycle indefinitely.”35
Interestingly enough, in this very gesture of circumventing Christian cosmology, the oscillating model collided head on with Hindu cosmology, which posits our age as just one in an infinite series of cycles. Friedmann himself, when considering the possibility of a bouncing universe in 1923, wrote that “one cannot help thinking of the tales from the Indian mythology with their periods of life.”36 This resonance, for many theorists, was far more welcome—perhaps even a tacit confirmation of the cyclical model’s transhistorical, cross-cultural truth.37
As it turned out, however, the oscillating model was even shorter-lived than the steady-state model had been. Weinberg notes that although the cyclical universe “nicely avoids the problem of Genesis,” it also runs headlong into the problem of entropy.38 According to the Second Law of Thermodynamics, the entropy (or measure of disorder) of a closed system always increases. This means that each universe that bangs will begin with a higher measure of entropy than the one that crunched before it. More entropy amounts to more radiation, and more radiation means a longer period of expansion before the cosmos contracts again.39 Each cosmos, in other words, would last longer than its predecessor. If the lives of universes get longer into the future, then one can only conclude that they were shorter in the past. Tracing these shortened universes back, we eventually come to a universe of no length at all. This means that the oscillating model leads just as inexorably as the big bang hypothesis to an absolute beginning, when the cycles must have started.40 So much for avoiding the singularity. Moreover, if the total entropy increases with each universe, then it becomes impossible to begin each cycle in the kind of well-ordered (which is to say, low-entropic) state needed to produce a decent cosmos; the universe, it seems, cannot simply collapse and rise again without bringing along its “baggage” from the previous cycle. And so the physics community gradually gave way to the story from which it had spent decades recoiling: the universe does, indeed, seem to have begun with a bang.
Although the story has undergone countless revisions since it was first told, it goes more or less like this. One day—but words fail us here already because there were no days—13.8 billion years ago, a tiny nugget of immeasurable density issued forth a searing white light. The light radiated from an ultrahot, ultradense plasma, producing a great flood of energy as particles raced apart from one another.41 This roiling chaos took 380,000 years to cool down sufficiently for atoms to emerge, leaving us with the thermal record of the CMB. Such cooling is attributable to a regime change that took place 75,000 years after the big bang, when the fires of radiation gave way to the gentler tug of gravity. From the crazy primordial plasma, gravity began to draw nuclei into atoms and atoms into molecules, eventually coaxing them into stars, galaxies, and eventually planets.
Then, at the turn of the third millennium, physicists began to realize that about 7.5 billion years after the big bang, the inward pull of gravity was overcome by the outward push of empty space, and “dark energy” began to push galaxies away from one another at a faster and faster rate (see introduction). So Einstein was not wrong after all. There is a countergravitational force (Λ), but it does not exist in a happy homeostasis with gravity to keep the cosmos steady. Rather, it is accelerating cosmic expansion with every passing moment. According to the now standard model, lambda currently constitutes about 73 percent of the universe.42 This means that as time goes on and space continues to expand, the energy of “empty” space will occupy an increasingly greater percentage of the cosmic mass–energy, accelerating the acceleration at a dizzying clip. Galaxies will eventually race apart from one another faster than the speed of light until they disappear from one another’s view.43 As Robert Kirshner explains, “[I]nstead of seeing more of the contents of the universe, we will see less and less,” our night sky darkening as galaxies slip from sight.44 Even galaxies will eventually unravel, stars will burn out, and matter will become a “thin gruel of particles,” dissolving into a void of dark energy that races eternally outward into nothing.45 And so the world that began with a big bang seems destined to end in what physicists call a “big whimper.”46
As we shall see, however, there are other options. If the value of dark energy is greater than –1, then dark energy is not the “cosmological constant,” but one form of a hypothetical substance called “quintessence.” Its density will decrease with time, eventually producing a cosmic contraction and drawing the universe into an apocalyptic “big crunch.”47 If the value is less than –1, then the force is a different kind of quintessence called “phantom energy,” whose density will increase with time, eventually tearing the universe into a “big rip.”48 But these theories are more or less our three options for the end of the world: a continually expanding void, an infernal implosion, or a great cosmic shredding.
As we saw in the introduction, the rise of the multiverse as a scientifically viable possibility is often traced to the discovery of dark energy. The most common explanation of the multiverse’s sudden respectability is that dark energy’s baffling, quantum-field-theory-defying weakness transformed the old fine-tuning problem into an all-out crisis: if lambda were much stronger, it would have torn the universe apart before anything could form; if it were any weaker, gravity would have collapsed the early universe in on itself. The almost impossible just-rightness of lambda—the painstaking calibration needed to allow life to emerge—prompted theorists to take seriously the possibility that there might be a slew of other universes with different cosmological constants.49 If every value of lambda is tried out somewhere, then it makes sense to say we just happen to live in a universe whose lambda lets us live. I would also suggest, however, that there are also aesthetic, even existential motivations for turning from dark energy to the multiverse. Faced with the equally awful possibilities of the whimper, the crunch, and the rip, it seems that some physicists are looking for a different story to tell.
Of all the universe’s mass–energy, approximately 73 percent is dark energy, 23 percent is dark matter (a persistently unidentified substance that neither absorbs nor releases light),50 and a meager 4 percent is visible matter.51 That is to say, all we can see—tables, particles, puppies, and stars—everything that seems to be, is only 4 percent of what is.52 Because the discovery of dark energy revealed the extent of our cosmic insignificance, theoretical physicist and cosmologist Lawrence Krauss has called it “the ultimate Copernican Revolution.” As we saw in chapter 3, Copernicus unsettled the Platono-Aristo-Christian fantasy that the earth occupied the center of the solar system. Subsequent centuries of astronomy showed that our solar system does not occupy the center of its galaxy and that our galaxy does not occupy the center of the universe. And now with the discovery of dark energy, it seems that the vast majority of the universe is totally inaccessible to us. Far from being the center of anything, Krauss shrugs, “we’re just a bit of pollution” made of cosmic leftovers and living nowhere in particular.53
As we have also seen, it has traditionally been the church that has resisted Copernican revolutions—insisting that there are no “antipodeans,” for example, or subjecting Galileo to lifelong house arrest, or terrifying Descartes into posthumous publication, or burning Bruno at the stake. But when it comes to dark energy, it is not the churches that are in crisis—for the most part, the churches either do not know or do not care about it—but (some) physicists themselves.
Not only was dark energy, from all accounts, “unexpected,” but once it was found, it was also infamously miscalculated by 120 orders of magnitude, prompting Marcelo Gleiser to call it “ugly and unexpected.”54 On top of that, it is still not clear what dark energy is. “The term doesn’t mean anything,” quipped David Schlegel in 2007. “It might not be dark. It might not be energy. The whole name is a placeholder for the description that there’s something funny that was discovered nine years ago now that we don’t understand.”55 So the discovery of this way-too-small, ugly something has initiated what one might call an epistemological crisis in the physics community. As Krauss recently told a group of physicists and astronomers, “You are liable to spend the rest of your lives measuring stuff that won’t tell us what we want to know.”56 Strikingly, however, the way physicists communicate this discovery also reveals the presence in their midst of something akin to an existential crisis.
Assuming dark energy remains at a steady density forever, it will cause galaxies to disappear from our view as time goes on, effectively extinguishing the faraway lights in the sky. Our “descendants” (whatever that means on the time scale of hundreds of billions of years) will eventually see nothing more than a supercluster of the Milky Way, Andromeda, and a few dwarf galaxies, swimming in an endless, empty sea. In other words, as Lawrence Krauss and Robert Scherrer imagine, “for these future astronomers, the observable universe will closely resemble the ‘island universe’ of 1908: a single enormous collection of stars, static and eternal, surrounded by empty space.”57 What Krauss and Scherer find frightening, first, is that the observations by these “future astronomers” will produce an “incorrect” vision of the universe: with no galaxies to see receding, they will not even know that the cosmos is expanding.58 Second, future astronomers will be profoundly alone in the universe. And third, after a long period of deception and isolation, even galaxies will start to unravel, and, as Michael Lemonick puts it, “all that will be left in the cosmos will be black holes, the burnt-out cinders of stars and the dead husks of planets. The universe will be cold and black.”59 Robert Kirshner, a member of one of the two teams that discovered dark energy in 1998, imagines the universe’s final scene as “lonely, dull, cold, and dark.”60 Theoretical physicist Brian Greene sees it as “vast, empty, and lonely.”61 And Brian Schmidt, who led the Nobel Prize–winning High Z Supernova Team, of which Kirshner was a member, calls this end-time scenario “the coldest, most horrible end to the universe I can think of. I don’t know—it’s creepy.”62
“So this, then, is the story of the universe,” writes the Search for Extraterrestrial Intelligence Institute’s senior astronomer Seth Shostak. “A Big Bang, a hundred billion years of light, life, and late-night television, and then an infinitude of nothingness. Am I getting through to you? Not a long time—not a really long time—but an infinitude. A flash of activity, followed by a never-ending darkness. Our universe is destined to spend eternity in hell, without the fire.”63
Something about this “creepy,” hellish, dark-energetic end of the world seems to have prompted physicists to rethink its beginnings. This is not to say that any specific cosmologist is directly motivated by existential panic; it is simply to mark the coincidence at the broadest level of new projections about the end of the universe, on the one hand, and new models of its beginning, on the other. In particular, as some physicists have projected the inexorable unraveling of this universe, others have raced to map a profusion—even an infinity—of others. Some theorists have even sought ways to get new cosmic beginnings out of the end itself: a burst of new worlds out of even the most lifeless, dark-energetic void.
But, again, the past decade’s fascination with the multiverse did not emerge out of nothing. Rather, most of these theories had been posited—and more or less ignored—decades beforehand. So what was so suddenly attractive about the multiverse? There are a number of ways to answer this question, but one possibility emerges clearly in the work of Alexander Vilenkin, an early theorist of “eternal inflation.” In the wake of the discovery of dark energy, “the long-term prospects for any civilization appear rather bleak,” he writes. “Even if a civilization avoids natural catastrophes and self-destruction[,] it will, in the end, run out of energy. The stars will eventually die, and other sources of energy will also come to an end. But now eternal inflation appeared to offer some hope.”64 And it did so by offering an infinite number of other universes in which life might emerge.
A Remedy for Fine-tuning
The most widely accepted multiverse scenario comes out of what is called “inflationary cosmology.” Alan Guth first posited the theory of inflation in the early 1980s, borrowing an all-too-familiar economic term to describe the exponential growth of the earliest universe.65 He offered inflation as a way to resolve a number of problems with standard big bang cosmology. The first problem, as Brian Greene puts it, was that “the big bang leaves out the bang. It tells us nothing about what banged, why it banged, how it banged, or, frankly, whether it really banged at all.”66 In its standard form, the big bang hypothesis could explain in dazzling detail the processes of the early universe from about
of a second onward. But there remained what Steven Weinberg admits was “an embarrassing vagueness about the very beginning”—an uncertainty as to what happened at “t = 0” to get the universe hurtling outward to begin with.67 As Paul Steinhardt and Neil Turok summarize this problem, “[T]he universe is simply assumed to have appeared out of nothing, filled with all kinds of exotic matter and energy, at nearly infinite temperature and density.”68 But where did all of this matter–energy come from, and how?
The second shortcoming of the standard big bang hypothesis was that it left unresolved the “flatness” and “horizon” problems, both of which concern the appearance of fine-tuning in the early universe. The “flatness” problem refers to the shape of the universe itself. As Alexander Friedmann showed in the early 1920s, space as governed by the laws of general relativity can take on three different topographies, depending on the amount of matter and energy in the universe. If the amount is greater than a “critical density” of “about six hydrogen atoms per cubic meter,” then the universe will have a positive curvature, like the surface of a sphere. If the amount of matter and energy in the universe is less than the critical density, then space will have a negative curvature, like a saddle or (to use a remarkably common illustration) a Pringles® potato chip. If, however, the mass–energy of the universe is equal to the critical density, “the equivalent of a raindrop in every earth-sized volume,”69 then space will be “flat” in three dimensions. Every indication over the past few decades has shown that the density of matter is almost exactly equal to the critical density, so space is nearly perfectly flat on large scales.70 The problem is that if a split second after the big bang the density had been at all lower or higher than the critical density, then the universe would have taken on either severely negative or severely positive curvature, the former spreading matter out too far to form any major structures and the latter drawing all matter together into a collapse. So, Greene explains, “some mechanism … must have tuned the matter/energy density of the early universe extraordinarily close to the critical density,” and as Martin Bucher and David Spergel charge, “the big bang theory offers no explanation apart from dumb luck.”71
The “horizon” problem refers to the striking thermal homogeneity of the early universe. Since the discovery of the CMB in 1965, physicists have marveled that regions too far apart ever to have been in contact (that is, regions that lie beyond one another’s cosmic horizons but within our own) nevertheless have almost identical temperatures. “So,” as Andrei Linde relates the thought process, “one could only wonder what made these distant parts of the Universe so similar to one another.”72 The first solution was to appeal to the “cosmological principle,” a neo-Atomist dictum that simply states “that the Universe must be uniform” in all directions.73 But to say that the universe is uniform because of a principle that makes the universe uniform is tantamount to saying, with Molière’s doctor, that opium puts people to sleep by virtue of its “dormitive property”;74 it is not really an explanation at all. Moreover, although the universe is uniform on the largest scales, it is not perfectly uniform; after all, there are galaxies in some places and leagues of dark space in others owing to slight thermal inhomogeneities in the early universe.75 How, then, are we to account for the extraordinary flatness, homogeneity, and inhomogeneity of the early universe, all of which allowed existence as we know it to exist?76
Once again, these problems are familiar, boiling down to the mystery of matter’s creation and distribution throughout the universe. Now, of course, one can always repeat the Cartesian–Newtonian solution and say that a skillful, intelligent creator-God made and scattered matter and energy with the values that God’s eventual creatures would need to emerge. Or one can posit an immanent cosmic principle to fulfill these functions, as Kant did with his “repulsive” or “centripetal” force and as Einstein did with his cosmological constant. But although the cosmological constant has been vindicated in recent years, it is not nearly powerful enough to perform these cosmogonic functions. So we need another repulsive force—a stronger, faster energy that Guth posited in the 1980s, calling it “inflation.”77
Inflation in its most basic form is a brief burst of insanely rapid universal expansion that kicks in right after the big bang—a hyperactively repulsive gravity that, “according to even conservative estimates,” blows the universe up by “a factor of 1,000,000,000,000,000,000,000,000,000,000 in [0].00000000000000000000000000000000001 second.”78 As Stephen Hawking and Leonard Mlodinow explain the process, “[I]t was as if a coin 1 centimeter in diameter suddenly blew up to ten million times the width of the Milky Way.”79 Because inflation happens so quickly, it irons out any positive or negative curvatures and leaves the universe flat. Moreover, because it expands space-time faster than the speed of light, inflation explains how regions that appear never to have been in contact could have “thermalized” (that is, reached the same temperature) before inflation flung them apart. And finally, as inflation stretches space-time outward, it stretches quantum fluctuations into slight temperature variations that then become the seeds of galaxies,80 solving all of the big bang’s fine-tuning problems at once. But this first formulation of inflation still did not explain the bang. In the years that followed the publication of Guth’s landmark paper in 1981, a number of theorists therefore set out to answer a number of unanswered questions—most pressingly, what got inflation going and what turned it off. In the process, they unintentionally opened the door to a slew of multiple universes.
Inflation and Infinity
Theorists working on inflationary cosmology collided with what they now call the “multiverse” from two directions. First, they discovered that the universe that inflation produces appears to be spatially infinite. Second, they discovered that once inflation gets under way, it does not seem to stop. These two insights opened, in turn, onto what mathematician Max Tegmark calls the “Level I” and “Level II” multiverses, respectively.81
Our visible universe is composed of the expanse of space-time that light has been able to travel since the big bang banged 13.8 billion years ago. Because space has been expanding since then (and accelerating for the past 5 billion years), the farthest visible object–events are now about 40 billion light-years away, even though they took place fewer than 14 billion light-years ago. The sphere 40 billion light-years in radius, centered wherever a given satellite is located, is called our “Hubble volume,” or “observable universe.” Now according to even the most standard cosmological models, there are regions of space-time beyond our Hubble volume; light just has not had enough time to reveal them to us (and if dark energy stays constant, it never will). So there is something beyond what we can see, and one of the central predictions of inflation is that this “something” is infinite.
Although a number of physicists and cosmologists contest this prediction and are currently seeking to establish the finitude of the universe, many others take it almost as given.82 They assume, moreover, that the infinite space beyond our Hubble volume is similarly filled with matter and energy: this contemporary articulation of the old “principle of plenitude” is now known as the cosmological principle.83 And as we will recall from the Atomists, Giordano Bruno, and even Nicholas of Cusa, the moment the universe is said to be both spatially and materially infinite, a number of remarkable conclusions follow. For Epicurus and Lucretius, for example, an infinite number of atoms with a finite number of shapes ensured not only that there were an infinite number of worlds “beyond the fiery ramparts” of our own, but also that some of them would be exactly like ours (see chap. 2, sec. “Fire and the Phoenix”). After all, if there is a limitless amount of material but a limited number of ways to configure it, then all possibilities will have to repeat; in fact, they will have to repeat an infinite number of times. Greene invokes the same principle when he argues that the universe is most likely infinite and that there are an unfathomably high but nevertheless limited number of arrangements that particles can assume. Sounding like a latter-day Lucretius, Greene concludes that “an infinite number of occurrences combined with a finite number of possible configurations ensures that outcomes are infinitely repeated.”84
The popular multiverse literature therefore almost always opens with a guided meditation along the following lines: imagine that somewhere out there, there is another “you” reading this book.85 The two of you have made all the same decisions in your lives, from college to shoes, except that just now she (or he) has stopped reading to check Facebook, whereas you have dutifully decided to read on. She reads a disturbing news story about a natural disaster and decides to jump on the next plane to join the relief effort, eventually changing careers forever, whereas you hold on to your current job and apartment. In the meantime, somewhere else “out there” another “you” has just gotten up to make the fourth cup of coffee that you have just decided you really should not have, while another is doing exactly what you are doing and, in fact, is just now wondering about the other “you” who is just now wondering about her. In an infinite universe, all these possibilities play themselves out, and each of them does so an infinite number of times.

Where are these other yous? As in Atomist cosmology, they are spatially arrayed, far beyond any possible connection to our world. Tegmark estimates that your first doppelgänger is nearer than
meters away: an unfathomable distance, but nonetheless finite. Even farther out, “about
meters away should be an entire Hubble volume identical to ours”—that is, an observable universe with the same distribution of matter, the same Milky Way, the same rings of Saturn, the same Brontosaurus that turns out to have been an Apatosaurus all along, and the same disturbing Twitter feed that mashes up quotations from Søren Kierkegaard with the wisdom of Kim Kardashian.86 And in between that world and ours, there are countless other Hubble volumes, some of them very similar to ours with a few minor differences, others completely different. This whole ensemble of universes constitutes what Tegmark calls the “Level I multiverse” and what Greene calls the “quilted multiverse” (figure 5.3). Like Cusa’s perspectival multiverse, this multiverse is composed of an endless and overlapping ensemble of observable universes, each of them centered around any given cosmic body and all of them issued forth from the same big bang nearly 14 billion years ago.
Inflation and Eternity
But we still have not accounted for the bang itself—in particular, for where it came from or what it was. Such an account began to emerge in the mid-1980s with the “new” and “chaotic” inflationary scenarios that developed the work Guth had begun.87 Although there are some significant mathematical differences between these two models,88 they are often grouped together cosmologically because they translate into very similar stories. The first major insight that the models share is that inflation takes place “outside” our universe—before the bang, as it were. And the second is that inflation is eternal; once it starts, it never ends.
Depending on the author, this story takes one of two very different points of departure. Alexander Vilenkin tends boldly, even gleefully, to proclaim that his account begins “from nothing!”89 As I have argued elsewhere, what he means by “nothing” is not nothing at all, but a vacuum, which according to quantum mechanics is not empty, but seething with “virtual particles” that flash into and out of existence.90 This vacuum, then, is Vilenkin’s primordial scene: a spaceless space that he himself calls “chaotic” and “foamlike”—neither nothing nor something, but a bustling fray of particles appearing and disappearing at random. One day, the story continues, one of these particles seems to have flashed into existence and then failed to flash back out, tunneling through the energy barrier that should have caused it to collapse and inflating instead to form space-time as we know it.91 Edward Tryon initially proposed this idea in 1970, apparently blurting out during a seminar on quantum mechanics, “[M]aybe the universe is a vacuum fluctuation!” As Vilenkin relates the story, “[T]he room roared with laughter,”92 yet a little more than a decade later Tryon’s weird idea would provide the basis for Vilenkin’s own weird idea: that the universe quantum-tunneled into being once upon a time.
In order to grow into a universe, this initial region of space-time would have been filled with inflationary energy, a repulsive gravity that expands the region exponentially at every moment. This state of renegade expansion is called the “false vacuum”—“false” because it is unstable and tends to decay into a lower-energy state, like the one in which we exist with our tiny cosmological constant. This false vacuum, incidentally, is where most other inflationary scenarios begin: with a rapidly stretching inflationary sea.93 Again, this state is unstable; regions of space-time cannot sustain this level of energy for long and so will soon “roll” down to a lower-energy state, forming “bubbles” or “pockets” of “true vacuum,” which is to say regions of space-time with a low-energy cosmological constant. Crucially, at the point of this roll-down, the excess energy is converted into matter in the form of radiation, which is flung into the newly stable pocket of space-time as “a hot fireball of elementary particles.”94 The end of inflation, in other words, is the big bang, providing the primordial plasma that gravity will eventually assemble into a universe.95 Inflation therefore fulfills both of the functions for which Descartes and Newton had to appeal to God: the creation and the distribution of matter in the universe.
In the meantime, although inflation has “turned off” in one particular region, it continues unabated (and, for Andrei Linde, jumps to even higher energy levels) elsewhere, sending space-time “outward” faster than the speed of light. In other words, as Guth and a host of others have shown, once inflation turns on, it never turns off.96 In fact, the false vacuum is always producing exponentially more of itself, relaxing into isolated bubbles of true vacuum here and there, but also sustaining an ever-growing sea of runaway space-time between them.97 In this “fractal” cosmology, then, “one inflationary universe sprouts other inflationary bubbles, which in turn produce other inflationary bubbles.”98 And because the process is eternal, inflation “produces not just one universe” and not just a lot of universes, “but an infinite number of universes.”99
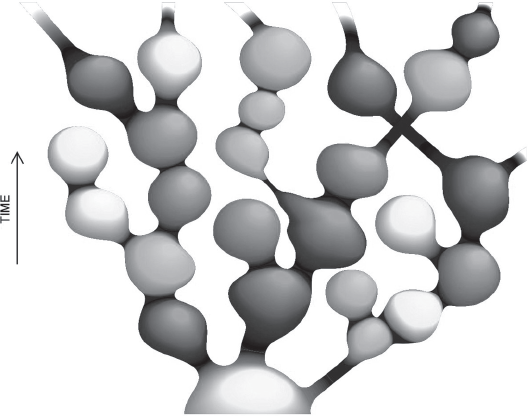
This infinite collection of universes, which Tegmark calls “Level II” and Greene calls the “inflationary multiverse,” looks more Brunian than Cusan—an infinite number of worlds are arrayed throughout infinite space, held apart by an ever-expanding “void” (figures 5.4 and 5.5). For nonspecialist audiences, the inflationary multiverse is often compared to “island universes” floating in a vast sea or to “gas pockets in a loaf of rising bread” or to holes in a “gigantic block of Swiss cheese.”100 Each island, pocket, or hole is a universe; the sea, bread, or cheese is the inflationary region that separates the worlds from one another. Unlike the Level I multiverse, each of these Level II universes arises out of different big bangs and, as such, might have very different “constants” from ours, including the strength of the electromagnetic force, the number of spatial dimensions, and the value of the cosmological constant (Λ). With a lambda value much smaller than ours, a new bubble would “expand for the shortest of time before collapsing again,” whereas with a large lambda, a bubble would “expand so fast that matter is spread far too thin for stars and planets ever to form. The overall result,” as John Gribbin explains, “would be a chaotic mess of bubbles all expanding at different rates in different regions of spacetime.”101
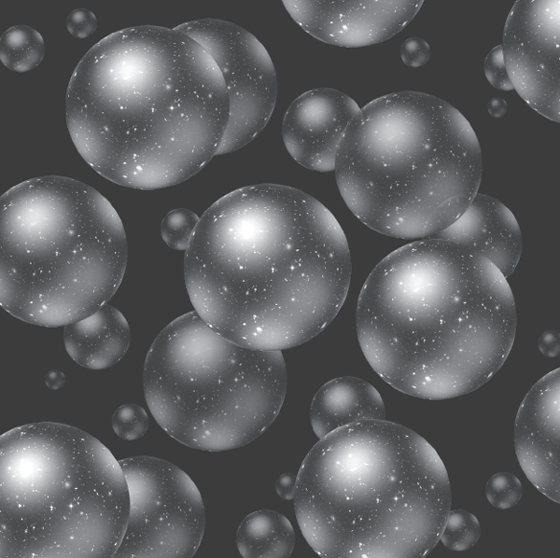
For the most part, these worlds will never come into contact with one another because the space-time between them is expanding faster than the speed of light. For the most part, worlds with a sustainable cosmological constant will live for a time and then, along Vilenkin’s account at least, suffer a strikingly Epicurean death by decay.102 But it is always also possible that a universe might suffer a more Democritean fate and collide with another universe before inflation has time to fling them apart, the “collision send[ing] destruction screaming through the walls of both bubbles.”103 Fascinatingly, though, such destruction might not be total; in fact, a cosmic collision might leave pockets of each universe intact, stamping a kind of thermal bruise on each of them. As we shall see in chapter 6 and “Unendings,” some cosmologists are currently examining the CMB for signs of this sort of a collision, which would provide invaluable evidence in support of the inflationary multiverse.104
All told, the scene looks like this: from an “outsider’s perspective” (assuming for a moment that it makes sense to speak of such a thing), each bubble universe appears as a bounded region within a larger multiversal expanse. Each universe, in other words, looks finite from the outside. But as noted in the preceding section, the view from inside one of these universes—ours, for example—seems to suggest that the universe is infinite. The explanation here is remarkable: just as Einstein’s theory of special relativity says that “rest” from one perspective looks like “motion” from another and that neither perspective is wrong,105 inflationary cosmology says that “each of the bubble universes appears to have finite spatial extent when examined from the outside, but infinite spatial extent when examined from the inside” and that neither perspective is wrong.106 At this point, we might do well to bring Cusa back into this neo-Atomist scene. For Cusa, the term contracted infinity was a way of expressing the puzzling truth that what looks infinite from the perspective of creation looks finite from the perspective of an infinite God (see chap. 3, sec. “End Without End”). For inflationary cosmology, the explanation is that the infinite time outside each bubble is converted into infinite space inside each bubble, so that from the perspective of the sea each bubble is spatially bounded, whereas from our perspective the universe goes on forever. And although, observationally speaking, “our” perspective is the only one we will ever have, neither view is more “correct” than the other.
In conclusion, then, a Level I multiverse—that overlapping set of worlds with all those copies of “you” doing an infinite number of identical, similar, and different things—can be found within each of the bubbles of the Level II multiverse, an infinite number of which contain particles and creatures like us and an infinite number of which do not. From the “outside,” each of these bubbles looks finite, but from the inside each looks infinite. It is as if a Cusan multiverse were contained within each of Bruno’s infinite worlds, and then the whole mess were subject to Cusa’s perspectivalism. Infinities here become nestled within infinities—or within finitude, depending on how you look at it.
As both Vilenkin and Linde have testified, the eternal inflationary scenario was barely noticed when it was first proposed; in fact, it is said that Guth fell asleep when Vilenkin first presented the idea to him.107 Others were more directly critical than Guth, accusing inflationary cosmology of looking more like metaphysics than science. In Vilenkin’s account, “[T]he main objection against [eternal inflation] was that it was concerned with the universe beyond the horizon[,] which is not accessible to observation.”108 Stephen Hawking remains a vocal critic of any effort to determine what happened “before” or “beyond” the bang; as he wrote in his mega–best seller A Brief History of Time (1988) and has reaffirmed in his recently co-authored book The Grand Design (2010), nothing that might have taken place before the big bang could have produced any “observational consequences” on the universe as we know it, so there is no use speculating about such events.109 Eternal inflation not only requires a robust pre–big bang scenario, but also generates numberless regions of space-time forever beyond our horizon. For these reasons, inflation was initially thought to create more problems than it solved; an infinite number of universes seemed a high price to pay for an explanation of the flatness and homogeneity of this one.
Just around the turn of the millennium, however, the fortunes of eternal inflation began to change. Vilenkin and his collaborator, Jaume Garriga, along with a number of other theorists, attribute this change to the discovery of dark energy in 1998.110 The perplexing smallness of the cosmological constant, the extent to which it seems improbably calibrated to enable life as we know it, seems far less mysterious if there are an infinite number of universes, with all possible values of lambda. Eternal inflation, as we saw in the introduction, suddenly looked like a promising way to redeem the anthropic principle, which in its strong form verged on theism and in its weak form could say little more than that “the world we observe must be consistent with our existence, insofar as we are observing it.” With a multiverse, the weak anthropic principle can become far more robust and can now proclaim along with the Atomists that, given an infinite amount of space, time, and material, every possible configuration is bound not only to emerge, but also to repeat. And so the universe is the product of neither wild improbability nor divine action. Thanks to the age-old pair of accident and infinity, “nature,” as Lucretius might say, is “rid of her proud masters.”111
For his part, Linde attributes this millennial inflationary turn to developments in string theory that caused it, independently of inflationary theory, to collide with its own multiple-worlds scenario. But these two sources are not totally separate; as Linde explains it, string theory’s multiverse emerged out of efforts “to solve the cosmological constant problem.”112 String theorists themselves would likely disagree, attributing the emergence of this scenario to the discovery of “fluxes” within string theory itself. But regardless of causation, the correlation is striking: the rise of multiverse theories at the dawn of the twenty-first century seems to have been the product of what political theorist William Connolly might call a “resonance machine” composed of inflationary cosmology, dark energy, and string theory.113
String theory is commonly said to be the most promising candidate for a “theory of everything”—that is, a theory that accounts for the universe simultaneously on the largest scales (general relativity) and on the smallest scales (quantum mechanics).114 According to string theory, subatomic particles are not “points,” but one-dimensional “strings,” whose vibrations constitute their mass. String theory also posits higher-dimensional objects called “branes” that can assume all kinds of shapes, or “topologies,” and that occupy up to nine spatial dimensions.
Until fairly recently, as Helge Kragh has explained, “string theorists … [had] hoped that the theory, when sufficiently developed and understood, would result in a unique compactification or in a ‘vacuum selection principle,’ from which the one and only vacuum state describing the universe would emerge.”115 They hoped, in other words, that string theory would eventually explain why the values of the cosmological constant, the gravitational constant, the nuclear and electromagnetic forces, and all the other seemingly fine-tuned parameters of fundamental physics have to be the way they are. What they discovered, however, was that string theory allows for a staggering number of possible “vacua” or “stable configurations of spacetime.”116 The most common estimates range from 10500 to 101,000 possible vacua, which means that according to string theory there are between 10500 and 101,000 different kinds of universe.117 These numbers, although unassimilably huge, are still finite, and so (as Epicurus realized millennia ago) they ensure the repetition of any given universe, including our own.118 All of this makes the fundamental parameters we observe seem a bit less impossible than they would otherwise appear. “In particular,” explains Guth, “the cosmological constant (e.g. the vacuum energy density) would be expected to have different values for different vacua”;119 in effect, the vacuum energy has had at least 10500 chances to obtain the wildly unlikely value it takes on in our universe.
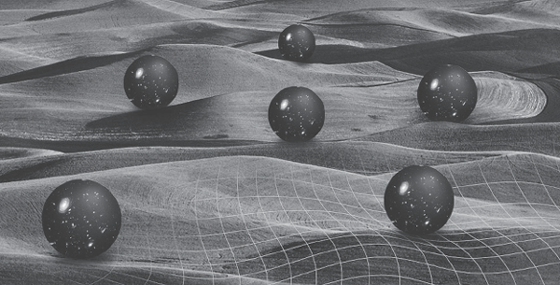
In 2003, Leonard Susskind proclaimed that with the discovery of these vacua solutions, string theory had reopened and redeemed the anthropic principle, calling its set of manifold vacua the “landscape” of string theory.120 Notoriously impossible to get one’s head around, the landscape is a mathematical representation of string theory’s 10500(ish) different dimensional compactifications, which is to say its different types of universes. It is usually represented as a kind of mountainous terrain, with each “valley” corresponding to one of the stable vacua (figure 5.6).
Such representations, of course, are two or, at best, three dimensional, a limitation that makes visual depictions rather comically impaired from the outset. Susskind leads his nonspecialist readers in the following thwarted meditation: “Try to imagine a space of 500 dimensions with a topography that includes 10500 local minima, each with its own Laws of Physics and constants of nature. Never mind.”121 If the landscape is a schematic representation of all possible universes, then which cosmology describes their actual generation? As Steven Weinberg has explained, there are a number of candidates—including the most recent incarnation of Stephen Hawking and Thomas Hertog’s “no-boundary” proposal, which we will encounter in chapter 6.122 But the cosmological model that is most commonly paired with the landscape is eternal inflation. Linde remarkably made this connection in the mid-1980s,123 but it seems that a broader acceptance of the idea had to wait for the “perfect storm” of eternal inflation, string theory, and the cosmological constant problem. If it is the case (as Guth, Raphael Bousso, and Joseph Polchinski seem to suspect and as Linde and Susskind wholeheartedly believe),124 that these two theories describe the same multiverse—or “megaverse,” as Susskind would have it, or “universe-of-mini-universes,” as Linde prefers—then each of the types of bubbles of inflationary cosmology would correspond to one of the 10500 (give or take a gazillion) valleys in the string theoretical landscape. And each type of universe, as long as the number is finite,125 would recur an infinite number of times.
Now a major problem posed by both the landscape and inflation multiverses is how to calculate the probability that any given type of universe will be generated—especially if any possibility can be said to be generated an infinite number of times throughout eternity. “Inflation plus the landscape” thus runs us up against the so-called measure problem: if the number of Type X universe is infinite and the number of all universes is infinite, then how do we calculate the probability that any given universe will be Type X? How is one to divide infinity by infinity?126 This, then, is the challenge for landscape and inflationary theorists alike: “to determine how these potential [inflationary] universes are distributed across the corresponding Landscape Multiverse.”127 Greene stages this task as a kind of heroic ordeal, explaining that “detailed maps of this mountainous terrain have yet to be drawn. Like ancient seafarers, we have a rough sense of what’s out there, but it will require extensive mathematical explorations to map the lay of the land.”128 But as far as Susskind is concerned, even before the details are fully worked out, the landscape has already done a great service: it has provided the ultimate solution to the fine-tuning problem and a vindication of the anthropic principle. Why is our universe the way it is? Because, the landscape tells us, “somewhere in the megaverse, the constant equals this number; somewhere else it is that number. We live in one tiny pocket where the value of the constant is consistent with our kind of life. That’s it! That’s all! There is no other answer to the question.”129
The Cost of Inflation
One of the earliest physicists to discover the eternal nature of inflation was Paul Steinhardt, a theoretical physicist and cosmologist who has gone on to become one of the theory’s most noteworthy critics. In a recent popular article, Steinhardt runs through the case for and against inflation, admitting that the theory is “powerfully predictive.”130 It accounts beautifully for the flatness and homogeneity problems, predicts the slight thermal variations imprinted on the CMB, and tends to be “in exquisite accord” with the latest data streaming in from the Wilkinson Microwave Anistropy Probe (WMAP) and Planck satellites, which measure the CMB with increasing precision as the years go on.131 For these reasons, inflation has become the most widely accepted theory of cosmic origins among physicists. But, Steinhardt reminds us, in the process of solving these problems and making these predictions, inflation comes with an “awkward corollary,” which is that it never turns off. And as we have just seen, the upshot of eternal inflation combined with string theory is an infinite number of 10500 (or more) different types of universe, arranged in some unvisualizable landscape and eternally separated by a ballooning expanse of useless space.
One cannot avoid the sense that Steinhardt harbors an aesthetic revulsion to this multiversal excess; in one interview, he calls it “a dangerous idea that I am simply unwilling to contemplate,” and he and Neil Turok warn that eternal inflation “seems likely … to drag a beautiful science toward the darkest depths of metaphysics.”132 As the latter quotation indicates, however, Steinhardt’s aesthetic revulsion stems from a commitment to a particular kind of scientific rigor. Here, he explains, is the problem: “[I]n an eternally inflating universe, an infinite number of islands will have properties like the ones we observe, but an infinite number will not.” And the minute this is said to be the case, he charges, the theory’s predictive power is completely undermined: “What does it mean to say that inflation makes certain predictions—that, for example, the universe is uniform or has scale-invariant fluctuations—if anything that can happen will happen an infinite number of times? And if the theory does not make testable predictions, how can cosmologists claim that the theory agrees with observations, as they routinely do?”133 The problem, as Steinhardt sees it, is that the theory designed to explain this universe has ended up explaining any universe, thereby undermining its most celebrated function. As for the additional claim that eternal inflation explains the fine-tuning of dark energy and the other constants, Steinhardt believes that the price of this explanation is simply too high. As he and Turok explain in their popular book Endless Universe, “[T]he two of us confess to being among those who are skeptical about the anthropic principle as a panacea for fundamental physics. The main problem is that it relies on a host of assumptions that cannot possibly be tested: the existence of the multiverse, the notion that different pockets have different physical laws … and so on.”134 Rather than relying on a host of extracosmic kosmoi to explain the one we are in, Steinhardt and Turok therefore set out to find a different story to tell.
The New Ekpyrotic Scenario
As Steinhardt and Turok recall, their new model was inspired by a lecture they invited Burt Ovrut to deliver to cosmologists on “M-theory,” which encompasses all previously unreconciled branches of string theory.135 The element that captured their imagination was not string theory’s slew of vacuum solutions, they say, but its idea of membranes—those up to nine-dimensional objects of varying topologies. For each of these “branes,” they learned, there is a corresponding “antibrane partner,” and this realization got them thinking.136 What if our universe is a three-dimensional membrane? What would its “partner” be? Where would its partner be? And what would happen if the two of them were to collide?
The resulting theory was first called the “ekpyrotic scenario,” intentionally named after the signature aspect of Greek Stoic cosmology.137 Since then, as details of the model have changed, it has been variously renamed the “cyclic model,” “new cyclic model,” and “new ekpyrotic model.”138 In the meantime, its reliance on string theory has weakened: one paper even considered abandoning the idea of branes before a follow-up paper reinstated them.139 But the best-known model looks like this: two membranes of three spatial and one temporal dimension are suspended in a fourth spatial dimension. Our observable universe—everything we can see and not see between here and 40 billion light-years in any direction—“lies on one of the branes, often called our ‘braneworld,’” explain Steinhardt and Turok. “It is separated by a tiny gap, perhaps 10–30 cm across, from a second ‘hidden’ braneworld…. All the particles we are familiar with, and even light itself, are confined to our braneworld. We are stuck like flies on flypaper, and can never reach across the gap to the ‘hidden’ world, which contains a second set of particles and forces with different properties from those in our braneworld.”140
The only forces that can cross the gap are gravity and dark energy, which keep the two branes separated during one stage of the cosmic cycle and draw them together during the next. As the branes are drawn together, the energy between them increases, and they gain speed before finally colliding, at which point they separate again, their energy converted into a mass of radiation flung outward on each brane. The collision, in other words, is the big bang.
On each brane, the model temporarily follows the course of the better-known big bang hypothesis, except without the inflationary energy. First, there is the big bang, then radiation, then gravity, then dark energy, but at this point when the standard model predicts the gradual unraveling of the world, the ekpyrotic model changes course. Dark energy does not continue to speed the cosmos outward forever, either dissolving it into a void or ripping it apart. Rather, after a trillion years, the energy density of dark energy begins to decay. Its outward force starts to reverse, drawing the two branes back together, another big bang bangs, “and the cycle begins anew” (figure 5.7).141
Here, then, we have a temporal rather than a spatial multiverse. One universe (composed of two partner branes) undergoes continual destruction and rebirth throughout eternity. Steinhardt and Turok, like their mid-twentieth-century predecessors, note the resonance with Hindu cosmology; in fact, they even map the two worldviews onto each other mathematically, showing that one full ekpyrotic cycle corresponds almost exactly to a year in the life of Brahma (around 3.11 trillion years).142 We may recall that the downfall of these midcentury models came at the hands of the Second Law of Thermodynamics: if entropy increases from cycle to cycle, then two problems ensue: first, a new universe will never begin in an ordered state comparable to the one before it, and, second, each cycle will last longer than the previous one, which means the cycles get shorter and shorter into the past, eventually reaching an initial singularity. The new ekpyrotic theorists avoid the first pitfall by pointing out that the problem for any new universe is not the amount of entropy, but the density of entropy. In the braneworld scenario, both branes increase in size from cycle to cycle, spreading the entropy across a larger surface area and decreasing the overall entropy density of each new universe.143 This solution may or may not also address the second problem, that of an absolute beginning, but Steinhardt and Turok seem untroubled by the outcome. The cycles may be past-eternal, or they may not be: “[O]ne could imagine the sudden creation from nothing of two infinitesimal spherical branes arranged like two concentric soap bubbles,” which would collide and expand until they became large and flat.144 Or at least Steinhardt and Turok could imagine such a thing.
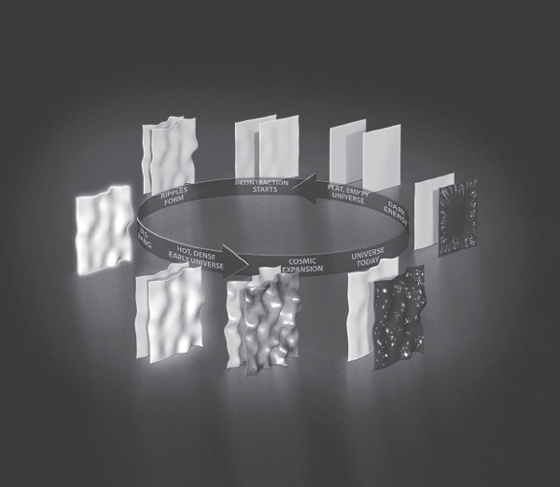
Either way, what is crucial for these cyclical theorists is that their model addresses all the concerns inflation addresses, but without introducing unconnected regions of space-time governed by differing laws of physics. The reason the universe is so flat after the bang is that it was flat before the bang: both branes remain large and extended throughout the cycle. The reason the CMB is so uniform is that dark energy empties the branes of all matter before the contraction that gives way to the bang. And the inhomogeneities arise from small quantum fluctuations that wrinkle the branes slightly as they draw near to each other: the places where the branes collide first are the “hot spots” where galaxies will eventually form. All this with no (spatial) multiverse and no anthropic principle: “[A]ccording to this view, the universe is a single, coherent entity that exists in a stable cycling state whose properties can eventually be understood as a consequence of the basic laws of nature.”145 The physical constants, in other words, remain constant in this model.
What we have in the ekpyrotic scenario, then, is a neo-Stoic rival to the neo-Atomist theory of inflation. Like the Stoics, the new Ekpyrotics insist that there is only one world. Like the Stoics, they maintain that the one world is tensionally interrelated by means of a substance that suffuses and governs it—the role that pneuma played for the Stoics is here filled by dark energy, which “regulate[s] the cycling,” acts both attractively and repulsively, and absorbs systematic shock.146 Like the Stoics, the new Ekpyrotics describe this immanent force by means of pantheological metaphors of creation, governance, and providence, telling us that “stars, galaxies, and the larger-scale structures observed in the universe today owe their existence to the period of dark energy domination in the previous cycle. And the dark energy dominating the universe today is preparing similar conditions for the cycle to come.”147 And finally, like the Stoics, they teach that this one world will be destroyed and re-created throughout eternity.
The new Ekpyrotics are notably reluctant, however, to indulge in any fantasies of eternal return. Nowhere in the specialist or popular literature is there a meditation on the infinite number of times that you have read this book before or that Socrates has taught Plato or that the same demon has stolen into your loneliest loneliness to proclaim you the same speck of dust. Nevertheless, they do project that in the model’s simplest form, “all the physical properties of the universe are the same, on average, from cycle to cycle.”148 A more complicated version of the theory entertains the idea that “some properties thought to be constants … could actually vary over long periods,” but they would do so by means of a slow, evolutionary process—not a haphazard “decision” among wildly different vacua.149 In short, although the new ekpyrotic model returns to the Stoics, it tempers this return with the “old” modernist efforts to avoid speculation about that to which we have no access and to explain physical constants as they are in this universe rather than as they might be in a multitude of other universes.
As it turns out, however, comparatively few theorists have been converted to the ekpyrotic cause.150 Many admit that the scenario accounts for the appearance of the CMB as well as inflation does, but even so they raise a host of objections to it.151 Some charge that the ekpyrotic model does not actually escape the problem of entropy at all. Others ask why there are only four large spatial dimensions or why there is only one braneworld and its partner. “Why stop at two branes?” asks John Gribbin. “The most exciting thing about M-theory, … the most compelling reason to take the idea of a multiverse seriously, is that it offers an infinite choice of possible worlds, not just one boring pair of cymbals clashing out the same old song.”152 And perhaps the most vocal critic of this cyclic model has been Andrei Linde, who a week after its first publication proclaimed ekpyrosis “a house of cards.”153 The problem, he charged, was the arbitrary starting point of two perfectly flat membranes. “If you start with perfection,” he conceded, “you might be able to explain what you see … but you still haven’t answered the question: Why must the universe start out perfect?”154 In recent years, Steinhardt and his colleague Jean-Luc Lehners have suggested that the universe might not start out “perfect” at all, but might keep cycling until dark energy forms it into a “large, flat, habitable universe.”155 But even this possibility remains too speculative for Linde and the majority of his colleagues.
During the first few years of this inflation–ekpyrosis debate, it seemed as though the argument would be easily arbitrated once the data were clear. Unlike inflation, whose repulsive force would cause gravitational waves (B-modes) to become imprinted on the CMB, the cyclic theory predicted that its big bang would not produce such waves. If, then, the European Space Agency’s Planck satellite were ever to detect these waves, the ekpyrotic theory would be ruled out; as Turok explained in 2007, their model had the scientific advantage of being falsifiable.156 In recent years, however, Steinhardt and Lehners have complicated this issue—perhaps because Planck’s increasingly refined results have still revealed no B-modes. In the new “new ekpyrotic” scenario, there is far more “instability” and destruction than in the original model (the early literature relies heavily on adjectives such as gentle, regular, slight, and regulated). In the new version, “an overwhelming fraction of the universe fails to make it through the ekpyrotic phase” and is simply incinerated at the collision. But as long as the preceding cycle undergoes a moderately long stage of expansion before collapsing, there will be “a sufficiently large patch of space” that can cycle successfully through the big bang. Everything else will turn to dust, but this one patch will survive the infernal bounce and go on to re-create the universe. “As with the mythical phoenix,” Steinhardt and Lehners conclude, “a new habitable universe grows from the ashes of the old.”157
In this revised scenario, which Lehners and Steinhardt claim has more right to the “phoenix” moniker than its predecessors, the less gentle, less regulated collision will produce gravitational waves, “but very weak and of a kind that may never be detected.”158 According to these new calculations, then, if B-modes were to be found, the discovery would neither disprove the ekpyrotic model nor confirm the inflationary model. So the neo-Stoic model is likely to remain an underdoggish rival to the neo-Atomist model as long as the former finds theorists with enough energy, enough willingness to be slightly overlooked, and enough distaste for the infinite bubbles of eternal inflation.
Alternative Cyclic Cosmologies
Such distaste has prompted the emergence of other cyclic models as well—lesser known than the ekpyrotic scenario (and, for the most part, far less respected), but stemming from equally fervent critiques of inflation. For example, Lauris Baum and Paul Frampton, admitting that their objections to inflation are “more aesthetic than motivated directly by observations,” have set forth a cyclical multiverse that is at once temporal and spatial.159 According to this model, dark energy is neither inflation’s cosmological constant (which retains a constant density) nor ekpyrosis’s quintessence (which decays over time). Rather, lambda in the Baum–Frampton scenario is what Robert Caldwell has named “phantom dark energy,” whose density increases with time.160 Instead of “simply” dissolving all matter into an ever-expanding void (the “big whimper” ending), dark energy becomes so powerfully repulsive that it splinters the universe into an immense number (1084 to 10103) of “patches” of space-time, sending them all on a course toward a final “big rip.”161 The patches are flung farther than light can travel between them, at which point dark energy empties each of them of all its matter. Each patch is eventually so distended and emptied that it “contains no quarks or leptons and certainly no black holes.”162 And then, just before the patch is shredded in an apocalyptic cosmic rip, it contracts, collapses, and bounces outward again, “reinflating in a manner similar to the big bang.”163
It is “similar” to the big bang, but it is not the big bang; in fact, as far as Baum and Frampton are concerned, there is no “big bang” at all insofar as the term connotes an absolute cosmic starting point. As one paper they coauthored with Shinya Matsuzaki puts it simply, “[T]ime never began.”164 Rather, the universe has been cycling from eternity, with the dark-energetic demise of one universe producing a slew of others—one for each causal patch that contracts and rebounds.165 Of course, these theorists admit, some patches that are insufficiently emptied out will “fail to cycle,” but the probability of at least one patch successfully cycling into a full-blown universe is 1.166 So the demise of each universe produces anywhere between 1 and 10103 new universes. Without positing multiple vacua or extra dimensions, the Baum–Frampton model branches outward like a tree as the cycles go on, “leading to an infinite multiverse” in both space and time.167
But there are yet other models. Using the framework of loop quantum gravity (the leading alternative to string theory),168 Martin Bojowald predicts “a universe collapsing as a result of overpowering gravitational attraction” and approaching what looks like a remarkably Empedoclean stage of “strife.” As the universe approaches the quantum level, Bojowald explains, it enters a state of “utter turmoil, chaos, and confusion,” or the so-called State of Hell that constitutes the loop quantum vacuum. Although “all forces struggle for dominance,” the eventual victor is gravity, which acts repulsively at the quantum level, “calms the seas, and lets the universe expand to vent its anger.”169 Then there is radiation, there is matter, and Empedocles’s stage of “love” returns for another round. As far as Bojowald is concerned, the advantages of this model over inflationary and string models are manifold: loop quantum gravity avoids initial and terminal singularities;170 it requires neither six extra dimensions nor 10500 extra vacua; and, in the words of Helge Kragh, “the repulsive force … is not introduced ad hoc.”171 Rather than positing a new kind of energy to account for the gravitational “push” (whether it be Kant’s centrifugal force, Einstein’s cosmological constant, quintessence, phantom dark energy, or inflation), loop quantum gravity needs only one force, which acts attractively at the classical level and repulsively at the quantum level. As Bojowald explains it, “[Q]uantum gravity [itself] … provide[s the] forces counteracting its own classical attraction.”172
Meanwhile, back in the string theory camp, Cristiano Germani and his colleagues have proposed a model they call the “Cosmological Slingshot” as an alternative solution to the flatness and horizon problems.173 According to this scenario, our world is one of a number of three-branes traveling through a “Calabi–Yau manifold,” an unstable ten-dimensional space with “spikes” and “throats.” As the universe falls into one of these “warped throats,” it collapses into a big crunch but then flies back up the throat and reexpands; “the kinetic energy of the brane is passed to matter fields,” and the universe cycles through the stages of radiation, gravity, and dark energy before slipping down the throat again and being re-created.174
Or there is Roger Penrose’s “conformal cyclic cosmology,” framed in opposition to inflation and to cosmologies arising from what he disparagingly calls “string theory culture.”175 Inspired by Maurizio Gasperini and Gabriele Veneziano’s “pre–big bang scenario” (but, Penrose is careful to reiterate, without their reliance on string theory),176 Penrose’s scheme is one in which the end of one universe becomes the beginning of the next so that “the universe as a whole is to be seen as an extended conformal manifold consisting of a possibly infinite succession of aeons.”177 Toward the end of one aeon, the accelerated expansion of the universe flings distant galaxies apart from one another. In the meantime, nearby galaxies circle one another and eventually collide. At this point, the black holes at their centers merge and produce “whacking big explosions,” which produce ripples in the fabric of space and time.178 These ripples from the end of one aeon appear as “families of concentric circles” imprinted on the new universe; in fact, Penrose and Vahe Gurzadyan claim that they have detected such circles on the CMB.179 This claim, it should be said, has found very little support; although other researchers agree that the circles are indeed detectable, they attribute them to quantum fluctuations in this universe—not to the influence of a previous universe.180 David Spergel summarizes a fairly common critique of Penrose: “[A] universe with dark matter, dark energy and inflation is bizarre enough—we don’t, however, get to detect circles from alternative universes.”181
But, of course, it all depends on how you define “bizarre.” Even the multiverse scenarios that look most outrageous from some perspectives seem to be perfectly simple—even parsimonious—from others.