PALEONTOLOGISTS, scientists who study fossils, base their understanding of the history of life on the fossil record, which comprises all fossils discovered as well as those awaiting discovery. The fossil record of dinosaurs indicates that they existed for about 160 million years. During that time, many kinds of dinosaurs evolved. Key to understanding this evolution is determining the family tree, or genealogy, of dinosaurs. Which dinosaurs were closely related to each other, and which were only distant relatives? Answering these questions requires some understanding of the principles of evolution and phylogeny. Knowing how scientific names are given to dinosaurs or to groups of dinosaurs requires an understanding of the basic ideas and methods of biological classification.
Evolution, simply defined, is the origin and change of organisms over time. Today, biologists and paleontologists concluded that evolution occurs in the specific way first formulated by Charles Darwin (1809–1882).
In his book On the Origin of Species by Means of Natural Selection (1859), Darwin argued that organisms adapt to the environments in which they live. In other words, each organism has a specific way of living and interacting with its environment. When the environment changes, those organisms that are better able to cope with the changed environment are more successful—which, in Darwinian terms, means they reproduce more—than those organisms less able to cope with the change. The most successful organisms are thus “selected for,” and those least successful are “selected against,” in the jargon of what is called natural selection. Another phrase that has been used to describe this is “survival of the fittest,” where “fittest” refers to the most successful organisms: those producing the most offspring.
FIGURE 2.1
Natural selection can lead to the evolution of larger dinosaurs. (© Scott Hartman)
In Darwin’s view, certain organisms are more successful than others when faced with environmental change because of the variation that occurs in any population of organisms. We see this variation most easily by recognizing that no two people are alike, and the same is true in any population of animals or plants. So, when the environment changes, some individuals in a population are better able to cope with the change than others. Which organisms are better able to cope, however, is not something that the organisms themselves control, because they cannot anticipate what kind of environmental change might occur.
For example, in a population of one species of dinosaurs, we would expect to find a range of body sizes (
figure 2.1). If climate were to change to favor larger dinosaurs in the population, the larger variants would be selected for. Because they would reproduce more successfully than the smaller dinosaurs, we would expect the dinosaurs to become larger in the next generation, provided that the trait of larger size could be passed on from one generation of dinosaurs to the next. In this way, larger dinosaurs might evolve by the process of natural selection.
Evolution by natural selection is also called “Darwinian” evolution. In Darwinian evolution, the evolutionary history of a group of organisms is diagrammed by a family tree (or genealogy) of populations undergoing natural selection. The family tree emphasizes the fact that there are ancestors, descendants, and other relationships among a group of organisms. Because organisms change as a result of natural selection, Darwinian evolution can also be described in Darwin’s own phrase as “descent with modification.”
The family tree of a group of evolving organisms is also termed its
phylogeny, from the Greek words for “tribe” (
phylum) and “birth” (
genos). On a phylogeny, each branch, or each bundle of branches with a common stem, is called a
clade (
clados is Greek for “branch”), whereas a horizontal slice through a phylogeny is called a
grade (
figure 2.2). When a new kind of organism appears, we speak of
origination, and a new clade or segment of a clade is added to the phylogeny. When a kind of organism disappears, we speak of
extinction. When one clade splits into two we speak of
divergence; the evolution of similar features in two unrelated clades is called
convergence. The populations of organisms shown on a phylogeny are usually assigned to groups called
taxa (singular:
taxon). For example, each different kind of dinosaur in the phylogeny of dinosaurs is a dinosaur taxon, and these taxa can be grouped into larger dinosaur taxa based on their relationships to each other. When we speak of how taxa are related to each other, we are referring to their
phylogenetic relationships.
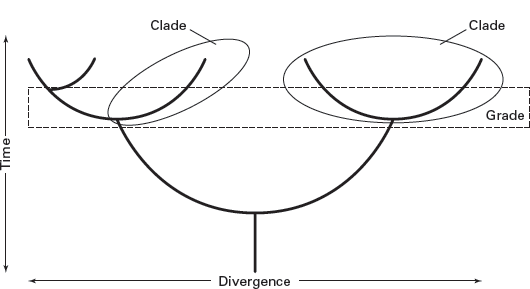
FIGURE 2.2
A phylogeny is an evolutionary family tree consisting of distinct clades (branches). A horizontal slice through a phylogeny is a grade. (Drawing by Network Graphics)
A phylogeny is a family tree, or genealogy, of taxa. How do paleontologists construct a phylogeny of a group of extinct taxa like dinosaurs?
For more than a century, the most common method was to consider the time span and overall similarity of the fossils of taxa as keys to their phylogenetic relationships. This method, called stratophenetic, from the words for “layer” (of rock) and “population” (of organisms), identifies ancestral taxa as those older than descendant taxa that resemble their ancestors closely in one or more features (
figure 2.3). Critical to a
stratophenetic phylogeny is the notion that the differences between the ancestor and its descendant are not so great as to seem implausible given the time interval between when the ancestor and descendant lived.
The problem many paleontologists have with stratophenetic phylogeny, especially those who study dinosaurs, is that it makes a great assumption about the completeness of the fossil record: the ancestor will be represented by fossils older than the fossils of the descendant. In theory, this would be the case, but in practice, the fossil record of many taxa, especially dinosaurs, is very incomplete. Because of this, we can almost never be certain when older fossils represent the ancestors of younger fossils, and we cannot assume that we have discovered all the ancestors and descendants in a phylogeny.
FIGURE 2.3
A stratophenetic approach to phylogeny identifies ancestors and descendants as time-successive, similar taxa. This diagram of skulls of horned dinosaurs is a stratophenetic phylogeny. (© Scott Hartman)
Because the known fossil record of dinosaurs lacks many ancestors and descendants, most paleontologists who study dinosaurs no longer use the stratophenetic method to construct phylogenies. Instead, they use a method that makes fewer assumptions about the completeness of the fossil record. This method is called
cladistics, and the resultant
cladistic phylogeny is called a
cladogram (
figure 2.4). The phylogenies of dinosaurs presented in this book are cladograms. Cladistics is the dominant method of phylogeny reconstruction used by vertebrate paleontologists.
A cladogram does not incorporate information about the time ranges of taxa. Instead, it is based only on the similarities of taxa. But neither overall similarity nor just any similarities are used to construct a cladogram. Those similarities of value to cladistics are those features that are
evolutionary novelties: inherited changes from previously existing structures. Cladistics argues that two taxa are closely related when they share evolutionary novelties. Taxa sharing the most such novelties are most closely related and are shown on the cladogram as diverging from a common ancestor (see
figure 2.4). In cladistic terms, these taxa form a
monophyletic group and share two or more clades with a single ancestor. In contrast, groups lacking a common ancestor on a cladogram are termed
polyphyletic (see
figure 2.4).
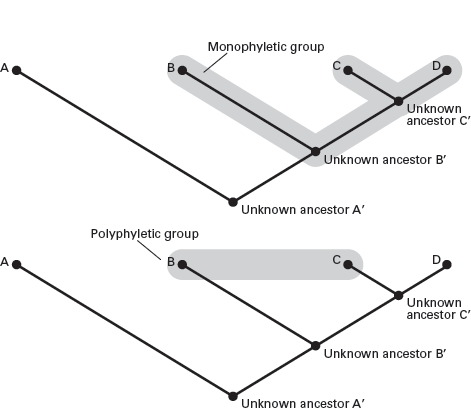
FIGURE 2.4
A cladistic phylogeny is called a cladogram. A monophyletic group consists of all the branches that share a stem, whereas a polyphyletic group is formed by uniting branches with different stems. (Drawing by Network Graphics)
A good example of a cladistic phylogeny is provided by trying to construct a cladogram of the three taxa trout, horse, and whale (
box 2.1;
figure 2.5). Based on many similarities, we might conclude that a trout and a whale are more closely related to each other than either is to a horse. But, if we focus on evolutionary novelties that distinguish mammals, such as the horse and whale, from bony fishes, such as the trout, then the cladogram that identifies the horse and whale as members of a monophyletic group is well founded. These evolutionary novelties include warm-bloodedness, hair, and giving live birth, which are features shared by horses and whales but not by trout.
Thus, evolutionary novelties allow us to distinguish a monophyletic group on the cladogram for whales and horses distinct from the clade for trout. But, how do we identify the evolutionary novelties to be used in constructing cladograms? In other words, which features shared by two taxa are evolutionary novelties and which features are not? No simple answers to these questions exist, but we can get a feel for how evolutionary novelties are identified by examining the theoretical basis for their identification.
When we view the origination and evolution of a taxon, we can see that it must inherit some features from its ancestor. The inherited features can be identified as
primitive. But, the features that arise for the first time in a new taxon—its evolutionary novelties—are thought of as
derived. These derived features, not the primitive features, unite the organisms into a group of closely related organisms. But, they do so only if the evolutionary novelties in question arose only once. Evolutionary convergence occurs when evolutionary novelties arise more than once in separate taxa not descended from a single close ancestor. For example, the wings of bats and of birds, though similar evolutionary novelties, arose in two taxa with very different ancestors and thus demonstrate evolutionary convergence. Convergence presents the greatest threat to arriving at the correct cladistic phylogeny of a group of taxa.
Once we understand the principles and methods behind cladistics, we should be able to construct a cladogram for any taxon, including the dinosaurs. Here, we will construct a basic cladogram of dinosaurs, birds, and nondinosaurian “reptiles.” To do so, we are asking the question, which of these taxa are most closely related to each other?
Because we have to start somewhere, let’s begin with some long-standing ideas, treating them as reasonable assumptions with which to direct our cladistic efforts. These ideas are that reptiles are descended from amphibians and that dinosaurs are descended from some other group of reptiles. This allows us to identify the sprawling posture of most reptiles, in which the limbs are positioned at the sides of the body, as a primitive feature of reptiles inherited from their ancestors, the amphibians. The upright posture of dinosaurs, in which the limbs are positioned under the body, thus must be an evolutionary novelty of dinosaurs with respect to their reptilian ancestors. Birds share an upright posture with dinosaurs, so this shared evolutionary novelty (if it did not evolve convergently) suggests that birds and dinosaurs are more closely related to each other than either is to nondinosaurian reptiles. We thus can construct a cladogram in which nondinosaurian reptiles form one clade and birds plus dinosaurs the other (box
figure 2.1). For the sake of convenience, we can indicate the evolutionary novelty shared by birds and dinosaurs (upright posture) on the cladogram.
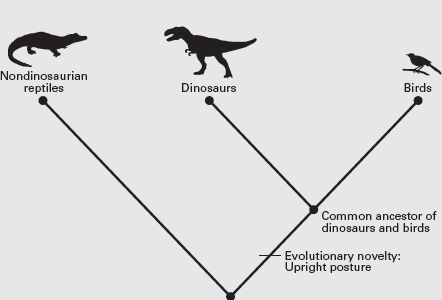
BOX FIGURE 2.1
This cladogram indicates that birds and dinosaurs are more closely related to each other than either is to nondinosaurian reptiles. (Drawing by Network Graphics)
Once we have constructed a cladogram, we can add information to this phylogeny to turn it into a
phylogenetic tree. The information we typically add is a geological time scale on the vertical axis and an indication of which taxa may have been ancestors and which may have been descendants (
figure 2.6).
FIGURE 2.5
This cladogram indicates that horses and whales are more closely related to each other than either is to trouts. (Drawing by Network Graphics)
FIGURE 2.6
A cladogram can be turned into a phylogenetic tree by making its vertical axis into a geologic time scale and indicating possible ancestors and descendants. (Drawing by Network Graphics)
Cladistics makes no assumptions about the completeness of the fossil record; it simply tries to identify closely related taxa by their shared evolutionary novelties. However, cladistics does not lack problems. Some question the method itself, arguing that it is a method of identifying clades by clustering character states to identify clades that runs contrary to our understanding of the evolutionary process (
box 2.2). Other problems arise from convergence, when a shared evolutionary novelty thought to unite two taxa turns out not to be that at all (
figure 2.7). Also, not all paleontologists may agree that a particular feature is an evolutionary novelty and not a primitive feature inherited from an ancestor. This leads to debate and disagreement among paleontologists attempting to construct cladistic phylogenies of extinct organisms. As we shall see in this book, such debates and disagreements are taking place over certain aspects of dinosaur phylogeny.
Cladograms of dinosaurs are generated by computer programs. The computer algorithm used is a kind of cluster analysis that analyzes a matrix of character states created for a group of taxa. The cluster analysis groups the taxa (identifies clades) by shared derived similarities of the character states. In other words, those taxa with the most shared derived similarities are clustered to form clades (
box table 2.2).
However, in 1999, University of California at Davis paleontologist Geerat Vermeij pointed out a serious problem with such computer-assisted cladistic analyses. Vermeij noted that a well-accepted conclusion about the evolutionary process is that if the evolution of animals takes place by a branching phylogeny, then evolution produces clades that are genetically distinct. This means that evolution within one clade cannot be affected genetically by other clades. However, cluster-analysis cladograms are based on all the character states included in the data matrix. This means that the character states are treated as interdependent, not independent, and therefore that the identification of a given clade by the cluster analysis is affected by the character states of the other clades.
This is most easily seen when taxa are added or removed from a character matrix and the shape of the cladogram changes, which often happens. In such a case, the cluster analysis is not identifying clades that are genetically independent of each other. Thus, it does not produce clades that mirror the well-accepted evolutionary process.
What to do? Criticisms of Vermeij’s observations have been few and have not challenged his central point. What we need is a method of phylogenetic analysis that identifies clades that are genetically independent of each other. At present, dinosaur phylogeny is wholly cladistic, so the phylogenies of dinosaurs presented in this book are cladograms. Clearly, a new or modified cladistic approach to produce phylogenies consistent with evolutionary theory is needed.
BOX TABLE 2.2
This character matrix lists taxa of hadrosaurs and the scored character-states of some features of their skulls. For example, character 6 is “skull with helmet-shaped crest” and scored as no crest (0) or crested (1). Such character matrices are the basis of computer-generated cladograms.
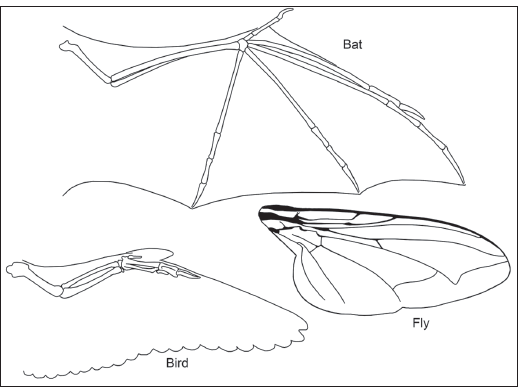
FIGURE 2.7
Convergence can produce evolutionary novelties in distinct clades that do not indicate close relationship. The wings of birds, bats, and flies are superficially similar but evolved in distinct clades. They are a classic example of evolutionary convergence. (© Scott Hartman)
Constructing a phylogeny based on shared evolutionary novelties is the fundamental basis for classifying taxa. This is because the biological classification of organisms is the grouping of them into taxa that share an ancestry. In other words, closely related taxa are placed in the same group so that the classification reflects their phylogenetic relationships.
Thus, the principal information paleontologists use to classify extinct organisms is their phylogeny. Based on their phylogeny, organisms are grouped into categories called taxa, and those taxa receive names. For the classification of animals, living and extinct, the categorical system used is a hierarchy first formulated in the eighteenth century by
Carolus Linnaeus (1707–1778), a Swedish naturalist. A set of international rules governs the method of naming the categories (taxa) (
box 2.3).
The International Code of Zoological Nomenclature spells out the rules by which the scientific names of animals are constructed. A central rule is the principle of priority. This rule mandates that the oldest correctly proposed scientific name for an animal has priority over later names proposed for that animal. In other words, if an animal received a proper scientific name in, say, 1900, and a second name was properly proposed for the same animal in 1980 (mistakes like this do occur!), the name proposed in 1900 should be used because it has priority. The name proposed in 1980 is then identified as a synonym of the 1900 name and should not be used.
The dinosaur generic names Apatosaurus and Brontosaurus provide a good example of the principle of priority. Paleontologist Othniel Charles Marsh coined the name Apatosaurus (deceptive lizard) in 1877, for gigantic dinosaur hip and backbones found in Colorado. In 1879, Marsh named an almost complete gigantic dinosaur skeleton from Wyoming Brontosaurus (thunder lizard). Marsh believed Apatosaurus and Brontosaurus were different kinds of dinosaur, so his proposal of two different names made sense. But, comparisons many years later revealed that the bones from Colorado and the skeleton from Wyoming belonged to the same kind of dinosaur. So, Brontosaurus is a synonym of Apatosaurus, which is the correct scientific name for this type of dinosaur. Despite this, Brontosaurus, which is a much more colorful name for this gargantuan dinosaur than is Apatosaurus, gained wide use, especially in popular books. Indeed, many people continue to use the name Brontosaurus—it even appeared on a stamp issued by the United States Postal Service in the 1980s—although the name is technically incorrect.
The rules in the
International Code of Zoological Nomenclature also cover the details of how to spell the scientific names of animals. These names must take Latin endings, and many of them have Greek or Latin roots. The genus and species names are always italicized. Such rules may seem a bit arbitrary, but they ensure uniformity in the scientific naming of dinosaurs and other animals, which facilitates communication among scientists worldwide. So, even though a dinosaur may be discovered in China and described by a Chinese paleontologist in an article written in Chinese, the dinosaur’s scientific name will consist of two Latinized words (
box figure 2.3). This makes it possible for those paleontologists who do not read or write Chinese to understand and use the dinosaur’s name.
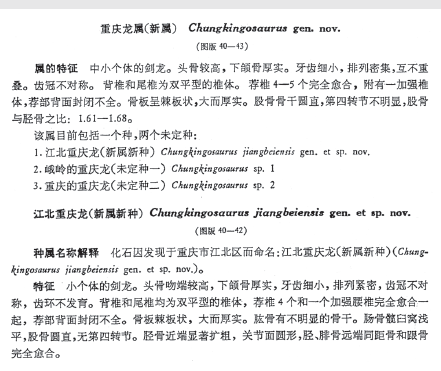
BOX FIGURE 2.3
This portion of an article describing a new dinosaur from China follows the International Code of Zoological Nomenclature by naming the new genus and species in Latinized words. (From Z. Dong et al. 1983. The Dinosaurian Remains from Sichuan Basin, China. Beijing: Science Press)
The
Linnaean hierarchy is a system of classification in which smaller (lower) taxa are nested into larger (higher) taxa (
figure 2.8). The categories of this classification extend from large and very inclusive to small and very exclusive, and each category has a category name. Thus, a phylum is a very large category in the Linnaean hierarchy. It contains one or more classes, and each class contains one or more orders and so on. The name assigned to a taxon follows the international rules that mandate the endings and forms of Latinization of most names (see
box 2.3). For example, the rules specify that
family names must end with the suffix “-idae.” So, the name of the family containing
Tyrannosaurus and closely related dinosaurs is Tyrannosauridae.
The name of a species of organisms, the lowest rank in the Linnaean hierarchy, always has two parts and is called a binomen. These are the genus (plural: genera) name followed by the species (plural: species) name. Thus, the name of the species to which all readers of this book belong is Homo (the generic name) sapiens (the trivial name), a Latin name that literally means “man the wise.” Another way to explain this is to say that living humans belong to the genus Homo and to the species Homo sapiens. The specific name sapiens cannot stand alone as the name of a species, which must be a binomen—therefore Homo sapiens.
The international rules of zoological nomenclature set standards for naming taxa that allow all scientists worldwide to name taxa in a consistent way so as to facilitate communication (see
box 2.3). But, because different ideas exist about the phylogenetic relationships of many organisms, the process of constructing classifications from phylogenies is full of disagreements that are useful in an active science. Once it comes time to name taxa, however, the rules that govern their naming ensure uniformity in the construction of the names and their endings. Nevertheless, the naming of taxa is a creative enterprise in which biologists and paleontologists are free to base the name on some attribute of the organism or organisms to be named or on anything else (
box 2.4).
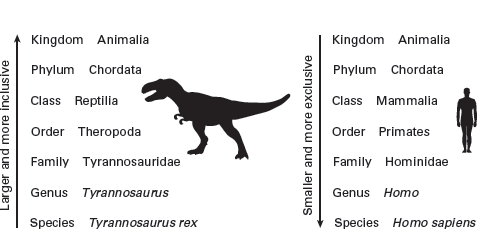
FIGURE 2.8
The Linnaean hierarchy groups organisms into progressively more inclusive groups. The major categories in the hierarchy—kingdom, phylum, class, order, family, genus, species—can be easily memorized with this mnemonic: “King Phillip Can Order Fresh Green Salad.” (Drawing by Network Graphics)
The International Code of Zoological Nomenclature describes the rules by which the names of dinosaurs are constructed. But, just what goes into those names is left to the creativity of the paleontologist(s) coining the name. Some dinosaurs are named after a distinctive anatomical feature. A good example is Diplodocus, which means “double beam,” in reference to the double-beamed chevron bones beneath the dinosaur’s tail. Other dinosaurs are named for the places where they were found. Tuojiangosaurus takes its name from the Tuo Jiang, a river in Sichuan Province, China near the discovery site. And, a few dinosaurs are named after people, such as Piatnitzkysaurus, named for Alejandro Piatnitzky (1879–1959), a Russian-born Argentinian geologist. However, it is most often the species name that is named after people, such as Diplodocus carnegii, named after multimillionaire and museum patron Andrew Carnegie.
Many dinosaur names are based on Greek or Latin roots, especially those named after an anatomical feature. But, this need not be the case, and there are a variety of unusual dinosaur names based on other linguistic root words. A good example is Khaan, a Cretaceous theropod from Mongolia. The genus name is from the Mongol word for “lord.” An unusual dinosaur name based on a person’s name is Drinker, a tiny Jurassic ornithopod. This dinosaur was named for paleontologist Edward Drinker Cope. (Drinker was the maiden name of Cope’s mother.) Another unusual name is Irritator, a Cretaceous theropod from South America, named after the state of mind of the paleontologists who named it. Most unusual and difficult to pronounce is Nqwebasaurus, a theropod from Jurassic–Cretaceous boundary strata in South Africa. This name is taken from the Bushman language of southern Africa and includes a click sound characteristic of the language. Thus, it is pronounced N-(click with tongue)-kwe-bah-SORE-us.
More traditionally constructed names can also be quite creative. Thus, there is
Bambiraptor, from the Upper Cretaceous of North America. This tiny theropod was named after the Disney cartoon character Bambi. Also, there is
Colepiocephale, a Late Cretaceous pachycephalosaur from North America (
box figure 2.4). This name was constructed from the Greek roots
colepio (knuckle) and
cephale (head), a perfect name for a dome-headed dinosaur!
BOX FIGURE 2.4
This is the small (about 13 centimeters long) skullcap of the Cretaceous pachycephalosaur Colepiocephale. (Courtesy Robert M. Sullivan)
As an example, place yourself in the shoes of paleontologist Henry Fairfield Osborn, who, in 1905, was presented with the huge skeleton of a meat-eating dinosaur found in Montana. In the Linnaean hierarchy, dinosaurs belong to the phylum Chordata, animals with a flexible cord or rod running down the middles of their backs. Within Chordata, dinosaurs belong to the subphylum Vertebrata, in which the rod is segmented into vertebrae. The class Reptilia, vertebrates that lay hard-shelled eggs and have scaly skin and limb structures for fully terrestrial locomotion, includes the dinosaurs. Reptilia is one of several classes in the subphylum Vertebrata. Dinosaurs can be more precisely classified within Reptilia as members of the infraclass Archosauria (the “ruling reptiles,” discussed in
chapter 4). Most meat-eating dinosaurs belong to an order called Theropoda (see
chapter 5).
But, Osborn’s new meat-eating dinosaur differed from all other known theropods, so he had to name a new genus and species for this huge predator. The name he coined, Tyrannosaurus rex, was constructed in accordance with the rules of zoological nomenclature. It is Latin for “king” (rex) of the “tyrant lizards” (Tyrannosaurus), an appropriate and colorful name for what was then considered to be the largest land-living predator of all time. In the following year, 1906, Osborn created the family Tyrannosauridae for this dinosaur, because he realized it was quite different from most other dinosaurs in the order Theropoda.
Paleontologists view evolution as taking place at two levels. Evolution at the species level, or microevolution, is the evolution of populations of organisms that results in the origination of new species. Evolution above the species level, or macroevolution, is the origination and evolution of taxa larger than species, including genera, families, and orders, and may take millions of years. Macroevolution also refers to the origin of major adaptive features.
To decipher microevolution from fossils requires a large number of fossils that are close to each other in geologic time. Only with such a dense fossil record can paleontologists document the variation and small changes of extinct organisms during relatively short intervals of geologic time (100,000 years or less)—the types of changes that lead to the origination of new species.
In contrast, to study macroevolution does not require such an extensive fossil record. A good example of a macroevolutionary problem relevant to dinosaurs is the origin of birds. Fossils of dinosaurs and those of the earliest birds provide extensive insight into the origin of a higher taxon, the birds (class Aves), from the dinosaurs, as will be discussed in
chapter 15. This is the case even though a dense fossil record of the evolutionary changes from a species of dinosaur to the first bird species remains to be documented.
The fossil records of many shelled invertebrates (animals without backbones, such as clams) and of some mammals are extensive enough to allow paleontologists to study microevolution. But, the fossil record of most dinosaurs is not. Dinosaur fossils are rarely abundant enough or close enough to each other in time to allow paleontologists to study the microevolution of dinosaurs. Instead, dinosaur fossils do document the macroevolution of some of Earth history’s largest and most bizarre animals.
Dinosaur fossils thus are evidence of the evolutionary diversification of one of the most successful groups of land animals to have existed on Earth. This diversity can best be understood as a variety of adaptations to different environments and ways of life. Dinosaur extinction ended a complex and prolonged episode of evolution.
1. Evolution is the origin and change of groups of organisms over time. It can also be described by Darwin’s phrase as “descent with modification.”
2. Darwinian evolution occurs by natural selection. This means that variants in a population of organisms better adapted to their environment will reproduce more successfully than less adapted variants.
3. The stratophenetic method of phylogeny reconstruction requires a much more complete fossil record than exists for dinosaurs. Therefore, most paleontologists who study dinosaurs do not use this method.
4. The cladistic method of phylogeny, which consists of constructing cladograms, is favored over the stratophenetic method. Cladistics identifies closely related taxa as those that share evolutionary novelties.
5. Problems with using the cladistic method arise from evolutionary convergence and difficulties in identifying features as evolutionary novelties.
6. Biological classification groups organisms into taxa according to their phylogenetic relationships.
7. The names of taxa are constructed according to international rules.
8. The fossil record of dinosaurs is best applied to problems of macroevolution.
binomen
biological classification
clade
cladistic phylogeny
cladistics
cladogram
convergence
Charles Darwin
derived
divergence
evolution
evolutionary novelty
extinction
family
fossil record
genus (plural: genera)
grade
Linnaean hierarchy
Carolus Linnaeus
macroevolution
microevolution
monophyletic group
natural selection
origination
paleontologist
phylogenetic relationship
phylogenetic tree
phylogeny
polyphyletic
polyphyletic group
primitive
principle of priority
species (plural: species)
stratophenetic phylogeny
synonym
taxon (plural: taxa)
1. Explain the central ideas behind Darwinian evolution.
2. Why do paleontologists who study dinosaurs favor cladistics instead of the stratophenetic method of phylogeny reconstruction?
3. Why does convergence pose a problem to cladists? What are other potential problems in constructing cladistic phylogenies?
4. Construct a cladogram for the three taxa: frog, dog, and cat. What shared evolutionary novelties led you to construct the cladogram?
5. What is the basis of biological classification?
6. What is the principle of priority and how is it applied to the scientific names of dinosaurs? What problems are alleviated by applying this principle?
7. What are the strengths and weaknesses of the dinosaur fossil record for studying evolution?
Benton, M. J. 2014. Vertebrate Palaeontology. 4th ed. Hoboken, N.J.: Wiley-Blackwell. (College textbook on the evolution of all vertebrates)
Futuyma, D. J. 2013. Evolution. 3rd ed. Sunderland, Mass.: Sinauer. (College textbook on evolution)
International Commission on Zoological Nomenclature. 2000. International Code of Zoological Nomenclature. 4th ed. London: International Trust for Zoological Nomenclature. (The international rule book for the construction of the scientific names of animals)
Prothero, D. R. 2007. Evolution: What the Fossils Say and Why It Matters. New York: Columbia University Press. (Explains the basics of evolution and its relationship to the fossil record)
Prothero, D. R. 2013. Bringing Fossils to Life: An Introduction to Paleobiology. 3rd ed. New York: Columbia University Press. (College textbook on all aspects of paleontology)
Wiley, E. O., and B. S. Lieberman. 2011. Phylogenetics: Theory and Practice of Phylogenetic Systematics. Hoboken, N.J: Wiley-Blackwell. (A complete introduction to cladistics)