We live in a world where most of the attention gets grabbed by the carnivores—and mammals are no exception. We have TV shows entitled “Fangs” but none (alas) called “Molars,” and the hoofed mammals are often regarded as little more than fodder.
—Christine Janis, 2003
After rodents and bats, the third-largest group of placental mammals is the ungulates, or hoofed mammals. Hoofed mammals make up about a third of the genera of living and extinct mammals, and nearly all large-bodied herbivorous mammals are ungulates. Most people are familiar with the common domesticated animals that provide us with food and services, such as horses, donkeys, cows, sheep, goats, pigs, as well as camels and alpacas, and know of more exotic hoofed mammals in the zoo or wild, such as rhinos, giraffes, antelopes, and hippos. (If elephants are members of Afrotheria, they may not be ungulates, but we will discuss these large hoofed mammals in this chapter anyway).
But the diversity of hoofed mammals of the past is much greater than the limited selection that survives today, with twice as many extinct families and genera. These include a wide array of hornless rhinoceroses, including the indricotheres, which towered over elephants as the largest land mammals that ever lived; bizarre chalicotheres, which were related to tapirs but looked like horses, except that they had claws like a ground sloth and knuckle-walked on their long forelimbs like gorillas; a great variety of North American camels, none of which had humps, but some of which did great impersonations of gazelles and giraffes; a number of extinct giraffes, none with long necks; pigs with horns; elephants without tusks that looked more like tapirs; and last but not least, an incredible array of transitional fossils that show how whales evolved from terrestrial hoofed mammals. All of this is well preserved in the fossil record because most ungulates are large-bodied and heavy-boned animals that tend to fossilize well.
Until recently, most of their history was not well understood or well documented. Part of the problem was logistical: the huge collection of fossil ungulates in the American Museum of Natural History in New York has still not been fully published since it became available for study in the 1970s. This collection occupies a separate wing of the museum, with 10 floors of storage and labs and offices and includes a whole storage floor of mastodonts and mammoths, a whole floor of North American rhinos, a whole floor of North American camels, a whole floor of horses, and three other floors of all the rest of the mammals. I was fortunate to arrive there as a graduate student in 1976 and got to work on many different groups of mammals. Many of these new collections contain cabinet after cabinet of undescribed complete skulls and skeletons of many species that had previously been known only from isolated teeth and jaws. For example, I did a lot of research on horses, peccaries, and camels (which is still ongoing), but my major task was documenting the long history of North American rhinos. This group had not been updated in a century, but I published a comprehensive monograph on the rhinos after more than 20 years of work (Prothero 2005).
Another part of the problem was conceptual: early work on many groups of ungulates focused too often on trying to find primitive ancestors and link them to descendants, ignoring shared derived characters. When cladistics came along, it made the study of ungulates much more rigorous, especially when cladistic analyses were completed on many of the major groups (see Prothero et al. [1986] on rhinos; Prothero et al. [1988] on the ungulates; and the chapters in Prothero and Schoch [1989] on perissodactyls and in Prothero and Foss [2007] on artiodactyls; and Prothero [2016] for the most recent information). Part of the problem had been a paraphyletic “wastebasket” group, the “Condylarthra,” which had been used to cluster any archaic ungulates that were not members of the living orders. With the aid of cladistics, this wastebasket has been broken up, and anyone who still uses the archaic and obsolete term “condylarth” (such as creationists) reveals their ignorance of the current state of research.
We can trace ungulates back as far as 85 million years ago in the early Late Cretaceous to fossils known as zhelestids from Uzbekistan (Archibald 1996). By the latest Cretaceous (67 million years ago), there were primitive ungulates known as Protungulatum that had many distinctive features, such as larger body size than any other latest Cretaceous mammal, more rounded cusps on the teeth, and a distinctive ankle region. After the extinction of the nonavian dinosaurs, the ungulates quickly dominated the habitats on the ground beneath the trees and radiated very rapidly in the Paleocene, so they are by far the most common fossils in Paleocene strata from the Bighorn Basin of Wyoming or the San Juan Basin of New Mexico. Most of these archaic ungulates (once called condylarths) are members of extinct groups that look nothing like the living hoofed mammals and were only distantly related to them (fig. 14.1). By the late Paleocene, we find the first fossils that clearly represent the origins of the major living orders of ungulates. These include Radinskya from the Paleocene of Mongolia, the primitive relative of the odd-toed perissodactyls, or horses, rhinos, and tapirs; Phosphatherium, Eritherium, Daouitherium, and Minchenella from Africa and Asia, the earliest members of the elephant-mastodont clan; Diacodexis and Dichobune, the earliest members of the even-toed hoofed mammals, or artiodactyls; and the mesonychids, which are distantly related to the whales. By the early Eocene, all of these ungulate groups were evolving rapidly into many different families and genera, most of which are now extinct. This story is so detailed and interesting that we can review only a few highlights in a chapter like this, but I recommend you take a look at my new book, The Princeton Field Guide to Prehistoric Mammals (published by Princeton University Press in 2016).

FIGURE 14.1. The evolutionary radiation of the hoofed mammals or ungulates. (Drawing by C. R. Prothero; after Prothero 1994b)
The geologic record of the ancestry of the horse is one of the classic examples of evolution.
—William Diller Matthew, “The Evolution of the Horse: A Record and Its Interpretation”
Let us start with the most familiar example of mammalian evolution, the origin of the horse (figs. 14.2 and 14.3). This is one of the oldest cases used to support evolution in the fossil record and still one of the best—and therefore the most distorted and misrepresented by the creationists. Shortly after Darwin’s book was published, fossil horses in Europe were connected into an apparent evolutionary series by Thomas Henry Huxley, French paleontologist Albert Gaudry, and Russian paleontologist Vladimir Kowalewsky. Then, in 1876, Huxley made a trip to North America, where he visited the amazing collections of fossil horses at Yale University amassed by crews working for the pioneering paleontologist Othniel C. Marsh. Huxley soon realized that the few European fossil horses were occasional immigrants to the Old World, not a continuous lineage that evolved in Europe, and that most of horse evolution had taken place in North America. Marsh pulled out drawer after drawer of specimens for Huxley, completely documenting the stages in the transition from tiny four- and three-toed early Eocene horses up to the modern Equus. Huxley was so amazed that he threw out his planned lecture notes and used Marsh’s specimens for his featured lecture instead. This early research on horses continued until it culminated in the famous book on horse evolution published by Matthew (1926), quoted in the epigraph above (fig. 14.2).

FIGURE 14.2. The evolution of horses as it was portrayed a century ago when there were relatively few fossils. The overall trend through time is clear: larger size, longer limbs, reduction of side toes, development of a longer snout and larger brain, and especially the development of higher-crowned cheek teeth for eating gritty grasses. However, a century of further collecting has shown that horse evolution is a more complicated bushy branching tree, rather than this oversimplified linear sequence. (After Matthew 1926)
FIGURE 14.3. A modern view of horse evolution, emphasizing the bushy branching nature of their history, as many more fossils have been found and new species named. However, the overall trends toward higher-crowned teeth (shown by the symbols for browsing leaves or grazing grasses), larger size, longer limbs, and reduction of side toes are still true. (Drawing by C. R. Prothero; after Prothero 1994b)
In general terms, these century-old diagrams are still valid. Horses did start as tiny beagle-sized animals with four toes on their front feet and three on their hind feet, low-crowned teeth for eating soft leaves, and relatively small brains and short snouts. These early Eocene horses have long been known as Eohippus (but that name is invalid for most of them) and Hyracotherium (but Hooker [1989] showed that Hyracotherium is a member of a native European group known as palaeotheres, not a true horse). Froehlich (2002) analyzed the North American fossils in detail and found that the old name Eohippus is only applicable to one of the species, E. angustidens. Instead, many of these early Eocene horses belong to Protorohippus, while others are assigned to a variety of genera, including previously proposed names such as Xenicohippus, Systemodon, and Pliolophus, as well as new genera such as Sifrhippus, Minippus, and Arenahippus. The old days when all early Eocene horses could be lumped into one genus (whether Eohippus or Hyracotherium) are long gone!
From these early Eocene horses, there is a general size increase through Eocene forms like Orohippus and Epihippus, culminating in the German shepherd–sized horse Mesohippus and Miohippus from the late Eocene and Oligocene (Prothero and Shubin 1989). These horses had three robust toes on their hands and feet, slightly higher-crowned teeth, and many other more horselike features of the snout and skull. By the early Miocene, horses began an explosive radiation (fig. 14.3) into multiple lineages. Some of these Miocene horses retained low-crowned teeth for leaf eating (the anchitherines), but most evolved higher-crowned teeth for eating gritty grasses and also developed longer legs and toes (with reduced side toes) to run fast across the grasslands. Finally, most of these diverse horse lineages went extinct in the late Miocene, leaving only the lineage leading to the modern genus Equus to flourish in the Pliocene and Pleistocene.
The biggest difference between the old pattern of horse evolution (fig. 14.2) and our modern one (fig. 14.3) is its bushiness. Back in the late 1800s and early 1900s, there were only a few horse fossils known at each level, and it was easy to imagine one continuous lineage evolving through time in a “ladder-like” fashion. But tens of thousands of additional horse fossils have been collected since the 1920s. Just as we have seen in every other instance, horse evolution is a bushy, branching pattern, with multiple lineages coexisting at the same time (fig. 14.3). Gingerich (1980, 1989) and Froehlich (2002) document multiple species of horses coexisting in the early Eocene. Prothero and Shubin (1989) found that three different species of Mesohippus and two of Miohippus coexisted in some upper Eocene beds in Wyoming. Some Miocene quarries in Nebraska, such as Railroad Quarry A in the Valentine Formation of north-central Nebraska, yield as many as 12 different contemporary species of horses. Even today, the genus Equus is very speciose, with not only domesticated horses and their ancestral stock, Przewalski’s horse, but three species of zebras, and several more species of wild asses and onagers. Thus the general trend of the classic diagram shown in figure 14.2 is factually correct, but a gross oversimplification that does not capture the bushy pattern that we now recognize.
Naturally, this example has been presented so often that creationists feel obligated to attack and distort it and defuse its powerful impact. Their lies about the horse evolution story never actually involve looking at real specimens or doing their own research. They are just quotations out of context that distort what the author is really saying or quotations from really old sources that are no longer describing the current state of our knowledge. For example, Gish (1995:189–197) devoted nine pages to the topic, all of which are highly misleading and dishonest. His most common tactic is quotes out of context that have authors pointing out that the old simplistic linear horse evolution story (fig. 14.2) is no longer valid—without giving the rest of the quote in which the author points out that the fossil record is now much better, and the horse phylogeny is very bushy! In other cases, he quotes authors as saying that we have few gradual transitions between species—but as we showed in chapter 4, under the model of punctuated equilibrium, we don’t expect gradualism in horses. Nevertheless, by looking at the features of each successive species, we can see the evolutionary trends in the group just fine. Remember, just because they don’t show gradual continuous change doesn’t mean that they show no evolution! In fact, some paleontologists argue that they can show gradual change between horse species, one example being Phil Gingerich (1980, 1989), with his excellent detailed record of early Eocene horses from the Bighorn Basin of Wyoming. Similarly, when Neil Shubin and I (Prothero and Shubin 1989) looked closely at the horses from the Eocene and Oligocene deposits in the Big Badlands and related areas, we found that what had been interpreted as a gradual transformation from Mesohippus to Miohippus made more sense as a bushy pattern of evolution. Nonetheless, the transformation from one species to another is very subtle. MacFadden (1984, 1992) has documented the subtle distinctions between different species of Miocene horses, which form a nice series of species transformations, yet Gish (1995) misquotes him to indicate that horses didn’t evolve at all! Again and again, Gish falsely claims there are no transitional forms between the species, but if he had bothered to read the original literature closely, or better yet, look at the real specimens, he would have been overwhelmed by the continuous gradation in fossil species from the Eocene to Equus. Finally, Gish falsely claims (based on out-of-context quotations from outdated books written 50 years ago) that there are no transitional forms linking horses to anything else. But those transitional forms have been known for a long time. They include the archaic ungulates known as phenacodontids (documented by Radinsky 1966, 1969), and the Paleocene Mongolian creature Radinskya (fig. 14.4), which is such a good link between early perissodactyls and their relatives among arsinoitheres and elephants that McKenna et al. (1989) had a hard time deciding what group to put it in.

FIGURE 14.4. The Paleocene Chinese fossil Radinskya, which is a primitive relative of the perissodactyls, shows that they originated in Asia in the Paleocene. The differences between this skull and the earliest horses and rhinos are very subtle. (Photo courtesy M. C. McKenna)
Intelligent design creationist books also mention horse evolution but do not discuss it in any detail. Davis and Kenyon (2004:96) deny the existence of the evolutionary sequence of horses, yet make no further mention of it anywhere else in their book. Wells (2000:195–207) discusses how the ideas of horse evolution have moved away from the old linear “straight-line” notions to the modern complex phylogeny, but nowhere does he dispute the reality of horse evolution. Instead, his convoluted argument seems to suggest that if we change our notions from a simplistic linear model to a more complex bushy model, we are denying that horse evolution occurred! Of course our notions have changed—we have more fossils and more data. We would be bad scientists if our ideas didn’t change in the face of new data. Sarfati (2002) makes the absurd claim that “the other animals in the sequence show hardly any more variation between them than within horses today” (133). Clearly, he has never looked at the actual specimens, because no one would mistake the tiny collie-sized Eocene horses, nor Mesohippus, which was the size of a Great Dane, for any living horse (not even the tiniest pony). Not only are they dramatically smaller than all living horses, but they have a whole range of primitive anatomical features (three functional digits on their hands and feet, shorter snout, much lower crowned teeth, and many other features) that clearly distinguish them from any living horse, no matter how small.
In fact, if creationists had bothered to spend any time at all looking at real fossils, they would have been amazed by how subtle the transition is from phenacodontids and Radinskya to the early perissodactyls. Even more surprising, the earliest Eocene horses, rhinos, and tapirs are also very hard to tell apart—yet modern horses look nothing like the living tapirs or rhinos today. This fact struck me when I was working on my undergraduate research project on early Eocene mammals from the Bighorn Basin of Wyoming. Although the literature on the subject was clear, it was a major challenge trying to tell the earliest horse teeth from the teeth of Homogalax, the earliest member of the rhino-tapir lineage. They are virtually identical in size and in cusp-by-cusp detail (fig. 14.5), except that Homogalax tends to have slightly better connections of the crests between the cusps. Anyone without a sharp eye would miss this difference completely, and the same is true of the skulls and skeletons. All of the early perissodactyls (horses, rhinos, tapirs, and brontotheres) look so similar when they begin their evolution that only a trained eye can tell them apart. Yet we can trace the evolution of each of these distinct lineages through time, and they soon begin to look very different, so that by the late Eocene, they are dramatically distinct in size and body shape, and even a kid could tell them apart. This is one of the best examples of how we can document the origin of many modern distinct lineages back to ancestors that converge to the point of being virtually indistinguishable.

FIGURE 14.5. The evolutionary radiation of perissodactyls, showing the major branches of the horses, rhinos, tapirs, chalicotheres, bronthotheres (Megacerops), and other extinct groups. As can be seen from the crown views of the upper left cheek teeth, the details of the crests and cusps are extremely similar between Radinskya, the early brontothere Palaeosyops, the primitive horse Protorohippus (long called “Hyracotherium”), the primitive moropomorph Homogalax, the chalicothere Litolophus, the tapiroid Heptodon, and the primitive rhinoceros Hyracodon. Shown next to the upper cheek teeth are typical skulls of horses, tapirs, and rhinos, emphasizing how similar they all looked in the early stages of perissodactyl evolution. The numbered branching points are as follows: 1, Perissodactyla; 2, Titanotheriomorpha; 3, Hippomorpha; 4, Moropomorpha; 5, Isectolophidae; 6, Chalicotherioidea; 7, Tapiroidea; 8, Rhinocerotoidea. (Phylogeny after Prothero and Schoch 1989; redrawn by Carl Buell)
But if the evolution of horses is not convincing enough, let’s look at my favorite group, the rhinoceroses. They have just as long and dense and detailed a fossil record as horses, yet they have received almost no attention because their systematics was a mess for decades and nothing could be concluded until the valid species were determined using the new collections (Prothero 2005). Once that was done, however, we again see a highly bushy, branching family tree of rhinos (fig. 14.6) in North America (and a similar pattern in Eurasia), with many different families, species, and genera spanning almost 50 million years. The earliest relative of rhinos was the early Eocene form known as Homogalax, which also gave rise to tapirs, and yet Homogalax is virtually identical to the early Eocene horses (fig. 14.5). By the middle Eocene, we see the split between the tapiroid lineage and the lineages that lead to the three main families of rhinocerotoids. Unlike horses, which evolved mainly in North America with occasional emigrations to Eurasia, rhinos evolved on both hemispheres and immigrated back and forth, so their family tree is much more bushy and dominated by sudden immigration events than that of the horse (fig. 14.6). Although most of the species are distinct, we can still see evolutionary trends, particularly in the front of the snout (fig. 14.7A), where the primitive forms have many incisors and small canines, and as rhinos evolve, they lose most of the incisors and develop sharp, short tusks between their remaining incisors. In addition, their cheek teeth (fig. 14.7B) also show evolutionary trends, such as the modification of the primitive premolars into molar-like crests that resemble the Greek letter “π.” My rhino monograph (Prothero 2005) documented many other changes, both gradual and punctuated, such as the size changes in many lineages, and the gradual development in horns within the genus Diceratherium. Like horses, rhinos also get larger and more specialized throughout their evolution. They started out with four toes on the front foot, and reduced it down to three by the middle Eocene—but unlike horses, they remained three-toed even today and never became highly specialized one-toed runners like living horses.

FIGURE 14.6. The evolutionary history of North American rhinoceroses. In the Eocene, they branched into three families, the hippo-like amynodonts, the long-legged running hyracodonts, and the living family Rhinocerotidae. During their evolution, they varied not only in body size and limb and skeletal proportions but also in the number and position of horns (or lack of horns), the details of their teeth, and many other features. (Drawing by C. R. Prothero; after Prothero 2005)

FIGURE 14.7. The different rhinocerotoid families can be distinguished largely through the modifications of their teeth. (A) Primitive forms such as Hyrachyus (a) have the tapir-like condition, while hyracodonts like Ardynia (b) and Hyracodon (c) have reduced their canines and modified their incisors. The gigantic indricothere Paraceratherium (d) has lost all its front teeth except for huge conical incisor tusks. The amynodont Metamynodon (e) has reduced its incisors and developed heavy chisel-like upper and lower canines. The true rhinoceros Trigonias (f), on the other hand, has almost all of its incisors and canines, with a chisel-like upper incisor and a tusk-like lower incisor. (Drawing by Carl Buell). (B) Evolutionary transformation of the cheek teeth of Hyracodon, showing the gradual transformation of the premolars into more molarized crests. (After Prothero 2005)
It comes as a shock to many people that most fossil rhinos were hornless. Modern rhino horns are made of tightly compacted hairs and have no bony support (unlike the horns of cattle or antelopes), so they seldom fossilize. We can usually determine the presence of a horn by a patch of spongy roughened bone on the snout or forehead that served as the attachment point. On this basis, rhinos were hornless during most of their evolution, and then in the Oligocene (about 28 million years ago) they independently evolved two different types of paired horns on the tip of the nose (Diceratherium and Menoceras). After these lineages disappeared, there was a long period dominated by hornless rhinos, except for a single nose horn in the Teleoceras lineage. Asian rhinos also had a single nose horn, while the two African rhino genera, the black rhino and white rhino, have two horns in tandem. The elephant-sized Eurasian ice age elasmotherine rhinos had a huge horn (1.5 meters or 5 feet long) on their foreheads, but none on their noses!
Closely related to the rhinos are the tapirs, which look vaguely piglike, except that they have an odd number of toes and a flexible snout or proboscis. Most people have seen them only in zoos (if at all) because all the living species are restricted to the tropics, including Southeast Asia (the Malayan tapir) and three species in Central and South America. But tapirs were widespread in North America and Eurasia through most of the past 50 million years, although their teeth are adapted for chopping leaves, so they have always been restricted to the more forested regions. Tapirs, too, have an impressive fossil record of their evolution (fig. 14.8). The early more primitive forms like Homogalax mentioned earlier (fig. 14.5) can barely be distinguished from the earliest horses and rhinos. The only clue is that the cross crests on their molars are slightly more developed (foreshadowing the strong crests on later tapir teeth), but otherwise their skulls and skeletons look almost identical to the earliest rhinos and horses. However, through the rest of the Eocene they become more and more specialized, and more and more like living tapirs. Even by the middle Eocene, their teeth have more strongly developed cross crests. My friend Matt Colbert described a new species of tapir, Hesperaletes, from the Eocene deposits of San Diego (not far from the old headquarters of the ICR) that is the oldest fossil to show evidence of a nasal notch on the skull for attachment of the proboscis. Through the past 40 million years, tapir skulls show a deeper and deeper retraction of the nasal notch, suggesting a larger and better-developed proboscis, until we reach the condition in living tapirs, which have the largest proboscis of all. During this time their molars become more and more highly specialized, until they are simple cross crests ideally suited for chopping leaves.

FIGURE 14.8. Evolution of the tapirs from primitive forms with skulls much like Eocene horses and rhinoceroses, through progressively more specialized forms that have a deeper retraction of the nasal notch, indicating a larger proboscis. (Modified from Prothero and Schoch 2002)
Finally, we should mention one more example from the perissodactyls: the brontotheres, also known as the titanotheres (figs. 14.9 and 14.10). At the climax of their evolution in the latest Eocene, these elephant-sized beasts sported a pair of blunt bony battering rams on their noses, yet they evolved from beagle-sized unspecialized early Eocene ancestors (Palaeosyops) that are difficult to distinguish from the earliest Eocene horses and tapir-rhino ancestors. A century ago, the famous paleontologist Henry Fairfield Osborn spent years studying them and published a huge two-volume work (Osborn 1929) that was chock-full of bad taxonomy (even by the standards of his day). It was further confused by his unorthodox ideas about orthogenesis, or straight-line evolution going out of control in one direction without the restraint of natural selection. Unfortunately, this outdated example (fig. 14.9) continues to be published in textbooks today, even though it falsely portrays their evolution as a single straight lineage. In fact, brontothere evolution was very bushy as well (fig. 14.10), with multiple lineages coexisting in the middle and late Eocene, after which the entire group died out. Lest the creationists attempt to misquote me, brontotheres do show evolutionary changes in size and horn development through time, but it is in the context of many different species branching out, not a single lineage marching to their ultimate extinction as Osborn once thought.
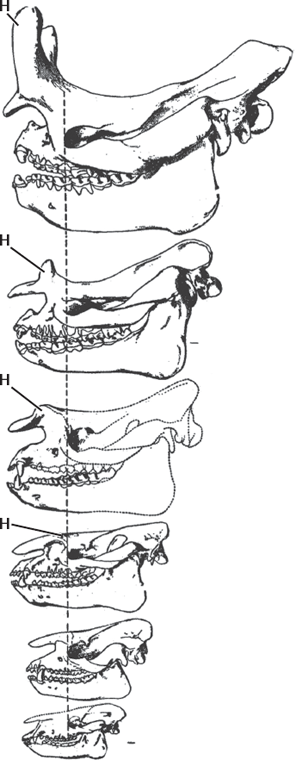
FIGURE 14.9. Conventional linear view of brontothere evolution through the Eocene from primitive forms like Palaeosyops that are barely distinguishable from contemporary horses (fig. 14.5) through larger and larger forms that eventually developed two blunt horns (H) on their noses. (After Osborn 1929)
FIGURE 14.10. A modern view of brontothere evolution, showing the more branched bushy pattern of species through time, based on the work of Mader (1989). (From Prothero 1994b)
Nevertheless, these shall ye not eat, of them that chew the cud or of them that divide the cloven hoof; as the camel and the hare, and the coney; for they chew the cud, but divide not the hoof, therefore they are unclean unto you. And the swine, because it divideth the hoof, yet cheweth not the cud, it is unclean unto you; ye shall not eat of their flesh, nor touch their dead carcass.
—Deuteronomy 14:7–8
After the Perissodactyla, the second great living order of hoofed mammals is the even-toed hoofed mammals, or Artiodactyla. They are “even toed” or “cloven hoofed” because the axis of symmetry of the foot runs between the third and fourth toes, and so they usually have either two toes or four. Today, they are the most diverse and abundant ungulates on the planet, with over 190 living species, including pigs, peccaries, hippos, camels and llamas, deer, pronghorns, giraffes, sheep, goats, cattle, and dozens of species of antelope (fig. 14.11). Nearly every domesticated animal we eat is an artiodactyl (pigs, sheep, goats, cattle, and deer), and they provide us with all of our milk (whether from a cow, goat, or camel) and wool (from either sheep or alpacas). Almost every large herbivore you might see in East Africa is an artiodactyl except for zebras, rhinos, and elephants. Artiodactyls are indeed a modern success story, yet they acquired that dominance gradually as the odd-toed perissodactyls (especially horses, rhinos, tapirs, and brontotheres) dominated in the Eocene and gradually were displaced, while artiodactyls (especially the ruminants, such as camels, sheep, goats, and cattle) with their superior mode of digestion came to dominate the earth.

FIGURE 14.11. Evolutionary history of the even-toed hoofed mammals, or artiodactyls. (Drawing by C. R. Prothero; from Prothero 1994b)
Yet once again, a look at the first known artiodactyls from the early Eocene reveals a form that no one would connect with a cow, giraffe, or camel (fig. 14.12). Diacodexis and Dichobune were tiny creatures, about the size of a rabbit, with long delicate limbs and simple primitive teeth and skulls. Some of them had very long hind limbs and were apparently well adapted to hopping and leaping as well. Both anatomical cladograms of the ungulates (Prothero et al. 1988) and the molecular phylogenies (Murphy et al. 2001a, 2001b) place their branching point at the very base of the ungulate radiation (fig. 14.1). Ken Rose (1987) has suggested that the Paleocene archaic ungulate Chriacus looks very much like a possible transitional form between the earliest ungulates and artiodactyls. Once artiodactyls appeared in the early Eocene, they became very stereotyped and distinctive in many features. Their simple cusped teeth soon evolved into half-moon-shaped crests, giving them their signature selenodont teeth. Their legs and feet were long and delicate from the very beginning and soon began to lengthen the central toes and reduce the side toes (but in a different way than horses). All artiodactyls have a distinctive double-pulley bone in their ankle known as the astragalus, which made their legs very efficient for front-to-back running motions (but prevented them from rotating their foot or hind leg out of the front-to-back plane).
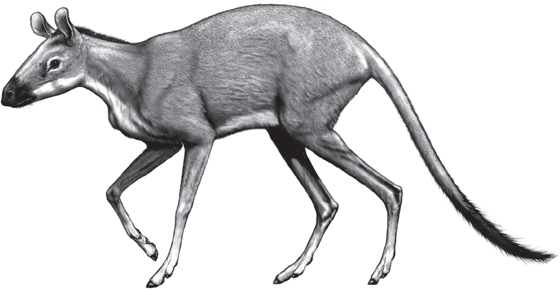
FIGURE 14.12. The earliest even-toed hoofed mammals, or artiodactyls, such as Diacodexis and Dichobune, were small primitive forms that looked nothing like their living descendants, the pigs, hippos, camels, deer, giraffes, pronghorns, cattle, sheep, antelopes, and goats. Nevertheless, they had the characteristic teeth found in all primitive artiodactyls and distinctive features of the skull and ankle region that mark them as ancestors of this great order of mammals. (Drawing by Carl Buell)
By the middle Eocene, artiodactyls were undergoing an explosive radiation with many different families, most of which are now extinct (fig. 14.11). However, the primitive relatives of pigs were present, as were the first camels and the first ruminants. In the Oligocene, another explosive evolutionary radiation occurred, this time with mostly living families, such as the camels and early ruminants, and by the Miocene, the pronghorns, giraffes, antelopes, and cattle were beginning to diversify as well. Some of these families have extraordinary fossil records, and we can trace their lineages back to the Eocene, then look forward to how they evolved into a wide diversity of forms in the Oligocene and Miocene. For space reasons, we’ll look at just two examples, the camels and the giraffes.
Most people are surprised to learn that extinct camels did not have humps and that the camel family evolved in isolation in North America (fig. 14.13A). They only escaped this continent in the late Cenozoic when they reached South America 3 million years ago to evolve into llamas, guanacos, and vicuñas, and Eurasia about 7 million years ago, where they evolved into the African dromedary and the Asian Bactrian camels. After all this success, they vanished from their ancestral North American homeland at the end of the last ice age 10,000 years ago. Fossil camels are also surprising in their amazing array of ecological types, far exceeding the limited forms we see today (Honey et al. 1998). The earliest camels were tiny rabbit-sized creatures (Poebrodon) that are known from isolated teeth and jaws from the late middle Eocene of Utah, Texas, and California. But by the late Eocene and early Oligocene they had evolved into sheep-sized creatures known as Poebrotherium (Prothero 1996), which are common in the Big Badlands of South Dakota. Poebrotherium has all the hallmarks of a typical early camel: very high-crowned selenodont teeth, long limbs that were nearly fused into a cannon bone, and the signature features of the skull and skeleton as well (fig. 14.13B). Yet its proportions looked more like those of an antelope or a gazelle, and it apparently had no hump either. In the late Oligocene and early Miocene, camels underwent an explosive evolutionary radiation (fig. 14.13A) into relatively short-limbed varieties (protolabines and miolabines); tiny delicate gazelle-like forms with extraordinarily high-crowned teeth (stenomylines); long-legged, long-necked forms that looked much like the modern guanaco or vicuña (aepycamelines); and even a group that evolved long necks and performed the roles of treetop browsers that giraffes occupied in the Old World. Some of these late Miocene and Pliocene “giraffe-camels” were huge as well, with appropriate names like Gigantocamelus and Titanotylopus. Then, after spreading to Eurasia and South America in the late Miocene and Pliocene, camels dropped in diversity during the ice ages, and only a few species were left when they vanished from North America 10,000 years ago.
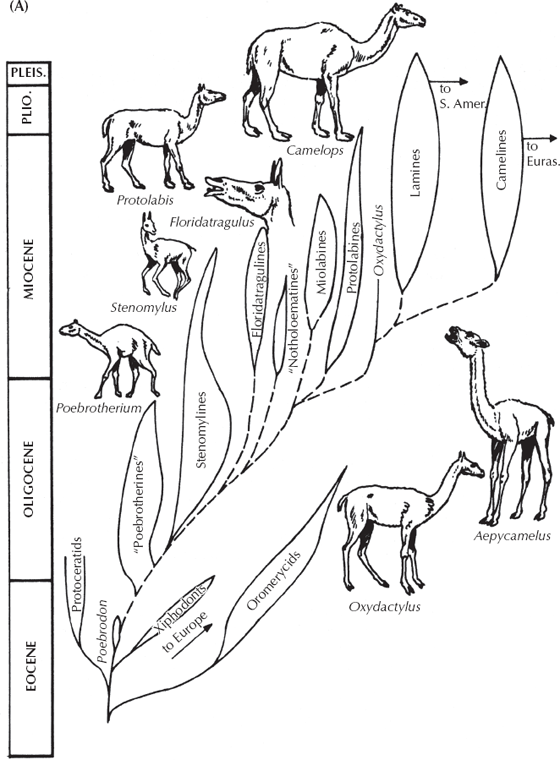

FIGURE 14.13. The evolution of camels in North America. (A) The family tree of camels, showing the great diversity of forms, from small primitive deerlike creatures to the gazelle-like stenomylines, the short-legged protolabines and miolabines, the long-legged long-necked “giraffe camels,” and the modern humpless South American camels (alpaca, llama, vicuña, guanaco), which are more typical of the whole family. Only the living African dromedary and two-humped Asian Bactrian camels have humps. (Drawing by C. R. Prothero; after Prothero 1994b) (B) Evolutionary trends within the camels, from the tiny oromerycid Protylopus through the Oligocene camel Poebrotherium through more advanced Procamelus. Although their history is not a straight line of evolution but a bushy branched pattern, there are trends toward larger body size, loss of the front teeth, longer snouts and larger eyes, longer legs and toes (reducing to just two toes fused together), and higher-crowned cheek teeth. (After Scott 1913)
For our final example from the artiodactyls, let us consider the giraffe. As we have been pointing out all along, the modern representatives of many mammalian families are very atypical of most of the members of the family during its evolution. Most fossil rhinos did not have horns, most fossil camels did not have humps, and most fossil giraffes did not have long necks. Extinct giraffids (Solounias 2007) sported a wide diversity of horn shapes and body sizes (fig. 14.14). Most fossil giraffes looked more like the short-necked okapi (fig. 14.14B), a shy white-and-brown-striped denizen of the African rain forests, and the only other living giraffid. Others, like Sivatherium, got to be huge and heavy with moose-like palm-shaped horns. In the late Miocene, we finally see the lineage that leads to the modern Giraffa. Although the fossil teeth are common enough, complete skeletons with the neck vertebrae are rare, so we can see how the lineage evolved, but didn’t yet have fossils to show how the neck got longer. The oldest known fossils of the genus Giraffa jumae from the late Miocene of Africa already seems to have a long neck, so we need to seek fossils from earlier in the Miocene. Nikos Solounias (1999) has shown the mechanisms by which the neck lengthens, and the prevailing ideas have to be revised. Giraffes lengthen each vertebra somewhat but actually add an extra vertebra to the neck, then shift the last neck vertebra to the shoulder region. This is why the giraffe neck begins behind the forelimbs and why giraffes have their distinctive posture with the legs out in front and the neck balanced almost over the center of the body, rather than sticking forward all the time as in most mammals. Finally, Solounias has just described a description of a classic transitional form (fig. 14.15): a giraffe fossil with an intermediate-length neck, longer than that of the okapi and the other extinct forms but shorter than that of the living giraffes. For so many years, people have speculated about how giraffes got their long necks, and now we finally have the fossils to show exactly how it happened! Once again, the fossil record has yielded a transitional form that the creationists claimed could never exist.

FIGURE 14.14. Evolution of the giraffe family. (A) The modern okapi is more typical of the group, with its short neck and relatively short horns or “ossicones.” Some fossil giraffids, however, had very unusual branching and flaring cranial appendages. (Drawing by C. R. Prothero; after Prothero 1994b) Only the lineage of the modern giraffid evolved a long neck. (B) The modern okapi, much more typical of the giraffe family than its long-necked cousin. (Photo by the author)
FIGURE 14.15. Neck vertebrae of a recently discovered fossil giraffid, Samotherium major, that are intermediate in length between those of primitive giraffids and the modern long-necked species. This amazing discovery is a true “missing link” between okapis (bottom) and the long-necked modern species (top). (Drawing courtesy N. Solounias)
These dogmatists, who by verbal trickery can make white black, and black white, will never be convinced of anything, but Ambulocetus is the very animal that they proclaimed impossible in theory…. I cannot imagine a better tale for popular presentation of science or a more satisfying, and intellectually based political victory over lingering creationist opposition.
—Stephen Jay Gould, “Hooking Leviathan by Its Past”
People are startled to learn that most fossil rhinos didn’t have horns, most fossil camels didn’t have humps, and most fossil giraffes had short necks. But they are even more surprised to learn that whales are related to hoofed mammals and are descended from a group of carnivorous hoofed mammals. In debates, creationists love to exploit this public ignorance of the fossil record and zoology by putting up a slide of “Bossie” the cow and “Blowhole” the whale and a ridiculous cartoon of an intermediate between a cow and a whale. But when we said that whales are descendants of ungulates, we did not say “cows.” Apparently, when creationists hear the words “hoofed mammal,” cows are the only kind they can think of. Actually, hippos would be better models for a modern relative of whales, and they are not nearly so different from whales (both are large and aquatic).
Ever since people realized that whales and dolphins were mammals, they have speculated about how they might have evolved from land-dwelling mammals and from which group of mammals they originated. By the 1830s and 1840s, specimens of huge primitive whales known as archaeocetes (fig. 14.16) were being discovered in middle Eocene beds of Alabama, but these specimens were fully aquatic, with flippers and tail flukes and a sinuous 24-meter-long (80 foot) body. Clearly, the origin of whales must have occurred before the middle Eocene, but nothing was known of their fossil record prior to that time. In 1966, Leigh van Valen and others had shown that the skulls and teeth of primitive whales looked very much like the predatory archaic hoofed mammals known as mesonychids. Even though mesonychids were land mammals with hooves, there were many similarities in the skull and skeleton (especially the large, serrated triangular bladelike teeth) that suggested close relationship with archaeocete whales. Yet for over a century, there were no transitional fossils known between mesonychids and archaeocetes.

FIGURE 14.16. Evolution of whales from land creatures, showing the many transitional fossils now documented from the Eocene beds of Africa and Pakistan. (Drawing by Carl Buell)
Until very recently, paleontologists were comfortable with the idea that whales were related to mesonychids, and the fossil evidence seemed to bear this out. Then, in the late 1990s, molecular studies showed that among living mammals, the artiodactyls (and particularly the hippos) were the nearest relatives of the whales. This wasn’t too surprising because artiodactyls and whales are very closely related on the ungulate cladogram (fig. 14.1), although we always thought they were sister taxa, not that whales were nested within artiodactyls (Prothero et al. 1988). But in 2001, two independent groups of scientists (Gingerich et al. 2001; Thewissen et al. 2001) found specimens of early whales that preserved the ankle region (fig. 14.17). Amazing as it seems, these fossils clearly showed that early whales had feet bearing ankles with the characteristic double-pulley astragalus, the signature feature of the whole order Artiodactyla. Since then, we’ve rethought the evidence, and now most scientists would agree that whales are a group that evolved from the hippo-pig lineage within artiodactyls and that mesonychids are the distant relatives of both whales and artiodactyls (Geisler and Uhen 2005).
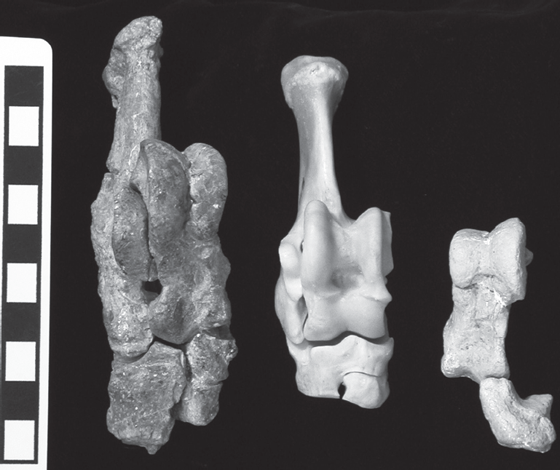
FIGURE 14.17. Ankle bones of middle Eocene whale Rodhocetus balochistanensis (left) and Artiocetus clavis (right) from Pakistan, compared with those of the pronghorn Antilocapra americana (center). Note double-pulley astragalus characteristic of mammals of the order Artiodactyla. (Photo courtesy P. D. Gingerich, University of Michigan Museum of Paleontology)
The breakthrough in our understanding of whale ancestry occurred when scientists began to collect fossils in the lower Eocene beds of Pakistan. In 1983, Phil Gingerich and colleagues described Pakicetus, based on a skull with an archaeocete braincase but lacking ears that were capable of echolocation and with teeth intermediate between those of mesonychids and archaeocetes (fig. 14.16). Pakicetus came from river sediments bordering shallow seaways, suggesting that it might have been a semiaquatic predator that waded in rivers part-time to find food. The skeleton of Pakicetus is still quite wolflike, with long slender limbs and a tail, so it still resembles a mesonychid in most features. The chemistry of its bones showed it lived in freshwater.
The next development occurred a few years later, when Gingerich et al. (1990) described new specimens of the archaeocete Basilosaurus from the middle and upper Eocene deposits of Egypt (in a locality just west of the Pyramids). Although it was like other archaeocetes in being fully aquatic, these new specimens had something never previously preserved: the hind limbs. In most whales, there are no external hind limbs, but the remnants of the hip and thigh bones are buried in muscles along the spine halfway down the body (fig. 4.9). These specimens, however, had tiny hind limbs (about as large as a human arm on a 24-meter-long body!), which clearly did not function for locomotion. Like the vestigial hind limbs of modern whales buried inside the body, these tiny limbs were functionless relicts of the day when “whales” did walk on land. Since this discovery, other archaeocetes such as Takracetus and Gaviocetus have been found to retain vestigial hind limbs.
The most important discovery occurred when Thewissen et al. (1994) discovered and described Ambulocetus natans, whose name means “walking swimming whale.” Found in middle Eocene marine beds of Pakistan, it was about the size of a sea lion (fig. 14.18), with functional flippers on both its forelimb and huge hind limb (which still had vestigial hooves as well). Its skull and teeth, however, were still like those of mesonychids. On the basis of its highly flexible vertebrae, Thewissen et al. (1994) suggested that Ambulocetus swam with an up-and-down flexure of its body, similar to the swimming motion of an otter rather than paddling with its feet like a penguin or seal or wriggling side to side like a fish. This is a precursor to the up-and-down motion of a whale’s tail flukes as it swims through the water.

FIGURE 14.18. Ambulocetus natans, the primitive whale from the Eocene of Pakistan that still retains a mesonychid-like head, large functional webbed hands and feet, and a semiaquatic mode of life. (A) Photograph of the nearly complete skeleton laid out in anatomical position. (Photo courtesy J. G. M. Thewissen). (B) Reconstruction of Ambulocetus lunging out of the water to capture another Eocene mammal. (Reconstruction by Carl Buell)
Further discoveries (mostly in the middle Eocene of Pakistan) followed one after another. Dalanistes, for example, had fully functional front and hind limbs with webbed feet and a long tail but was much more whalelike with a longer snout. Rodhocetus was even more like a dolphin, yet still retained functional hind limbs. Indohyus, on the other hand, provides a link between Pakicetus and the primitive land relatives of whales and hippos known as anthracotheres. As the years go by, more and more transitional whales are being discovered, so that by now the amazing transformation from land mammals to whale is one of the best examples of evolutionary transitions in the fossil record (fig. 14.16). This may not make creationists happy, but the fossils cannot be denied.
Creationists have been flummoxed by all this new evidence. The ID creationist textbook Of Pandas and People: The Central Question of Biological Origins (Davis and Kenyon 2004:101–102) claims “there are no transitional fossils linking land mammals to whales.” They could not be more wrong. This false statement is carried over from their 1989 edition into their 2004 edition, yet the 1980s and 1990s yielded an amazing array of transitional whale fossils that clearly link terrestrial land mammals to full-fledged aquatic whales. These fossils have been well documented in many television shows and websites, in popular books such as Carl Zimmer’s (1998) At the Water’s Edge: Macroevolution and the Transformation of Life, and in high-profile scientific journals such as Science and Nature, so there is no excuse for creationist ignorance or denial of these fossils. Davis and Kenyon (2004:101, figs. 4–5) illustrate two extremes of the whale evolutionary sequence (the terrestrial mesonychids and the aquatic archaeocetes) but falsely state that there are no transitional forms between them. Sarfati (2002:135–141) snipes at the fact that a few of these spectacular whale fossils are not 100 percent complete and suggests that we cannot draw any conclusions from fossils that are 80 percent complete! He also falls back on the false idea that if whales did not form a clear series of ancestors and descendants, then they do not show an evolutionary transition. But as figure 14.16 shows, one could not ask for a more clear-cut series of intermediate forms from fully terrestrial to fully whalelike fossils.
Every time Duane Gish got into another debate, his opponent brought up Ambulocetus and the amazing sequence of transitional whale fossils and showed any open-minded individual how whales evolved. Gish (1995:199–208) blustered on for nine pages about these new discoveries, but his discussion is very confused and self-contradictory. He never addressed the main evidence but sniped at minor quibbles between specialists. After he has mentioned numerous red herrings and blown smoke screens on irrelevant points, he makes the following revealing comment (Gish 1995:203): “Confused? So are we.” Nowhere does he address the obvious intermediate anatomy of Ambulocetus, Dalanistes, Rodhocetus, and all the rest of these new fossils. His verbal gyrations basically boil down to this: if it is a modern whale without hind limbs it is really a whale, but if it is a transitional form with a whale’s head but intermediate forelimbs and hind limbs (for either walking or paddling), it cannot be a whale, but some unknown fossil! Essentially, he dodges the problem by defining whales in his mind so they cannot have the possibility of intermediate forms. That is not intellectually or scientifically honest and shows the complete bankruptcy of his illogical thinking. And the final clinching evidence is the fact that living whales do have hind legs—they are merely vestiges of the hip bone and thigh bone, usually buried deep in their muscles and not visible on the surface (fig. 4.9). Nevertheless, this is final proof (if all the molecules and fossils were not already enough) that whales indeed are descended from four-legged land mammals.
There is no creature among all the Beasts of the world which hath so great and ample demonstration of the power and wisdom of almighty God as the Elephant.
—Edward Topsell, The Historie of Foure-Footed Beastes
Now that we have seen that most of the popular large hoofed mammals—horses, rhinos, camels, giraffes, and whales—have excellent fossil records that document transitional forms going all the way back to the Cretaceous, we need to look at one more group: the elephants and their relatives. Elephants, too, have an excellent fossil record in the late Oligocene and more recent rocks, because mastodonts left Africa about 18 million years ago and migrated among all the northern continents (fig. 14.18). Unfortunately, we are somewhat handicapped because most of their early evolution took place in Africa, and we have a relatively poor fossil record in Africa before the early Oligocene. Nevertheless, we can trace their lineage back from the modern Asian and African elephants and their extinct relatives, the mammoths and mastodonts, through more primitive lineages with a wide variety of tusks and different lengths of trunks. Some (the anancines) had two huge long straight tusks protruding from their skulls, while others (the stegotetrabelodonts) had four long straight tusks; others (the deinotheres) had two tusks that curled down from the lower jaw, and still others (the amebelodonts) had their lower tusks flattened into large shovel-like blades. Going back farther into the early Oligocene, the famous Fayûm beds of Egypt (source of the archaeocete whales with tiny hind limbs) also produce very primitive, small mastodonts with short jaws and even shorter tusks, known as Palaeomastodon and Phiomia. In the early Oligocene, the various lineages of proboscideans (elephants, mammoths, and mastodonts) are very primitive and hard to tell apart, typical of the early stages of an evolutionary radiation (figs. 14.19 and 14.20). These primitive forms can be traced back to the ultimate transitional fossil, Moeritherium, from the late Eocene of Egypt. Superficially, it looked more like a tapir or a pygmy hippo than an elephant and probably only had a short proboscis, not a long trunk. But a close look at the skull shows that it had very short tusks in the upper and lower jaws, the teeth of a primitive mastodont (not those of a tapir or hippo), and the details of the ear region and other part of the skull (such as the condition of the jugal bones in the zygomatic arch) are unique to the Proboscidea as well.
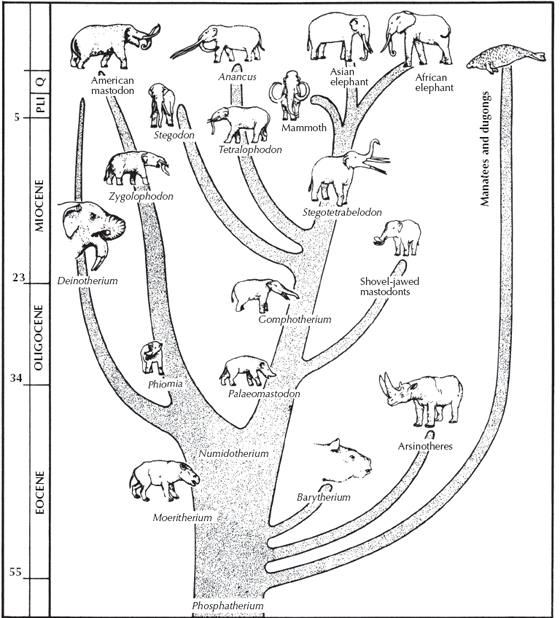
FIGURE 14.19. Evolutionary history of the elephants and their kin (Proboscidea), starting with pygmy hippo-like forms like Moeritherium with no trunk or tusks, through mastodonts with short trunks and tusks, and concluding with the huge mammoths and the two living species. Early in their history, the other tethytheres branched off from the Proboscidea. These include the manatees, order Sirenia, the extinct desmostylians, and the extinct horned arsinotheres. (Drawing by C. R. Prothero; from Prothero 1994b)
FIGURE 14.20. Details of the evolution of the skull, tusks, and trunk of proboscideans, from the pygmy hippo-like Moeritherium through mastodonts with longer tusks and trunks to mammoths. (Drawing by M. P. Williams).
All of these fossils have been known for decades, but in the last few years, paleontologists have found even older and better transitional forms. There is the 1984 discovery of an even more primitive proboscidean, Numidotherium, from the early Eocene of Algeria (Mahboubi et al. 1984). Although the specimen is very incomplete, it already had the high forehead, the retracted nasal opening (indicating a short proboscis), short upper tusks, mastodont-like teeth, and the lower front jaw is beginning to develop a broad scoop, a diagnostic feature of mastodonts. It was only a meter tall (3 feet) at the shoulder, smaller even than Moeritherium, yet it already had the limb characteristics found in later, larger mastodonts. In 1996, Gheerbrant and others reported the discovery of an even earlier proboscidean, Phosphatherium, from the late Paleocene of Morocco. The fossil consists only of a partial skull (typical of the poor preservation of mammal fossils in the Paleocene worldwide), but the teeth already show the distinctive mastodont pattern at the very beginning of proboscidean evolution. Thus we now have fossils to trace modern elephants continuously back through many different transitional forms to forms that are almost 60 million years old, and that brings us almost to the time when all the hoofed mammal lineages diverged. Other fossils from the Paleocene of North Africa, such as Daouitherium and Eritherium, are even more primitive, although known only from teeth and jaws.
But proboscideans are not the only members of this clade. In his groundbreaking 1975 paper on the cladistic classification of mammals, Malcolm McKenna suggested that the closest living relatives of the elephants was the sirenians or “sea cows,” better known to us as the manatees and dugongs. Although sirenians are aquatic forms with flippers and a broad tail fluke and no hind limbs that look superficially nothing like elephants, all the evidence shows their close relationship. There are many details of the skull and jaw, and even of the teeth, that closely resemble the early mastodonts. In addition, both living species of sirenian have the same unique mode of tooth replacement that elephants have. Instead of pushing out baby teeth from below, as most mammals do, elephants and sirenians have horizontal tooth replacement. Their tooth row consists of a long “conveyer belt” of molars, with new teeth erupting from the back of the jaw, then pushing the rest of the tooth row forward until the older worn teeth are dropped off the front edge of the mouth. This unique condition occurs in no other group of mammals and is strong evidence for a clade of Proboscidea plus Sirenia that McKenna (1975) called the “Tethytheria.”
Since McKenna’s original daring hypothesis, the Tethytheria has been supported by many additional anatomical analyses, so the relationship of manatees and elephants is one of the best established in all of science. Every molecular system that has been examined, from the proteins to the mitochondrial DNA to the nuclear DNA always clusters these two groups together, so there is a complete convergence of evidence. In addition to the sirenians, several other previously mysterious groups of fossil mammals now appear to cluster within the Tethytheria. They include the huge two-horned elephant-sized beasts from the Oligocene of Egypt known as arsinoitheres (fig. 14.19). These had once been a complete zoological mystery, placed in their own order Embrithopoda for lack of a better place. But McKenna and Manning (1977) linked them to the Paleocene Mongolian mystery fossil known as Phenacolophus, and since then other arsinoitheres have been found in Eocene beds of Turkey and Romania. Yet another mysterious group was the desmostylians, a hippo-like marine mammal that is found only in the Oligocene and Miocene of the North Pacific. These too were placed in their own order for lack of a better hypothesis, until Domning, Ray, and McKenna (1986) described very primitive fossils of desmostylians called Behemotops and showed that they had the characteristic tethythere configuration of the jaws and teeth. Once again, the fossil record has yielded a transitional form that links a previously isolated group with other groups of mammals.
Finally, let’s return to the manatees, peacefully sleeping in the shallow warm waters of the tropics and munching sea grasses. According to some historians, the legend of the mermaids may have come from sailors who saw manatees floating upright, feeding their babies at their paired breasts (a configuration also found in humans and elephants) and possibly with seaweed draped over them that resembled hair, and imagined that they were mermaids. Close up, of course, they are so plug-ugly that they could never be mistaken for beautiful half-women/half-fish, but never underestimate what months at sea can do for homesick and horny sailors! But this myth, and the legend of the sirens trying to lure Odysseus’s sailors to their doom with their beauty and seductive songs, is the basis for the name of the order, Sirenia.
When we look at manatees up close, they have many remarkable specializations. Their skulls exhibit many unique features, especially in the way the upper bones are modified into a snout. They have horizontal tooth replacement, and some also have short tusks as well. Their ribs are unique among mammals in that they are extremely dense and heavy (pachyostotic). These act as diving ballast and help keep the manatee floating at the proper depth. Last but not least, the front limbs are modified into a flipper (different from the detailed bone configuration found in whale or ichthyosaur flippers), the hind limbs have vanished completely, and the tail is a broad flat horizontal fluke like that found in whales. Its shape is rounded in manatees, but with pointed lobes in dugongs.
Sirenian fossils are well known, although they consist mostly of their distinctively dense and heavy rib fragments, plus a few decent skulls that show their evolution (Domning 1981, 1982). But in 2001, another remarkable transitional form was discovered that clearly catches the sirenians in the act of evolving from land mammals. Known as Pezosiren portelli (literally, “Portell’s walking sirenian”) (fig. 14.21), it is a nearly complete skeleton from the Eocene of Jamaica that was described by Daryl Domning (2001). The skull is much like many other primitive sirenians, with all the hallmarks in the skull bones and teeth, and the ribs are thick and heavy, showing that it too was mostly aquatic. But instead of flippers it has four perfectly good walking limbs, with strong shoulder girdles, hip bones, and even well-developed hands and feet! One could not imagine a better transitional form: a creature with all the skull and skeletal features of manatees, yet it still has the ability to walk on land.

FIGURE 14.21. The mounted skeleton of Pezosiren portelli, the manatee with feet rather than flippers, next to Daryl Domning, who described and named it. (Photo courtesy Raymond L. Bernor)
In many respects, Pezosiren is comparable to Ambulocetus, which is a beautiful example of a walking whale making the transition to aquatic life, and to the enaliarctines, which are the transition fossils linking terrestrial bears to seals and other pinnipeds. Just a few years ago, we had no transitional forms to show how terrestrial ancestors of marine mammals like sirenians, whales, and seals went back to the sea and became aquatic, and now we have excellent fossil transitions for all three groups! The creationist websites have tried to discredit Pezosiren, but reading their discussions just shows how laughably incompetent they are in anatomy or paleontology. Their basic argument boils down to the idea that because it was terrestrial, it couldn’t be a sirenian! They fail to notice all the uniquely sirenian features of the skull (particularly in the snout and teeth, with their horizontal tooth replacement) and especially the heavy ribs for ballast, showing that this creature was hippo-like with both terrestrial and aquatic features—just as we would expect for a form making the transition between terrestrial and aquatic lifestyles. To a creationist, it is either in the fully aquatic sirenian “kind” or it’s some other sort of terrestrial mammal, but their conceptual blinders make it impossible for them to recognize a fossil that is perfectly intermediate between two of their “created kinds.” Pezosiren is the stuff of creationist nightmares, because you couldn’t ask for a better transitional fossil: half manatee, half walking land mammal.
Thus we have seen that the fossil record of hoofed mammals is full of transitional forms, showing how nearly all the familiar large ungulates (horses, rhinos, giraffes, elephants, and so on) evolved and how two groups of marine mammals (whales and sirenians) evolved from land ancestors. Even more examples are given in my new book, The Princeton Field Guide to Prehistoric Mammals. In the previous chapter, we saw a plethora of transitional forms among the other groups of placental mammals, and if we had more space, we could provide them for nearly every group. But it’s time to get to the real $64,000 question that interests us the most: what about us humans? That will be the subject of our chapter 15.
For Further Reading
Benton, M. J., ed. 1988. The Phylogeny and Classification of the Tetrapods. Vol. 2, Mammals. Oxford, U.K.: Clarendon.
Benton, M. J. 2014. Vertebrate Palaeontology. 4th ed. New York: Wiley-Blackwell.
Carroll, R. L. 1988. Vertebrate Paleontology and Evolution. New York: Freeman.
Janis, C., K. M. Scott, and L. L. Jacobs, eds. 1998. Evolution of Tertiary Mammals of North America. Vol. 1, Terrestrial Carnivores, Ungulates and Ungulate-like Mammals. New York: Cambridge University Press.
Janis, C., G. F. Gunnell, and M. D. Uhen, eds. 2008. Evolution of Tertiary Mammals of North America. Vol. 2, Small Mammals, Xenarthrans, and Marine Mammals. New York: Cambridge University Press.
MacFadden, B. J. 1992. Fossil Horses. New York: Cambridge University Press.
McKenna, M. C., and S. K. Bell. 1997. Classification of Mammals. New York: Columbia University Press.
Novacek, M. J. 1992. Mammalian phylogeny: shaking the tree. Nature 356: 121–125.
Novacek, M. J. 1994. The radiation of placental mammals. In Major Features of Vertebrate Evolution, ed. D. R. Prothero and R. M. Schoch. Paleontological Society Short Course 7:220–237.
Novacek, M. J., and A. R. Wyss. 1986. Higher-level relationships of Recent eutherian orders: morphological evidence. Cladistics 2:257–287.
Prothero, D. R. 1994. Mammalian evolution. In Major Features of Vertebrate Evolution, ed. D. R. Prothero and R. M. Schoch. Paleontological Society Short Course 7:238–270.
Prothero, D. R. 2005. The Evolution of North American Rhinoceroses. New York: Cambridge University Press.
Prothero, D. R. 2006. After the Dinosaurs: The Age of Mammals. Bloomington: Indiana University Press.
Prothero, D. R. 2013. Bringing Fossils to Life: An Introduction to Paleobiology. 3rd ed. New York: Columbia University Press.
Prothero, D. R. 2016. The Princeton Field Guide to Prehistoric Mammals. Princeton, N.J.: Princeton University Press.
Prothero, D. R., and S. Foss, eds. 2007. The Evolution of Artiodactyls. Baltimore, Md.: Johns Hopkins University Press.
Prothero, D. R., and R. M. Schoch, eds. 1989. The Evolution of Perissodactyls. New York: Oxford University Press.
Prothero, D. R., and R. M. Schoch. 2002. Horns, Tusks, and Flippers: The Evolution of Hoofed Mammals. Baltimore, Md.: Johns Hopkins University Press.
Rose, K. D., and J. D. Archibald, eds. 2005. The Rise of Placental Mammals. Baltimore, Md.: Johns Hopkins University Press.
Savage, R. J. G., and M. R. Long. 1986. Mammal Evolution: An Illustrated Guide. New York: Facts-on-File.
Szalay, F. S., M. J. Novacek, and M. C. McKenna, eds. 1993. Mammal Phylogeny. New York: Springer-Verlag.
Thewissen, J. G. M., ed. 1998. The Emergence of Whales: Evolutionary Patterns in the Origin of Cetacea. New York: Plenum.
Turner, A., and M. Anton. 2004. National Geographic Prehistoric Mammals. Washington, D.C.: National Geographic Society.