Sexual selection arises from the differential ability of individuals to obtain sexual partners and fertilize gametes. It is the “other form” of selection explicated by Darwin (1871). Unlike natural selection (Darwin 1859), which tends to forge adaptations in response to environmental demands, sexual selection tends to mold behaviors and other phenotypes to meet mating demands. As Darwin recognized, sexual selection is tightly interwoven with many other reproductive topics, including mating behaviors, mating systems, sex ratios, and sexual dimorphism. Even a century and a half after Darwin (Avise and Ayala 2009), modern extensions of sexual-selection theory continue to present empirical and conceptual challenges to evolutionary biologists (Eberhard 2009; Jones and Ratterman 2009; Shuster 2009). This chapter illustrates such research by focusing on sexual selection in relation to pregnancy-like phenomena in fish.
As intimated in chapter 1, anisogamy—the asymmetry in size and motility between male and female gametes—has many evolutionary implications for mating systems, modes of pregnancy, and the operation of sexual selection. These ramifications include the following (Avise 2010), all of which apply broadly (but not universally) to diverse animal taxa:
1. greater potential fecundity (fertility) for individual males than for females. Because sperm cells are relatively tiny and inexpensive to produce, each male can typically produce vastly more gametes than can a female.
2. a gender bias toward male pursuit of multiple mating. By securing multiple mates, a male is likely to produce many additional offspring, whereas a female is not. The statistical regressions that describe empirical relationships between mate numbers and progeny output are called sexual-selection gradients or Bateman gradients (box 7.1). The slopes in these regressions are steeper for males than for females in many species (especially those with female pregnancy). Notable exceptions include some male-pregnant pipefishes (described later).
BOX 7.1 Sexual-Selection Gradients
The relative intensities of sexual selection on males and females in various species have been attributed to several influences, including differences in parental investment by the two sexes (Parker and Simmons 1996; Trivers 1972); operational sex ratio (of sexually mature males to females; Kvarnemo and Ahnesjö 1996); relative variances in reproductive success in the two genders (Payne 1979; Wade and Arnold 1980; Wade and Shuster 2004); and the two sexes’ potential reproductive rates (Clutton-Brock and Parker 1992; Clutton-Brock and Vincent 1991). In a classic paper, Angus Bateman (1948) argued that all such influences can be subsumed under one overarching factor: the average relationship between an individual’s mating success (number of mates) and the number of offspring the individual produces (reproductive success or genetic fitness).
In experimental populations of fruit flies, Bateman documented that males’ mean genetic fitness increased rapidly as a function of mate numbers, yielding a steep sexual-selection gradient, whereas females’ genetic fitness did not increase nearly as rapidly with higher mate counts, yielding a nearly flat selection gradient (see the accompanying graph). Bateman interpreted this disparity as sexual selection’s true cause: multiple mating pays higher fitness dividends to males than to females.
The slopes in sexual-selection gradients (or Bateman gradients) are now routinely used to quantify and compare the relative intensities of sexual selection on males and females (Andersson and Iwasa 1996; Arnold and Duvall 1994; Jones et al. 2000, 2002; Lorch et al. 2008). Although Bateman’s approach to quantifying sexual selection has several limitations (Tang-Martinez and Ryder 2005; Snyder and Gowaty 2007), many researchers now accept the basic thrust of Bateman’s argument: reproductive success in males tends to be limited by access to mates, whereas in females it tends to be limited by access to resources (Jones and Ratterman 2009).

None of this is to imply that females never benefit from multiple mating. Direct benefits that might accrue to a female from having multiple partners include more nuptial gifts, improved territorial access, or additional help with parenting. A female might also receive “indirect benefits” (Jennions and Petrie 2000; Zeh and Zeh 1996, 1997, 2001; Tregenza and Wedell 2000) from multiple mating when she thereby gains higher genetic diversity in her clutch; greater opportunities for “good genes” in her progeny (Yasui 1998, 2001); “fertilization insurance” against the possibility that some males might be sterile; and/or a reduced risk of sexual harassment (Birkhead and Pizzari 2002). However, none of these factors is likely to boost a female’s genetic fitness nearly as much as multiple mating by a male can normally boost his genetic fitness. Of course, multiple mating by either sex also carries costs, such as the time and energy involved in finding mates and the higher possibility of contracting a sexually transmitted disease.
3. a bias toward the evolution of polygyny rather than polyandry. Polyandry (multiple mating exclusively by the females of a species) is relatively rare in the biological world, whereas polygyny (multiple mating predominantly by males) is common. This makes evolutionary sense given that Bateman gradients are often shallower for females than for males. However, the rarity of polyandry also has to do with asymmetrical assurances of genetic parentage. Especially in viviparous taxa such as mammals, a female can be certain that she is the biological mother of the offspring she nurtures, whereas a male has no comparable assurance of paternity. Because any male that enters into an exclusive relationship with a polyandrous female runs the risk of being cuckolded by a competitor, natural selection tends to disfavor the evolution of male acquiescence to mate with and care for a single polyandrous partner and her young.
4. a gender bias toward the evolution of postzygotic parental care (including pregnancy) by females. Anisogamy generally predisposes females more so than males to invest additional energy and resources in rearing offspring, a disparity that becomes exaggerated in viviparous species with female pregnancy. Thus, anisogamy biases evolution toward the emergence of gestation by females. Furthermore, female pregnancy can then amplify the following biological tendencies that anisogamy ultimately initiated.
5. a gender bias toward higher variation in reproductive success among males. Especially in species with polygynous mating systems, some males are huge winners in the reproductive sweepstakes, while other males may fail miserably, resulting in higher intermale than interfemale variances in reproductive output. By contrast, in any species with lifelong monogamy for all mating pairs, intermale and interfemale variances in genetic fitness are identical.
6. a gender bias toward male competitiveness for mates. This expectation applies especially to polygamous species, and it should also apply more strongly to males in polygynous taxa than to females in polyandrous taxa. However, this is not to imply that either gender in a monogamous species is immune to sexual selection because each individual in any mating system can presumably improve its genetic fitness by choosing a high-quality mate. Nevertheless, anisogamy (alone or especially when coupled with female pregnancy) generally makes females a limiting procreative resource, for which males compete for sexual access.
7. a gender bias toward sexual selection on males. This follows directly from the preceding item and again applies with special force to species with polygynous mating systems.
8. a gender bias toward the elaboration of secondary sexual traits in males. This follows directly from the preceding item and also applies with special force to polygynous species.
Figure 7.1 graphically summarizes these points, which collectively reflect conventional evolutionary wisdom about many standard connections between mating behaviors, sexual selection, sexual dimorphism, and mating strategies (Shuster and Wade 2003; Oliveira et al. 2008). However, none of these extended ramifications of anisogamy are universal evolutionary outcomes in the heterogeneous biological world (Bonduriansky 2009). For example, males in some species become pregnant (Vincent et al. 1992; Jones and Avise 2001), in which case many of the basic evolutionary ground rules shift dramatically. Nevertheless, each biological expectation listed earlier is met in a sufficiently wide array of taxa as to make the exceptions particularly informative. This is why the relatively few species that display male pregnancy and “sex-role reversal” are fascinating subjects for evolutionary research (see later discussion).
Modern researchers follow Darwin (1871) in dividing sexual selection into two broad categories: intersexual and intrasexual. The latter (sometimes called “male-male competition”) typically favors males that are good fighters, stalwart defenders of territory, or otherwise stiff competitors against other males in their ability to secure mates. Male-male competition has resulted in the evolution of impressive traits such as huge horns on the heads of bighorn rams, fighting spurs on gamecocks, and combative behavior in Siamese fighting fish. For champion males, the ultimate fitness payoff is more offspring via enhanced mating success. By contrast, intersexual or epigamic selection (sometimes abbreviated “female choice”) typically operates by favoring males that females find attractive as sexual partners. It, too, has resulted in the evolution of many impressive phenotypic features such as showy peacock tails, colorful fins on guppies, and elaborate courtship displays by males in many species, including fishes. Again, the reproductive payoff for showier males is additional progeny as a result of greater mating success.
Sexual selection of either sort can be a powerful evolutionary force that promotes striking intraspecific sexual dimorphism (consistent differences between conspecific males and females) in secondary sexual traits (gender-specific phenotypes other than the gonads). However, unless experiments or critical observations are made, it can be difficult to know in specific instances whether dimorphism in a secondary sexual trait arose via intersexual or intrasexual selection (or both). Do the showy fins of male guppies register the effects of male-male competition or female choice (or in this case perhaps artificial selection by human aquarists)? Another point is that sexual selection and natural selection often act in opposition on a trait. Whereas a showy caudal fin may enhance a male guppy’s attractiveness to females, it might also compromise his swimming ability and make him more vulnerable to starvation or predation. In each such case, evolution in essence strikes a compromise between the benefit of winning mates and the detriment of attracting predators (Endler 1991).
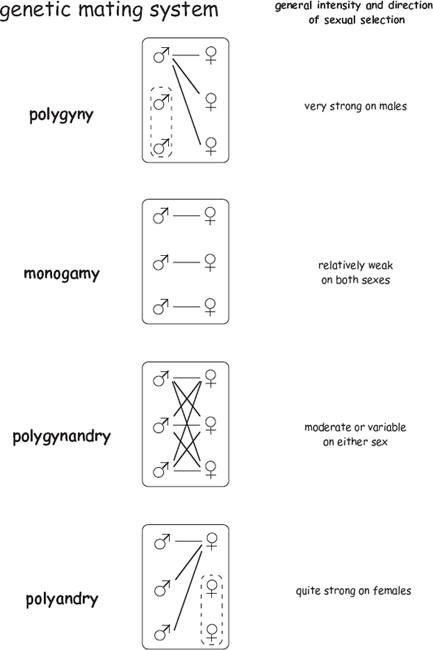
FIGURE 7.1 Four mating systems and their purported relationships to sexual selection and gender dimorphism in secondary sexual traits (modified from Avise et al. 2002). Solid lines indicate either “pair bonds” or successful mating events (depending on whether the reference is to the “social mating system” or to the “genetic mating system” of a population). Although the mating systems as depicted here are population-level phenomena, the terms can also apply to the mating behaviors of individuals. Thus, a female might be polyandrous or monogamous, depending on whether she has multiple mates or just one, and a particular male might show either polygynous or monogamous mating tendencies.
Factoid: Did you know? Many superfancy goldfish can barely swim at all, but this undoubtedly reflects extreme artificial selection by human aquarists more so than extreme sexual selection by other goldfish.
In principle, the genetic mating system of a population or species is pertinent to the direction and intensity of both intra- and intergender sexual selection. For example, in a population with a balanced sex ratio and a polygynous mating system, some males inevitably go without sexual partners, whereas others may obtain several mates, thus leading to a pronounced competition for females that can translate into strong sexual selection and the evolution of secondary sexual traits in males. Conversely, in a population with a 1:1 sex ratio and a polyandrous mating system, some females fail to mate, whereas others are multiple maters, thus driving sexual selection on females and promoting the evolution of secondary sexual traits in that sex. However, the disparity between the genders in the intensities of sexual selection (and in the elaboration of secondary sexual traits) is seldom expected to be as strongly female biased under polyandry as it is to be male biased under polygyny because anisogamy implies that the fecundity of females in most species remains inherently more limited than that of males. In effect, this gender-based asymmetry of fertility becomes even more pronounced in species with female pregnancy.
Notwithstanding the general bias toward female choosiness, active mate choice by males is also common in many animal species (Gwynne 1991; Gwynne and Simmons 1990; Gowaty and Hubbell 2009). This should come as no great surprise because individuals of both sexes surely are under selection to seek the best possible mates regardless of other circumstances. For both sexes, such a mate might be of high quality because the mate confers fitness benefits of either of two general types: better genes for the offspring or higher-quality care (more material resources) for its mate and/or for the resulting progeny. Despite this reality, it also remains true that females in most species exercise much of the authority over mate choice (chapter 1). Furthermore, especially for males, any fitness boosts obtained from acquiring a high-quality mate are likely to remain lower than those that might otherwise come from mating with multiple sexual partners (even if some of the latter are somewhat lower in quality).
Another way to dichotomize sexual selection is to take into account whether female “mate choice” takes place before or after copulation (Birkhead and Pizzari 2002; Birkhead 2010). For polyandrous species with internal fertilization, sperm from two or more males routinely co-occur within a female’s body. Growing evidence suggests that the reproductive tract of a female often plays a more active role than previously supposed in the postcopulatory choice of fertilizing sperm (Birkhead and Møller 1992, 1993; Mack et al. 2003). Furthermore, prolonged female sperm storage (a phenomenon known to occur in many viviparous organisms, including some live-bearing fishes; Liu and Avise 2011) probably enhances the opportunity for such “cryptic female choice.”
Multiple mating by a female also enhances the opportunities for postcopulatory sperm competition wherein gametes from different males undergo selection for traits (such as faster swimming speed or greater longevity within the female’s reproductive tract) that might improve their odds of successfully fertilizing eggs. Many reproductive phenotypes of males and their ejaculates (including sperm motility itself) can be interpreted as adaptations to meet the genetic challenges of direct or indirect competition with other males’ sperm (Smith 1984).
From a female’s perspective, mechanisms to prevent competition among sperm from different males are not necessarily desirable (Keller and Reeve 1995), and this can lead to reproductive conflicts of interest between the sexes (Eberhard 1998, 2009; Knowlton and Greenwell 1984). These and related discoveries about postcopulatory phenomena have made sperm competition and cryptic female choice major research arenas in the last several decades (Parker 1970; Smith 1984; Baker and Bellis 1995; Birkhead and Møller 1992, 1998).
In Darwin’s era, the concept of intersexual selection was slower to gain general acceptance than was natural selection, probably because many people found it hard to believe that females in “lower” animals have the perceptive wherewithal to choose mates with special phenotypic adornments. Today, most researchers recognize that females in many species do indeed exercise refined choices of alternative traits in their sexual partners, and the evolutionary details of such choosiness have sometimes been worked out to varying degrees. One case in point involves female-pregnant platyfishes and swordtails (Xiphophorus), an ensemble of more than 20 species of popular aquarium fishes native to Central America.
FIGURE 7.2 The swordtail fish, Xiphophorus maculatus, a live-bearing member of the Poeciliidae. The elongate caudal fin of the male is not an intromittent organ but rather an ornamental device that apparently evolved via sexual selection.
With respect to body form, all platys and swordtails look quite alike, and the most obvious difference is the presence in mature male swordtails of a long caudal fin resembling a cutlass (fig. 7.2). Researchers have documented that female swordtails prefer to mate with long-tailed males, thus implying that intersexual selection was probably responsible for the evolution of this striking phenotypic feature (Basolo 1990, 1995; Endler and Basolo 1998).
Interestingly, platyfish females also prefer to mate with long-tailed males when given the option, as was shown by laboratory experiments in which researchers surgically grafted plastic prostheses onto platyfish males that otherwise lacked long tails. These observations raised a chicken-or-egg question: which came first in Xiphophorus evolution—male swords or female preferences for male swords? Clues to the answer came from phylogenetic analyses. If platys were ancestral to swordtails, this would be consistent with the “preexisting-bias” hypothesis (Basolo 1995), which states that female preference for long tails came first in evolution; however, if swordtails were ancestral to platys, then swords (but not female preference for them) might later have been lost in the derived platyfish clade.
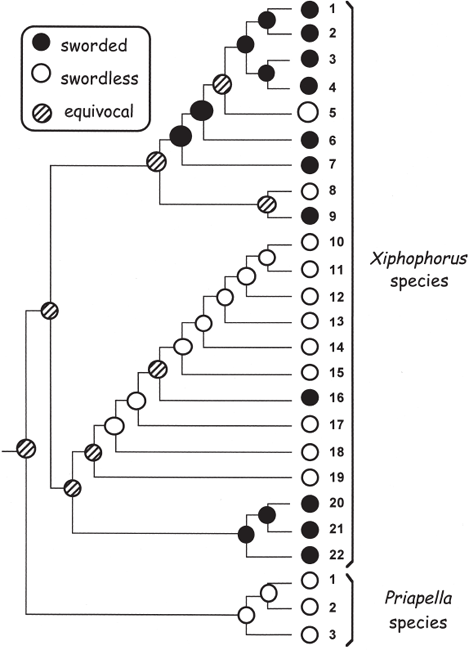
FIGURE 7.3 Evolution of caudal phenotypes in 25 species of swordtails, platyfishes, and their allies (after Schluter et al. [1997], based on the molecular phylogeny from Meyer et al. [1994]). Pie diagrams indicate the reconstructed likelihoods (from PCM analyses; see box 1.6) of alternative tail conditions at various nodes in the phylogenetic tree.
To address these issues, a molecular phylogeny for Xiphophorus species was generated and employed as a backdrop (fig. 7.3) for deducing evolutionary histories of the various phenotypes. This exercise in phylogenetic character mapping (PCM) revealed that swordless and swordtailed species are intermingled in the phylogenetic tree and that neither assemblage forms a coherent clade. Thus, evolutionary transitions between presence and absence of swords appear to have been both rapid and recurrent within the genus, thereby compromising attempts to determine the ancestral state of this clade (Meyer et al. 1994). Nevertheless, outlier species in the sister genus, Priapella, consistently lack a sword, so the shared ancestor of the more ancient Priapella + Xiphophorus clade was probably swordless. Behavioral experiments have shown that females of some Priapella species also prefer to mate with males adorned with long prosthetic tails. Thus, overall, the available data provide substantial evidence for the preexisting-bias hypothesis, in which a female preference for swords predated the evolutionary appearance of the swords themselves.
The phylogenetic reconstruction in figure 7.3 also indicates that swords were both lost and gained on multiple occasions within the Xiphophorus clade. This suggests that swordlike tails, despite their attractiveness to females, are otherwise a substantial burden for the males. Maybe the swords are energetically costly to produce, or perhaps they compromise the males’ agility. Laboratory experiments have confirmed that males with long swords do indeed expend more energy during normal swimming than do males without these encumbrances (Basolo and Alcarez 2003).
In one piscine taxonomic family—Syngnathidae—males (rather than females) become pregnant. In all of the 200-plus living species of pipefishes (fig. 7.4) and seahorses (fig. 7.5), males house the developing embryos. The process begins when a gravid female transfers some or all of her many eggs to the male’s abdomen or tail, where the eggs are either glued onto his external surface or deposited into a specialized pouch that evolved expressly for this purpose. In species with pouches, the male then fertilizes the clutch internally, seals the pouch, and carries the embryos for several weeks before giving birth to live young. During this pregnancy, the sire nourishes (Ripley and Foran 2009), aerates, osmoregulates (Ripley 2009), and protects his brood, whereas the mother plays no direct role in offspring care.
Pipefishes and seahorses are evolutionary cousins of sticklebacks (fig. 7.6), another group of fishes traditionally placed in the order Gasterosteiformes (Kawahara et al. 2008). In sticklebacks, an adult male builds a globular nest in which he may care for the clutches of several females simultaneously. Thus, one possibility is that the evolution of internal male pregnancy in the syngnathid lineage entailed a shift from the ancestral stickleback type of parental care (within a nest) to the pipefish type of internal parental care (within a male), with an intermediate stage wherein a female’s eggs were probably glued to the exterior of a male’s body. Two other possibilities not yet excluded by available phylogenetic evidence (Wilson et al. 2003; Kawahara et al. 2008) are that the syngnathid ancestor either displayed no parental care or perhaps practiced female brooding.
FIGURE 7.4 A pregnant male pipefish.
Factoid: Did you know? Like a mammalian womb, a syngnathid brood pouch can be an active site for postcopulatory sexual selection and parent-offspring conflict. For example, Paczolt and Jones (2010) show that pregnant males increase abortion rates in pregnancies from unattractive mothers, presumably thereby retaining their resources for future reproductive opportunities.
Although a higher assurance of paternity via internal fertilization likely played a selective role in the evolutionary transition to an enclosed brood pouch, other selection pressures may have come into play as well. For example, Andersson (2005) suggests that a loss of swimming agility in an ancestral nesttending species became exaggerated when early syngnathids evolved a slim snout for ambush hunting of small prey. As poor swimmers, these fish would have been vulnerable to predation, and in response they presumably evolved a cryptic morphology and began carrying eggs deeper within their bodies for safekeeping. These developments in turn truncated male fecundity and set the evolutionary stage for females to outpace males in reproductive capacity. Over evolutionary time, as the eggs and embryos became housed securely inside sires, these gender biases were exaggerated, eventuating in the situation observed today, in which female pipefish often experience sexual selection as they compete for mating access to the limiting reproductive resource: available brood space inside receptive males.

FIGURE 7.5 A pregnant male seahorse giving birth to live young.
FIGURE 7.6 The three-spined stickleback, Gasterosteus aculeatus, a nest-tending evolutionary cousin of pipefishes and seahorses.
Factoid: Did you know? A stickleback’s kidney secretes a gluelike protein that the male uses to build his nest from vegetable material.
The structure of the male’s brood pouch varies greatly among extant syngnathid species. At one end of the spectrum are fortified brood pouches with physical complexity (often including placenta-like features). This condition is characteristic of extant seahorses. At the other end of the spectrum are relatively simple, unprotected brooding areas on a male’s ventral surface, where eggs are glued but not encased. This condition is found in a few pipefish species. Between these two extremes are other pipefish species with either thin membranous compartments surrounding each egg or partially enclosed ventral pouches with protective coverings that extend across multiple eggs and embryos.
Wilson et al. (2003) address the evolutionary history of male brood pouches by mapping the phylogenetic distributions of alternative pouch designs on an mtDNA phylogeny for more than 30 syngnathid species (fig. 7.7). In this example of phylogenetic character mapping, a good agreement was found between clade membership and brood-pouch morphology. For example, all surveyed members of the Syngnathus pipefish clade possess closed pouches with two bilateral skin folds grown together, whereas seahorse species in the Hippocampus clade were unique in having a full saclike pouch enclosed by a single covering. On the other hand, some other pouch designs recurred in separate branches of the phylogenetic tree, thus suggesting independent evolutionary origins. Overall, the molecular phylogeny was consistent with the idea that pouches with simple designs evolutionarily predated more complex pouches.
One might suppose that male pregnancy itself should qualify syngnathids as being sex-role reversed relative to mammals. However, much of the scientific literature defines sex-role reversal as occurring whenever sexual selection operates more intensely on females than on males. By this criterion, some syngnathid species are sex-role reversed, but others are not. In some syngnathid species, females collectively produce many more eggs than males can accommodate in their brood pouches, thus making males a limiting reproductive resource for which females presumably compete. Sex-role reversal by this definition has other ramifications: females in such species should have polyandrous mating tendencies and should be the sex that displays sexually selected phenotypes. All of these properties differ diametrically from the biological situation in most other species, in which females are a limiting resource such that males experience stronger sexual selection and evolve elaborate phenotypes for attracting mates or battling among themselves for female access.
A vast scientific literature shows that the topics of sexual behavior, gender ratios, sexual selection, sexual dimorphism, and mating systems are all intertwined. To sort through some of this complexity for syngnathids, Wilson et al. (2003) also used their molecular phylogeny (see fig. 7.7) to address the evolutionary histories of sex-role reversal and alternative mating systems in male-pregnant fishes. One possibility the authors explored was that fancy brood pouches predict sex-role reversal because males with such high-investment pouches might be an even more limiting resource to mate-prospecting females. However, this possibility was not confirmed by the PCM analyses, which showed instead that sex-role-reversed clades were present in both simple-pouch and complex-pouch regions of the syngnathid phylogeny. Perhaps different brood-pouch designs are not reliable indicators of male investment in offspring care.
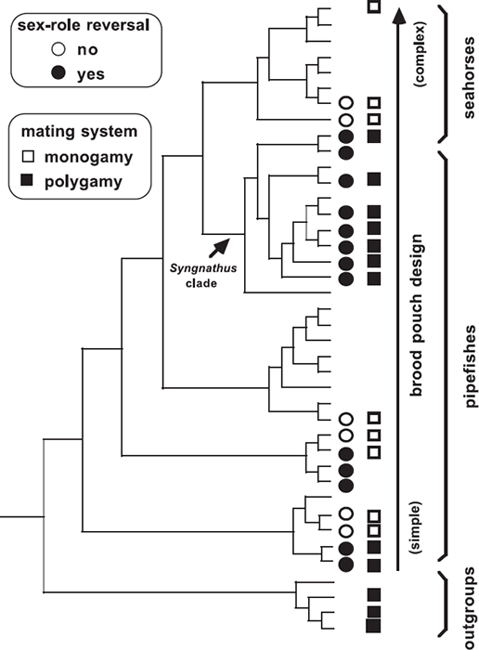
FIGURE 7.7 Molecular phylogenetic tree for 36 syngnathid species and four outgroup taxa (after Wilson et al. 2003). Mapped onto terminal nodes of the tree are the distributions of monogamous and polygamous mating systems and presence versus absence of sex-role reversal among extant species.
The PCM analyses by Wilson et al. (2003) do, however, support another evolutionary hypothesis: that sex-role reversal in syngnathid species tends to be associated with polyandry. For example, all Syngnathus pipefishes for which genetic information is available are both polygamous and sex-role reversed (see fig. 7.7), whereas all such Hippocampus seahorse species are monogamous and lack sex-role reversal according to the sexual-selection definition.
Male pregnancy, a phenomenon foreign to mammals, affords novel vantage points on animal reproductive behaviors. Apart from PCM, another comparative approach applied to numerous syngnathid species (Jones and Avise 2001) has involved genetic-parentage analyses via molecular markers (see the appendix). The general intent of this approach is to address the relation of sexual selection and sexual dimorphism to alternative genetic mating systems. For example, in some or all species of male-pregnant syngnathids, polyandry might be common, and sexual selection on females (via competition for mates) might actually be more intense than on males such that females evolve brighter colors or other special body ornaments. Indeed, during the breeding season females in many pipefish species become festooned with secondary sexual traits, a reversal of the typical situation in birds and mammals, in which males are the adorned sex. In theory, the keenness of female competition for mates should also relate to the genetic mating system of each pipefish and seahorse species. For example, sexual selection on females might be most intense in polyandrous species because any female who successfully woos several choosy males might markedly improve her genetic fitness (especially if she can produce more eggs than a male can typically incubate).
Almost all of the genetic-parentage analyses on syngnathids were conducted on broods of pregnant males collected from nature. Examples of the kind of maternity data that emerge from such analyses are shown in figure 7.8. As expected (given intrapouch fertilization), no instances of cuckoldry were detected in the dozens of syngnathid litters analyzed, meaning that each pregnant sire has a very high assurance of paternity for the offspring he carries. This certainty of paternity in the Syngnathidae in turn facilitated molecular analyses of biological maternity and genetic mating systems (because the dam’s genotype for each gestating embryo could be determined by subtracting known paternal alleles from each offspring’s diploid genotype).
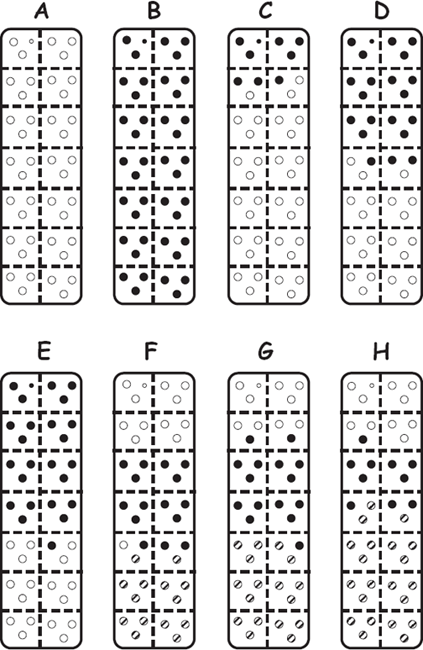
FIGURE 7.8 Diagrams of results from genetic-parentage analyses as applied to pipefishes (after Jones and Avise 1997b). Shown are embryos (small circles) within the brood pouches (large rectangles) of eight pregnant S. floridae males. Each pregnant male proved to have sired all of the embryos within his pouch, and 1–3 females contributed to a given brood. For example, the brood pouch of sire H housed embryos from three dams (open, closed, and lined circles), whereas sires A and B had only one successful mate each. Such data accumulated for many broods helped to reveal the population’s genetic mating system.
Factoid: Did you know? Whenever a pregnant pipefish male proved (by genetic-parentage analyses) to have mated with multiple females, the different full-sib clutches in his pouch were arranged in a neatly stacked configuration, suggesting that eggs received from successive mating events sink toward the bottom of his pouch.
From this type of molecular information on biological parentage, several different genetic mating systems ranging from monogamy to polygynandry to polyandry were uncovered in different syngnathid species (Jones and Avise 2001). Furthermore, when these genetic mating systems were interpreted in the context of observed levels of sexual dimorphism and the presumed intensities and directions of sexual selection in the surveyed species, the overall results appeared compatible with conventional wisdom (as summarized in fig. 7.1) for taxa that include species with proclivities toward polyandry and sex-role reversal. Thus, those assayed syngnathid species that proved to have polyandrous or polygynandrous genetic mating systems displayed greater sexual dimorphism than did the monogamous species. Furthermore, as might have been predicted for the sexually dimorphic syngnathids, it was invariably the female gender that exhibited the more pronounced development of secondary sexual characteristics.
Another interesting finding emerged from genetic analyses of seahorses. From behavioral observations in nature, most seahorse couples seem highly devoted to one another. However, genetic markers proved that the progeny in a male’s successive broods often had different mothers. Evidently, seahorse couples sometimes “divorce” and “remarry” a variety of partners (yielding a mating system known as serial monogamy). In other words, although each pregnancy consisted of full-sib offspring (evidencing monogamy), individuals frequently switch partners during or between breeding seasons (in effect evidencing polygamy over time).
Factoid: Did you know? In some seahorse species, a mated male and female greet each other daily with ritualized behaviors that include head nods plus hugs with their prehensile tails.
FIGURE 7.9 Sexual selection gradients empirically documented in a male-pregnant pipefish species, Syngnathus typhle. Note that slopes in the regressions for male and female reproductive success are reversed (steeper for females) compared to those that typify female-pregnant and many other species (see box 7.1).
Finally, some of the genetic-parentage analyses in the pipefish Syngnathus typhle were conducted under controlled laboratory conditions where sex ratios and mating opportunities were manipulated experimentally (Jones et al. 2000, 2005). The most salient finding was that females in this polygynandrous pipefish species show higher fitness gains from multiple mating than do males. Thus, Bateman gradients in this male-pregnant species indeed are reversed (fig. 7.9) compared to the standard situation in female-pregnant species and other taxa with conventional sex roles.
Fish display diverse reproductive modes ranging from pelagic group spawning to social monogamy and from internal female pregnancy (as in Poeciliidae) to internal male pregnancy (in Syngnathidae). Collectively, this diversity provides rich fodder for comparative evolutionary investigations of alternative reproductive strategies (Avise et al. 2002). Of special relevance to this book on pregnancy is the topic of parental care, which across fish species ranges from nonexistent to extensive. Approximately 89 of 422 taxonomic families of bony fish (21%) contain at least some species that practice parental care of offspring, and, interestingly, in nearly 70% of such cases the primary or exclusive parental custodian is the male (Blumer 1979, 1982). Parental care in various fish species includes not only commonplace phenomena like nesting and oral brooding but also some rare and bizarre mechanisms such as that displayed by the nurseryfish (fig. 7.10). Exclusive paternal care of offspring is otherwise quite uncommon in the biological world, so fish offer mirror-image evolutionary perspectives on parental care compared to many other animal groups where females are the primary caregivers (Clutton-Brock 1991).
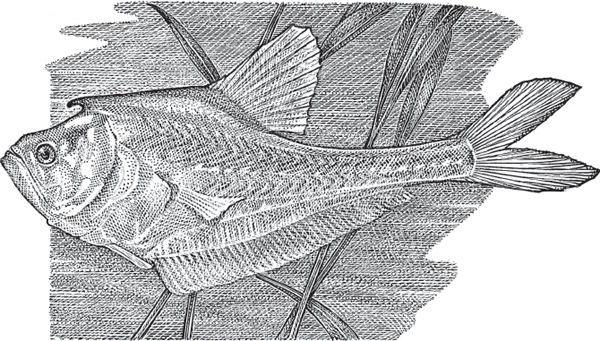
FIGURE 7.10 The nurseryfish, Kurtus gulliveti, an Australian species in which each male uses a special hook on his forehead to carry clusters of eggs (Berra and Humphrey 2002). How the eggs get transferred from the female (and fertilized) remains unknown.
Mouth brooding (or sometimes gill brooding) occurs in several piscine groups, including catfishes, lumpfish, cardinal fish, jawfish, and some blind cave fishes. However, nowhere has the phenomenon been studied more thoroughly than in the Cichlidae (fig. 7.11).
Factoid: Did you know? Some predatory cichlid fish ram the head region of a mouthbrooder, forcing the latter to spit out a few offspring, which the predator then eats (McKaye and Kocher 1983).
FIGURE 7.11 Mouth brooding behavior in cichlid fishes. Adults in many species in the family Cichlidae routinely safeguard eggs and young in their mouths. Depending on the cichlid species, oral brooding may be by sires, dams, or both parents.
FIGURE 7.12 Evolutionary transitions (as deduced from PCM analyses) between alternative modes of parental care of offspring in cichlid fishes with external fertilization (after Goodwin et al. 1998). Shown are the numbers of independent transitions between the following: (A) substrate guarding and mouth brooding; and (B) biparental, female-only, and male-only care.
Phylogenetic character mapping has focused on evolutionary transitions among alternative parental-care modes (including mouth brooding) in species representing nearly 200 cichlid genera (Goodwin et al. 1998). The results demonstrated that female-only care evolved many times from biparental care, whereas male-only care rarely did so (fig. 7.12). Results further showed that substrate guarding was the ancestral mode of parental care in Cichlidae, from which mouth brooding evolved on at least 10 occasions (fig. 7.12). Perhaps the transport of offspring between excavated pits by a substrate guarder provided a first step toward the evolution of mouth brooding, especially if the offspring were also retained in the adult’s mouth for some time to avoid predation (Baylis 1981). Additional research has shown that evolution of mouth brooding in cichlids is also associated with the loss of adhesive threads on eggs, reduced fecundity, increased egg size, and higher juvenile survival (Keenleyside 1991).
As described in chapter 2, when alternative modes of parental care are mapped onto molecular phylogenies of fishes, it becomes apparent that paternal care has arisen many times during piscine evolution, typically from ancestral states of external fertilization without parental care (see fig. 2.3). The evolution of any such gender-specific parental care in species with external fertilization is usually explained by the “proximity argument,” wherein the gender that is closest to the eggs at the time of fertilization tends to be the sex that is predisposed to care for the young, especially if the other sex then deserts or abandons the effort for any reason (Trivers 1972; Williams 1975; Dawkins and Carlisle 1976). Especially in fish species that practice male territoriality and nest guarding, the gender that is most proximate to the zygotes and the early embryos is typically the male. This proximity factor undoubtedly helps to account for the prevalence of male-only care in nest-tending fish species.
Especially when the magnitude and duration of paternal care are substantial, many nest-tending fish species display what can be thought of as “external male pregnancy” (Avise and Liu 2010, 2011). In such species, a “bourgeois” male typically constructs or adopts a nest, into which one or more females deposit eggs that the “shopkeeper” male may fertilize. Each bourgeois male then guards and tends the embryos for several weeks until the young leave the nest to begin independent lives. In effect, each nest-tending male assumes parental duties that in some ways parallel the time and energy expended by the gestating parent in viviparous fishes. Furthermore, genetic-parentage analyses (see appendix) can be applied to the broods of nest-tending fishes just as they can for broods in viviparous fish species with either internal male pregnancy or internal female pregnancy (fig. 7.13).

FIGURE 7.13 Examples of maternity revealed by molecular markers in a nest-tending fish species, Lepomis auritus (after DeWoody et al. 1998). Shown are embryos (small circles) at five sample locations within each of two nests tended by bourgeois males. The coded circles indicate that two females contributed to nest A and four females contributed to nest B. Such maternity assignments accumulated for many nests help reveal a population’s genetic mating system, which also might include instances of cuckoldry.
Factoid: Did you know? Fish nests have varied designs ranging from simple saucer-shaped depressions in the substrate (as in the sunfishes) to elaborate tunnel nests built above the substrate and consisting of woven and glued plant materials (as in refined nesters such as sticklebacks). Gouramis and Siamese fighting fish make froth nests consisting of masses of mucus-covered bubbles. Another odd type of fish nest is described in the caption to figure 7.14. A few fish species even lay their eggs in the nests of other nest-tending fish species (Helfman et al. 1997).
There are, nevertheless, several key differences between internal pregnancy and external pregnancy via nesting. For example, external pregnancy presumably entails fewer constraints on brood space (and therefore on progeny numbers) than does internal pregnancy, and this might have major consequences for the operation of sexual selection (chapter 8) and the evolution of piscine mating phenotypes and behaviors. External pregnancy also opens windows of opportunity for “stolen fertilizations” (cuckoldry events) that otherwise remain closed to viviparous species with internal fertilization. Such exposure to cuckoldry in nest-tending fishes in turn produces selection pressures that have led to an amazing array of “alternative reproductive tactics” (ARTs) by males (Taborsky 1994; Gross 1996; Henson and Warner 1997; Thomaz et al. 1997; Oliveira et al. 2008).
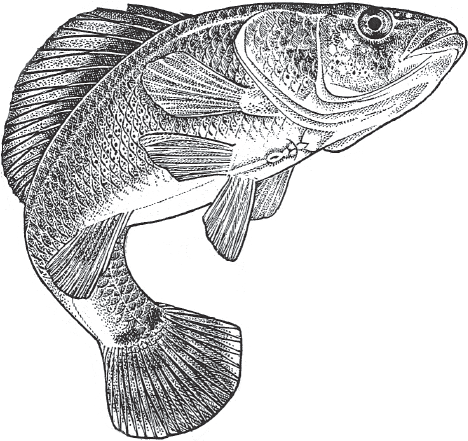
FIGURE 7.14 The pirate perch, Aphredoderus sayanus. In this freshwater species from swamps in the eastern United States, adults lay and fertilize eggs in tunnels excavated (by invertebrates) in the submerged balls of tree roots. The peculiar anterior position of the urogenital pore in the pirate perch allows each fish to enter a tunnel headfirst and spray its gametes into the root ball’s protective interior, where the zygotes and embryos then incubate without further parental involvement (Fletcher et al. 2004).
To introduce the topic of cuckoldry in fishes, consider the bluegill sunfish (fig. 7.15), a nest-tending species with several ARTs. Three types of bluegill males were found in surveyed populations of this species in Canada: (a) bourgeois males, which mature at about 7 years of age and then construct saucer-depression nests in colonies, attract and spawn with females, and vigorously defend the nest and embryos; (b) precocious sneaker males, 2–3 years of age, which dart into a bourgeois male’s nest and release sperm; and (c) older satellite males, which mimic females in color and behavior but also release sperm as the primary couple spawns in a bourgeois male’s nest (Gross and Charnov 1980). Genetic-parentage analyses (Colbourne et al. 1996; Neff 2001; Philipp and Gross 1994) have shown that about 20% of the offspring in a bluegill colony are foster progeny resulting from cuckoldry (fig. 7.16). Studies also have suggested that bourgeois males can detect lost paternity and adaptively lower their level of parental care accordingly (Neff and Gross 2001). Cuckoldry has been genetically documented in several other sunfish species as well (fig. 7.16), albeit typically at lower rates than in bluegills.
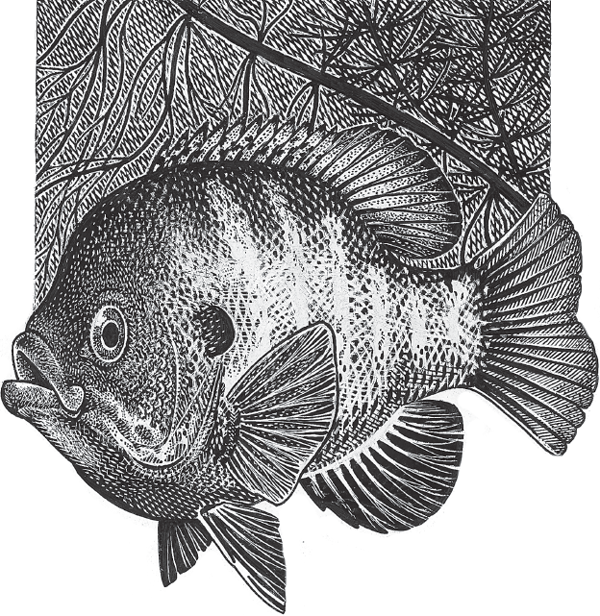
FIGURE 7.15 The bluegill sunfish, Lepomis macrochirus, one of many nest-tending species in the family Centrarchidae. In this species, various offspring in a nest might have been sired by the bourgeois male (the nest tender), sneaker males, or female-impersonator males (see text).
“Extrapair fertilization” (EPF) events that underlie foster parentage in nest-tending species (including birds and fishes) are generally thought to increase the intensity of sexual selection because they presumably often increase the overall variation in favorable male mating outcomes, especially if (as is often true) successful bourgeois males are among the effective cuckolders (Møller and Birkhead 1994). However, Jones et al. (2001) uncovered a converse case in which EPFs and ARTs probably decreased the intensity of sexual selection. For a marine goby (Pomatoschistus minutus), the authors showed by genetic-parentage analyses that sneaker males had fertilized eggs in nearly 50% of the assayed nests but also that, as a result, the overall intermale variance in reproductive success was lower than it probably would have been in the absence of such high rates of cuckoldry.

FIGURE 7.16 Molecular findings on genetic paternity in several species of sunfish (after Avise et al. 2002). Shown are percentages of progeny per nest that proved to have been sired by bourgeois males in: (A) 38 nests of Lepomis macrochirus; and (B) 104 nests of four other centrarchid species.
Similar molecular appraisals of genetic-parentage and mating systems in a wide variety of nest-tending fish species (see appendix) have been used in conjunction with behavioral observations to reveal other oft-cryptic reproductive phenomena, including those described in the following sections.
A given fish nest has often proved to contain half-sib offspring from multiple (typically 2–8) dams (DeWoody and Avise 2001). Such genetic evidence of multiple mating by bourgeois males has been gathered for several species of Lepomis sunfish (DeWoody, Fletcher, et al. 1998, 2000b; Mackiewicz et al. 2002), Etheostoma darters (DeWoody, Walker, et al. 2000; Porter et al. 2002), Spinachia sticklebacks (Jones, Östlund-Nilsson, et al. 1998), Pomatoschistus sand gobies (Jones et al. 2001), and Cottus sculpins (Fiumera et al. 2002), among others.
Two other behavioral avenues to nonpaternity by bourgeois males involve occasional nest takeovers and instances of egg piracy, which are provisionally evidenced when few or no offspring in a focal nest prove to have been genetically sired by the resident bourgeois male. Nest takeovers might be opportunistic responses by males to limited nest-site availability, or perhaps a nest holder captured at the time of sampling was merely a temporary visitor at the nest (e.g., there to cannibalize the embryos of another male). Egg thievery or nest raiding (i.e., the theft of a few eggs from a neighbor’s nest) has been documented in sticklebacks (Jones, Östlund-Nilsson, et al. 1998; Li and Owings 1978; Rico et al. 1992). Such egg raiding might seem counterintuitive from an evolutionary perspective, but one plausible explanation is that the behavior benefits the thief by seeding or priming his own nest with eggs that are known to be effective in many fish species in eliciting spawning responses by additional females, with whom the resident male then mates (review in Porter et al. 2002).
For males of many fish species, various body parts or pigmented regions have evolved a close resemblance to conspecific fish eggs. In fantail darters (fig. 7.17), each spine on the foremost dorsal fin carries one round glob that looks just like a fish egg. In other darter species, these egglike globs are located on the spiny tips of a posterior dorsal fin, and in yet another group of darters they reside on pelvic fins near the male’s throat. Thus, egg mimicry structures have evolved independently several times in various darter lineages (Page and Bart 1989). In many darter species, males nest in cavelike openings in a streambed, where females cement eggs to the cave’s ceiling or walls. Primitive versions of these fleshy protuberances in ancestral darters may have served to blunt a bourgeois male’s sharp spines, which otherwise could puncture some of the eggs that line his nesting chamber (Page and Swofford 1984). Only later in evolution did the structures presumably become refined and useful also as egg mimicry devices that might help a male to attract females to lay bona-fide eggs in his nest.
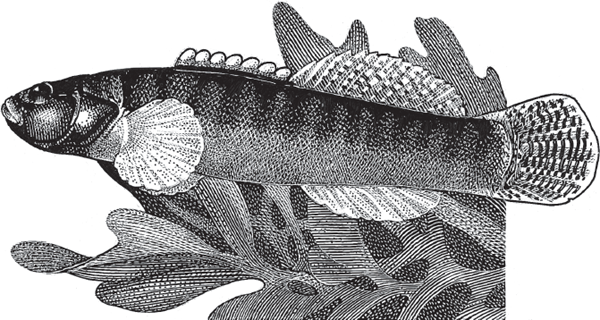
FIGURE 7.17 One of several darter species (family Percidae) in which body parts of the male have evolved to resemble fish eggs. In this case, egglike structures are located on spine tips of the male’s anterior dorsal fin.
For many nest-tending and mouth-brooding fish species, it is well documented that females prefer to spawn with males that seem to display or tend eggs (Knapp and Sargent 1989; Porter et al. 2002). This might be because such eggs are an indicator of good health in a potential mate, or they may speak favorably of a male’s parenting skills. Indeed, this is one reason that occasional egg thievery and nest piracy in nest-tending fishes (as described earlier) might make evolutionary sense after all. By stealing a few fertilized eggs or pirating a nest, a male may be priming a reproductive pump that brings additional unfertilized eggs into his nest from gravid females who are impressed with his apparent parenting potential.
FIGURE 7.18 Observed correlation (r = 0.74, P < 0.05) between numbers of egg-mimic spots and genetically deduced numbers of mates secured by nest-tending striped darter males (after Porter et al. 2002).
Factoid: Did you know? Egg-mimic spots can have other functions, too. In some mouth-brooding cichlids, males on their anal fins have “egg dummies” to which females are attracted. As the female mouths the dummies (just as she does her own bona-fide eggs), the male releases sperm and fertilizes the eggs that she carries within her mouth.
Factoid: Did you know? “Sperm drinking” is an even more bizarre form of insemination displayed by armored catfishes in the family Callichthyidae. In these species, a female swallows a male’s sperm, quickly passes the ejaculate through her digestive system, and then releases still-viable sperm to fertilize eggs that she holds between her pelvic fins (Kohda et al. 1995).
In most darter groups, the number of egg-mimic structures per male is essentially constant because each fish has a fixed number of fin rays. However, in one population of the striped darter (Etheostoma virgatum), the number of egg look-alikes varies from half a dozen to more than 20 per male. This is because, unlike the physical egg-mimic structures in the other darter species, these egg look-alikes are unpigmented spots on the darker background of the male’s pectoral fins. Because of this variable number of faux eggs on bourgeois males, Etheostoma virgatum offered researchers an opportunity to test the egg-mimicry hypothesis empirically. Do males with more mimetic spots spawn with more females than do males with fewer spots? To answer this question, Porter et al. (2002) used genetic maternity assignments for dozens of embryos in the nests of several striped darter males with varying numbers of egglike spots. Their results supported the notion that females spawn preferentially with males that display more of these spots (fig. 7.18).
Perhaps this is an honest display—male darters with many egg look-alikes might be healthier or of higher genetic quality on average and are merely advertising that truthful fact to females. On the other hand, the egg-mimic spots might be deceitful displays by males, signifying little or nothing about their true parenting abilities. This demonstrates that deceptive and honest displays can both be the objects of various forms of sexual selection in fishes and other species.
Cannibalism (eating other members of one’s own species) is a common phenomenon in the animal world and often makes good sense because successful cannibals may thereby increase their own survival and reproduction at the expense of their competitors for food, space, or mates. But why might a parent eat its own offspring as well, given that progeny carry copies of its parent’s genes? Yet “filial cannibalism” seems to occur in nest-tending fish species, where a guardian male is occasionally observed eating a few eggs or fry from the nest he is tending (Fitzgerald 1992). Filial cannibalism is different from “heterocannibalism” (the consumption of conspecific nonrelatives). Why might a sire consume some of his own offspring?
Several explanations are possible. Perhaps natural selection favors filial cannibalism when a nest-guarding male is otherwise facing starvation. Although clearly detrimental to those who are eaten, such cannibalism may be beneficial to the male and to his remaining kin if at least some members of the current and subsequent broods survive. A second possibility is that the eggs or fry that a male eats from his nest are infected with a fungus or are otherwise diseased and would not survive anyway. Indeed, by eating infected eggs, a sire might stop the spread of disease within his nest. A third possibility is that filial cannibalism is simply a nonadaptive behavioral byproduct of a fish’s voraciousness. In terms of the final tally of offspring produced, it probably matters little if a few babies are consumed from a nest that may contain scores or hundreds of eggs and fry.
A fourth viable hypothesis (especially given the high incidence of cuckoldry, as described early) is that a nest-tending male is actually ingesting foster progeny rather than his own. Because many fry in a nest now are known from genetic-paternity analyses to be tended by a foster father, might some purported instances of filial cannibalism actually register as heterocannibalism instead? To address this possibility, DeWoody, Fletcher, et al. (2000) genetically examined freshly eaten embryos retrieved from the stomachs of cannibalistic male tessellated darters (fig. 7.19) who were tending their nests. From genetic paternity analyses conducted on dozens of partially digested embryos, these researchers proved that the bourgeois males indeed had eaten some of their own biological progeny. Thus, filial cannibalism is a real phenomenon after all.

FIGURE 7.19 The tessellated darter, Etheostoma olmstedi, a species in which males tend nests in gravel streambeds and sometimes eat a few of their own offspring.
1. In contradistinction to natural selection that forges adaptations to environmental demands, sexual selection molds behaviors and other phenotypes to mating demands. Woven into a tapestry of topics, sexual selection includes mating behaviors, mating systems, sex ratios, sexual dimorphism, and other sexual phenomena that ultimately trace their evolutionary geneses and gender biases to anisogamy. Female pregnancy also fits into this conceptual framework since it is both a logical outgrowth of anisogamy and an amplifier of anisogamy’s effects, especially with regard to sexual selection.
2. Sexual selection can be dichotomized in several ways: intrasexual/intersexual; precopulatory (typically via female mating preferences)/postcopulatory (via sperm competition and/or cryptic female choice); association with alternative mating systems, including monogamy and polygamy (polygyny, polyandry, and polygynandry); or operating primarily on males rather than females. Such characterizations are not mutually exclusive, but they do highlight the many overlapping ways in which sexual selection can operate.
3. Fish are excellent subjects for comparative appraisals of sexual selection because they include many representative species with internal female pregnancy, internal male pregnancy, and external male pregnancy (e.g., nest tending). The evolutionary origins and selective ramifications of different forms of pregnancy have been addressed using three broad approaches: phylogenetic character mapping (PCM) to deduce the evolutionary histories of particular pregnancy-related traits; field observations and experiments to unveil the functional significance of those traits; and molecular-parentage analyses to reveal the genetic (as opposed to the “social”) mating systems of particular fish species. The strongest conclusions typically emerge from successful mergers of several of these research approaches.
4. Molecular-parentage analyses are especially germane because each pregnant adult is physically associated with its brood, and this association greatly facilitates deductions about who spawned the brooded progeny. Genetic-parentage analyses have been conducted on many broods in a wide variety of viviparous fishes in which either females (e.g., in Poeciliidae) or males (in Syngnathidae) become pregnant. Genetic analyses have been likewise conducted in numerous nest-tending and other fishes with external fertilization and external male pregnancy. A complication of these latter species is that a bourgeois (nest-tending) male has sometimes been cuckolded via extrapair fertilizations. Genetic markers have confirmed that foster parentage also arises occasionally in nest-tending species via egg thievery and nest piracy. Additional phenomena addressed by genetic-parentage analyses include egg mimicry and female choice, filial cannibalism, alternative reproductive tactics (ARTs), Bateman gradients, and sexual selection in relation to alternative expressions of piscine pregnancy, sexual dimorphism, and genetic mating systems.