4 Early Products in Agricultural Biotechnology
Two agricultural products that were in development in the 1980s gave the public its first glimpse of biotechnology’s early contributions to industrial farming. First, a genetically modified tomato with the trade name of Flavr Savr was promoted as a vine-ripened tomato that was less perishable and better tasting than its unmodified run-of-the-mill parental variety. Consumers were offered a new product and a new brand—the first whole-food product developed through biotechnology. The second product was a genetically engineered soil bacterium commonly called ice-minus bacteria, which was designed to prevent frost damage to crops.
The Flavr Savr tomato had a short commercial life as a marketed and labeled GE tomato and then as tomato puree. The GMO soil bacterium, which was designed and field tested to prevent frost damage in crops, was never commercialized as an agricultural product but nevertheless prompted considerable regulatory activity and public debate.
These two products, even in their failure, afforded the new agricultural biotechnology industry insights into the kinds of societal, market, and regulatory responses to genetically engineered products in agriculture that they would face in subsequent years. They were the product pioneers of a new biotechnology agricultural sector.
The Flavr Savr Tomato
Calgene was a West Coast biotechnology company that sought to apply new advances in molecular genetics to crop development with a focus on tomatoes, rape seed (canola oil), and cotton. The company was started in a garage in Davis, California, by a group of University of California biology professors led by Ray Valentine. Its most notable product was a slow-ripening and long-shelf-life tomato.
In 1987, Calgene researchers began investigating a tomato enzyme called polygalacturonase (PG) that dissolves the tomato’s cell wall, which consists of a complex set of polysaccharides called pectins. The breakdown of pectins, a key component of most terrestrial plants, is associated with the softening of ripe tomatoes. The role of PG in tomato softening during the ripening process was reported in 1982.1 The scientists questioned whether the expression of PG could be controlled in order to delay the rate at which ripening takes place.
Tomatoes were typically picked green from the vine so that they could be transported sometimes thousands of miles before they overripened and became unappealing to consumers. When they were ready to enter consumer markets, tomatoes were sprayed with a chemical that speeded up the ripening process and turned the tomatoes red.
Earlier, in 1978, scientists had discovered a new method of controlling protein synthesis called antisense technology. The basic process of protein synthesis consists of three stages: (1) DNA is copied into (2) messenger RNA (mRNA) (which is a single-stranded copy of the gene) by a process called transcription, where mRNA, with the help of transfer RNA (tRNA), serves as a template for the cell’s ribosomes to (3) synthesize polypeptide chains that form a protein, a process called translation. Briefly, the whole process, called the central dogma, is described as DNA → RNA → Protein (figure 4.1).
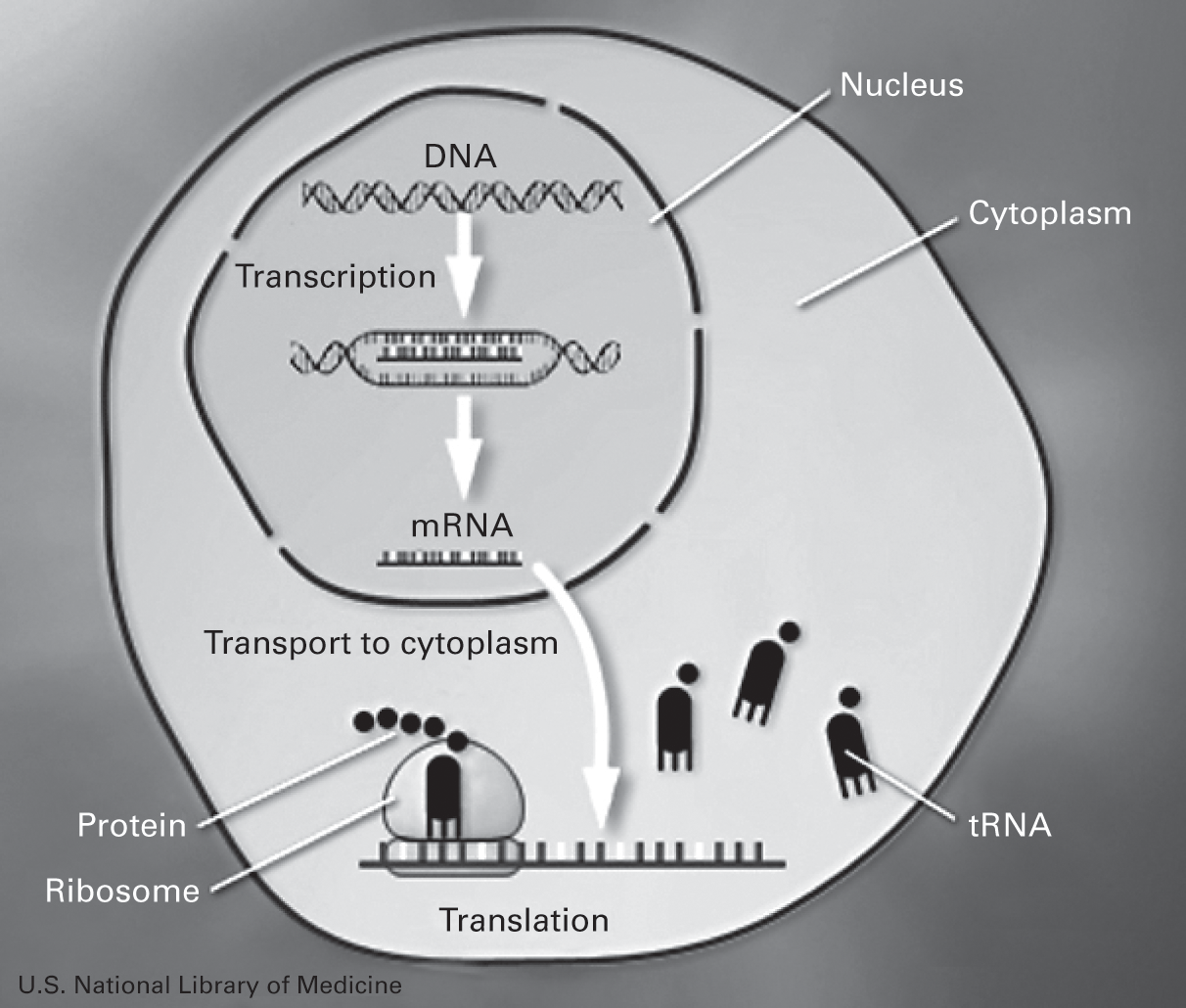
A canonical description of DNA transcription and translation in cells.
Source: U.S. National Library of Medicine.
The antisense method involves a molecule designed to bind to and interfere with a specific mRNA construct so that the protein synthesis is disrupted. In the case of the tomato, scientists were interested in disrupting the PG enzyme (protein), which is responsible for softening fruit. By reducing PG enzyme expression, the tomato could be allowed to ripen on the vine without softening. As previously noted, commercial tomatoes are harvested at the mature green stage. They are shipped long distance and ripened by treating them with ethylene for 12 to 18 hours at 20 degrees Celsius. Shipping tomatoes that are picked green, however, prevents the development of natural flavors and results in a tasteless tomato.
For the Flavr Savr tomato, Calgene scientists inserted an additional copy of the PG encoding gene but in a different orientation than the indigenous gene, flipping it upside down and backward.2 The mirror image of the PG gene codes for an antisense mRNA that is complementary and binds to the sense mRNA. This double-stranded mRNA blocks the expression of the correct mRNA. The insertion of the PG antisense gene essentially shuts off the PG enzyme and inhibits the ripening process.
The level of PG produced in the Flavr Savr tomato is as little as or less than 1 percent of the unmodified parental line. In other words, introducing a transgene in the plant that is a mirror image of a gene will code for an antisense mRNA, which blocks the expression of the correct mRNA and therefore when applied to PG reduces the amount of pectin-degrading enzyme.
With less PG produced, the tomato stays ripe and firm for a longer period of time. It can be picked red from the vine, which yields a tastier product than picking and shipping it green and then exposing it to treatment with the chemical ethylene.
The Calgene scientists produced a tomato that was alleged to be superior to the non-GM tomato in several ways. It had a longer shelf life, was promoted as tastier and of better quality, possessed equivalent nutrient content, and had higher viscosity, which was more desirable for processed juice and tomato paste.
A critical part of this process involves the creation of the DNA construct that includes the marker gene, the promoter, the transgene itself, and the termination sequence. For the marker gene, scientists used a gene from the bacterium Escherichia coli called kan(r), which encodes the protein APH(3ʹ)II that makes the plant cell resistant to the antibiotic kanamycin. Applying the antibiotic to the cultured cells allows scientists to tell which cells have the transgene of interest (only the ones that survive the antibiotic selection pressure). The promoter gene came from the cauliflower mosaic virus (CAMV 35s). The construct was inserted into a plasmid and through the vector Agrobacterium tumefaciens was inserted into tomato cells in a growth medium.
Calgene had no legal requirement to obtain regulatory approval for developing and marketing its genetically modified tomato. The U.S. Department of Agriculture’s Coordinated Framework for Regulation of Biotechnology (1986) set the roles of three agencies—the U.S. Food and Drug Administration (FDA), U.S. Department of Agriculture (USDA), and U.S. Environmental Protection Agency (EPA). The USDA’s oversight was over plant pests. Given that the genetically modified tomato was the first U.S. GM product commercialized, there was a heightened attentiveness to the potential risks. In October 1992, the USDA ruled that the PG antisense tomato lines were not plant pests and therefore did not require permits for field testing or transport. In 1995, the USDA added two additional genetically engineered lines to the determination of nonregulated status for Calgene’s Flavr Savr tomatoes.3
Belinda Martineau, a Calgene scientist who wrote First Fruit: The Creation of the Flavr Savr Tomato and the Birth of Biotech Food, discusses the types of considerations that the company faced in doing a risk assessment of its product: “The concern over unintended changes centered on the fact that we had no control over where our genes went into a plant’s DNA. They could be integrated harmlessly or smack dab in the middle of an existing gene, disrupting that gene’s function. If that disrupted gene was involved in the formation of an important vitamin, for example, levels of that particular vitamin could be dramatically reduced.”4
Calgene requested a consultation from the FDA on the Flavr Savr tomato, a decision that it made in order to gain consumer approval for the product. The company submitted safety data to the FDA on the use of the kan gene as a marker for its tomato. One concern was whether after the gene and its expressed protein were in the tomato, could they find their way into bacteria in the human gut. If that were to occur and the bacteria were to become pathogenic, they would be resistant to the therapeutic use of the antibiotic kanamycin. The FDA and a number of commentators considered the possibility to be remote. Calgene’s petition for the kan gene was approved by the FDA in May 1994 after the company presented various worst-case scenarios.5 According to Nina Federoff and Nancy Marie Brown, “When Calgene brought it to the FDA in 1992, the tomato was subjected to $2 million [or $3.4 million in 2016 dollars] worth of testing done by Calgene.… In a public meeting the FDA scientists brought the results of their extensive and sophisticated chemical analysis to a panel of external advisors.”6
Prior to entering the market, Calgene sponsored a number of studies to determine whether the antisense gene complex affected any other parts of the tomato besides ripening. Matthew G. Kramer and Keith Redenbaugh note “that the expression of the antisense PG gene affects only the composition of pectin in the fruit.… The gene has no effect on levels of vitamins and nutrients, on production of potential toxins (tomatine), on taste, on non-pectin related processing traits, on horticultural traits (growth form, time to flowering, time to fruit set, etc.), fruit pH and acidity, and fruit color and size.”7
Calgene had engaged with the FDA for five years seeking approval for its antisense tomato, during which time it undertook laboratory experiments, conducted field tests, and produced analytical papers. Finally, on May 18, 1994, the FDA concluded that the Flavr Savr tomato had not been significantly altered compared to nontransgenic varieties. Roger Salquist, CEO of Calgene, commented on the long-awaited approval: “The event of last week may have been the single most important step in establishing this new industry.”8
Beginning in May 1994, the Flavr Savr tomato was marketed in California and Illinois. It was sold under the MacGregor brand and labeled as a genetically engineered product, the first voluntarily labeled GM crop. At the time, there was growing competition for tastier, farm-fresh tomatoes, including hydroponically grown varieties and others green harvested for better transport. In that stiff competition, the success of Calgene’s tomato depended on how well it performed during shipment.
Belinda Martineau describes her first experience with the genetically modified tomato compared with its nonmodified strain: “Both groups of tomatoes were left at room temperature and observed over time. After 3 to 4 weeks the nongenetically engineered tomatoes were noticeably shriveled and rotting, while the Flavr Savr™ tomatoes looked and felt essentially no different than first picked.”9
But after the tomatoes emerged from the greenhouse and entered the distribution system, problems emerged. One of Calgene’s scientists reported on the results of the company’s transport management: “Contrary to the company’s early expectations, the Flavr Savr gene could not keep vine-ripened fruit firm enough to be packed and transported like green tomatoes.”10 High production costs, poor growing conditions in Florida, steep competition, and company debt led to the demise of the Flavr Savr tomato, despite the fact that the tomato’s genetically designed property of slow softening was successful (although some noted that the taste was not superior). Calgene spent more money getting the tomatoes to market in good shape than it charged in the grocery store—an unsustainable business plan. By 1996, Flavr Savr tomatoes were removed from the consumer market in the United States. They were not approved for sale in the European markets, although a puree made from genetically engineered tomatoes had a short success in the United Kingdom during 1996 to 1999. Public attitudes had turned against GE products, which dramatically affected the market share of GE tomato puree and its adoption in major UK food markets.
Molecular breeding involving antisense technology was a small step in the move toward genetically engineered crops. No new genes were introduced into the tomatoes: the gene for one enzyme was removed and inverted. Yet questions were being asked. Would there be any other effects from the insertion of the antisense PG gene? Although the FDA did not require those questions to be answered, Calgene needed to ensure that it would not face any unexpected outcomes before it took the tomato to market and that its rigorous premarketing review would make the product acceptable to skeptical stakeholder groups. Ironically, the Flavr Savr tomato’s short commercial life precluded it from being tested in the national consumer market.
A Microbe That Protected Crops from Frost
In areas of the country prone to spring frosts before the fruit and vegetable harvest, like the orange groves of south Florida or the strawberry fields of northern California, freezing temperatures can inflict significant damage to crops. Frost is one of nature’s unpredictable pests. To save their crops from frost damage, farmers have used a variety of methods, some of which can be environmentally detrimental, labor intensive, and costly. All these methods have the same goal—to keep the temperature of the frost-sensitive plants above the temperature at which frost forms. These methods have included using wind machines that mix warmer air with cooler air, burning fuel that heats the air around the crops, watering the soil to release heat to the frost-forming droplets coating the leaves of the plants, and creating artificially generated fog that shields the plants from heat loss. One of the methods is akin to fighting fire with fire. When water droplets on the plants are beginning to freeze, the farmer sprays water on the plant: “The latent heat of fusion, released when water freezes to form ice, maintains the ice-water mixture on leaves at 0°C. This mixture will remain at 0°C as long as sufficient water is continuously available to freeze.”11 Ironically, the crops are destroyed not by the temperature per se but by the ice crystals that interfere with the plant’s metabolism.
In 1974, a scientific team at the University of Wyoming published a paper titled “Ice Nucleation Induced by Pseudomonas syringae” in which they concluded that the soil microorganism may play a role in the production of ice nucleation that occurs in nature.12 Building on that work, a group of scientists at the University of California at Berkeley came up with a novel approach for mitigating frost damage in crops. P. syringae, which is ubiquitous in agricultural fields, has a protein complex in its outer membrane that provides the nucleation site for ice crystallization. The Berkeley scientists discovered that P. syringae had one particular protein that seeded ice formation. In 1982, Steven E. Lindow and his colleagues observed that higher concentrations of P. syringae on leaf surfaces were associated with warmer freezing temperatures. These bacteria were called ice nucleation active (INA): “Water in plant tissues can be supercooled to –5°C, without harming the plants, yet when the ice nucleating bacteria (INA+) are present, ice formation can begin at about –1°C.”13 The four degrees can be a significant difference in frost damages.
Two approaches were initially taken to apply the knowledge of ice nucleation for frost protection. The first involved eliminating INA+ bacteria on plants by locating a virus that could kill the bacteria. With no INA+ bacteria, there will be no ice nucleation. That work was supported by University Genetics Company of Norwalk, Connecticut, and the Frost Technology Corporation. But the method proved too difficult to gain results.
The second approach adopted by the Berkeley group used gene splicing methods to remove the genes responsible for the ice nucleating proteins. In other words, they created an ice-minus form of P. syringae. Their idea was to spray high concentrations of ice minus on the crops and thereby displace the INA+ bacteria. Lindow worked with Advanced Genetic Sciences to bring the technology into a commercial application.
In 1982, Lindow and his colleagues at UC Berkeley in collaboration with Advanced Genetic Sciences petitioned the Recombinant DNA Molecule Advisory Committee (RAC) of the National Institutes of Health (NIH) to field test ice minus. On June 1, 1983, NIH authorized the field test, but litigation delayed the testing of this genetically modified frost protectant for another year. Because this was the first environmental release of the genetically modified bacteria, there were immediate reactions from environmental groups that questioned the safety of the product. Ice minus was classified as a pesticide by the EPA on the basis that frost was considered a plant pest. This designation played a major regulatory role in the first field tests and gave regulatory oversight to the EPA. The EPA’s role began in 1982, and it took five years of reviews and court decisions before the first field tests took place in Brentwood, California, in April 1987 under intense media attention.
The principal risk concerns that environmental activists and communities raised were the effects that the ice-minus bacteria might have on precipitation patterns. It was feared that widespread use of the bacteria could cause the ice minus to waft into the atmosphere, cause cloud droplets to form ice crystals, and alter natural rainfall patterns. In response to the concerns, Monterey County, California, passed an ordinance prohibiting field tests in the county. During the first field trial, the EPA monitored movement of the ice-minus bacteria to assess atmospheric risks. None had been identified. The now defunct Office of Technology Assessment (OTA) reported on the scenario of reducing atmospheric concentrations of ice nuclei affecting rainfall: “Seeking to assess the likelihood of this scenario, OTA commissioned two analytical studies by groups taking slightly different approaches to the problem. Both groups made assumptions to produce a worst case scenario. They both concluded it is unrealistic to expect any significant negative impact on global climatological patterns from large-scale agricultural applications of ice-minus bacteria.”14
The engineered form of ice minus was found to occur in nature at very low frequencies. Under the laws in effect at the time, the release of the natural form of ice minus would not fall under regulation. Meanwhile, Advanced Genetic Sciences merged with the company DNA Plant Technology to form DNAP: “DNAP’s selection and marketing of a nongenetically engineered organism for frost control, was an interesting overall strategy to bypass the complex rules associated with the introduction of genetically engineered organisms.”15 The new company focused on using naturally occurring ice-minus strains for developing several formulations of Frostban but later drifted from frost control to disease control.16
Frostban, even with naturally selected ice-minus strains, was not a profitable product. Estimates of its profitability were downplayed.17 Ironically, it was the natural bacteria, ice plus (of which there was a plentiful supply), that became profitable for inducing snow production in ski resorts. No risks were confirmed with the use of Frostban. The case study illustrates the process of creating regulations on the spot because the EPA was operating on a regulatory framework developed for chemical pesticides and not genetically engineered biological agents.
These two bioengineered products, the Flavr Savr tomato and Frostban, are emblematic of developments to come. Improving the taste and extending the shelf life of a tomato were benefits to the consumer as well as the distributor. The next generation of genetically engineered crops displayed traits that benefited growers, such as insect and herbicide resistance. Frostban raised questions about the environmental release of bioengineered organisms, foreshadowing genetically modified salmon, crops with built-in bacterial toxin genes, or gene-drive technology, where gene-edited mosquitoes were genetically designed to resist malarial infection.
These early products taught scientists about the slow adjustment that society makes to new food technology and agricultural processes. One cannot compare the speed of society’s adaptation to digital technology to that of its adaptation to food technology. These early cases also reveal differences between cultural and technological forms of risk assessment. Public skepticism toward new products or technologies is not usually resolved by a laboratory experiment. The public must see that bioengineered products have benefits over existing products and come to believe that those benefits outweigh the risks or uncertainties. These early cases suggest that people are more inclined to believe in agencies of government that are independent of the manufacturing sector and that they are not reluctant to trust scientists who play a central role in evaluating risks but who do not have a profit-making interest in the bioengineered products they evaluate for society.
Notes
1. Gregory A. Tucker and Donald E. Grierson, “Synthesis of Polygalacturonase during Tomato Fruit Ripening,” Planta 155 (1982): 64–67.
2. Each gene in the plant cell consists of two strands (a double helix)—the sense strand and the antisense strand. Only one strand is used to make mRNA. If the cell has two DNA coding sequences, one the mirror image of the other, then the cell will make an mRNA molecule, ordinarily single stranded with a double-stranded segment. When part of the mRNA molecule forms a duplex with some antisense mRNA (where the sense mRNA is complementary to and bonds with the antisense mRNA), the plant’s cell machinery is unable to recognize the sense mRNA molecule (it sees it as a foreign molecule) and thus prevents the translation process to synthesize the PG enzyme. Stuti Giupta, Ravindra Pal Singh, Nirav Rabadia, Gaurang Patel, and Hiten Panchal, “Antisense Technology,” International Journal of Pharmaceutical Sciences Review and Research 9, no. 2 (2011): 38–45.
3. Animal and Plant Health Inspection Service, U.S. Department of Agriculture, “Addition of Two Genetically Engineered Tomato Lines to Determination of Nonregulated Status for Calgene, Inc.,” Federal Register 60, no. 145 (July 28, 1995): 38,788–38,789.
4. Belinda Martineau, First Fruit: The Creation of the Flavr Savr Tomato and the Birth of Biotech Food (New York: McGraw-Hill, 2001), 113.
5. Matthew Kramer, Rick Sanders, Hassan Bolkan, Curtis Waters, Raymond E. Sheeny, and William R. Hiatt, “Postharvest Evaluation of Transgenic Tomatoes with Reduced Levels of Polygalacturonase: Processing, Firmness and Disease Resistance,” Postharvest Biology and Technology 1, no. 3 (1992): 241–255; Matthew G. Kramer and Keith Redenbaugh, “Commercialization of a Tomato with an Antisense Polyglacturonase Gene: The Flavr Savr™ Tomato Story,” Euphytica 79 (1994): 293–297.
6. Nina Federoff and Nancy Marie Brown, Mendel in the Kitchen: A Scientist’s View of Genetically Modified Foods (Washington, DC: Joseph Henry Press, 2004), 175.
7. Kramer and Redenbaugh, “Commercialization of a Tomato with an Antisense Polygalacturonase Gene.”
8. Martineau, First Fruit, 187.
9. Martineau, 4.
10. Belinda Martineau, “Food Fight,” The Sciences 41, no. 2 (2001): 28.
11. Steven E. Lindow, “Methods Preventing Frost Injury Caused by Epiphytic Ice-Nucleation-Active Bacteria,” Plant Disease 67, no. 3 (1983): 327–333.
12. Leroy R. Maki, Elizabeth L. Galyan, Mei-Mon Chang-Chien, and Daniel R. Caldwell, “Ice Nucleation Induced by Pseudomonas syringae,” Applied Microbiology 28, no. 3 (1974): 456–459.
13. R. M. Skirvin, E. Kohler, H. Steiner, D. Ayers, A. Laughman, M. A. Norton, and M. Warmund, “The Use of Genetically Engineered Bacteria to Control Frost on Strawberries and Potatoes: Whatever Happened to All That Research?,” Scientia Horticulturae 84 (2000): 181.
14. Office of Technology Assessment, U.S. Congress, New Developments in Biotechnology: Field-Testing Engineered Organisms (Washington, DC: U.S. Government Printing Office, May 1988), 95.
15. Skirvin et al., “The Use of Genetically Engineered Bacteria to Control Frost on Strawberries and Potatoes,” 187.
16. Skirvin et al., 184.
17. John Love and William Lesser, “The Potential Impact of Ice-Minus Bacteria as a Frost Protectant in New York Fruit Production,” Northeastern Journal of Agricultural and Resource Economics 18, no. 1 (1989): 26–34.