The most fundamental innovation is the evolution of another fluid-filled sac, the amnion, in which the embryo floats. Amniotic fluid has roughly the same composition as seawater, so that in a very real sense, the amnion is the continuation of the original fish or amphibian eggs together with its microenvironment, just as a space suit contains an astronaut and a fluid that mimics the earth’s atmosphere. All of the rest of the amniote egg is add-on technology that is also required for life in an alien environment, and in that sense it corresponds to the rest of the space station with its food storage, fuel supply, gas exchangers, and sanitary disposal systems.
—Richard Cowen, The History of Life
Like the transition from “fish” to “amphibians,” the transition from “amphibians” to “reptiles” has long been confused by misunderstandings and inadequate terminology. If we use the terms “fish,” “amphibians,” and “reptiles” in the traditional sense, they are not natural monophyletic groups, but “grades” of evolution because they do not include all descendants of a particular group. We have already discussed in chapter 10 how the monophyletic group Tetrapoda is preferred to the archaic term “amphibian.” The same goes for the grade we call “reptiles.” If you look at the living turtles, snakes, lizards, and crocodiles, they all have scaly skins and sluggish metabolisms, and it is easy to lump them together based on these shared primitive characteristics. But as we will detail in the next chapter, birds are their descendants, so unless you include the birds within the Reptilia, it is an unnatural paraphyletic “wastebasket” group (figs. 5.4 and 11.1). To avoid confusion with this long-standing misuse of the concept, most modern systematists use the term “Reptilia” to mean the clade that includes turtles, snakes, lizards, and crocodiles plus birds. The so-called “mammal-like reptiles” (more properly, the synapsids), on the other hand, branched off from the family tree before the branching point of true reptiles, so it is incorrect to label synapsids by that obsolete term (even though people keep using it). Likewise, there are many primitive tetrapods that are neither synapsids nor reptiles but more advanced than the creatures we saw in the previous chapter. To avoid the misleading term “Reptilia” for these animals, most modern systematists prefer to use the term “amniotes” for all vertebrates that are more advanced than traditional amphibians. The Amniota thus includes the classical concepts of Reptilia, Mammalia, and Aves (birds).

FIGURE 11.1. Family tree of the amniotes. (Drawing by Carl Buell)
The diagnostic character shared by all living amniotes is the land egg, or amniotic egg (fig. 11.2). Instead of laying hundreds of tiny soft-shelled eggs in the water (as fish and amphibians do), amniotes lay fewer but larger eggs that can survive out of water. Each egg is covered by a shell (either leathery like a turtle egg or hard like a chicken egg) that protects the delicate tissues and embryo inside against predators and prevents it from drying out.
FIGURE 11.2. Diagram showing the parts of the amniotic “land egg.” (From Romer 1959; used by permission of the University of Chicago Press)
The embryo is also aided by multiple specialized systems. It is surrounded by a membrane called the amnion, which is filled with amniotic fluid to buffer it from shock and temperature change. Attached to the gut of the embryo but outside the amnion is the yolk sac, which provides food for the embryo so it can hatch out relatively well developed and ready to face the world. A second sac off the hindgut of the embryo is known as the allantois, and it collects wastes and aids in respiration. Finally, the entire assembly—amnion, yolk sac, and allantois—is surrounded by another fluid, albumin (the “egg white”), which fills the rest of the volume of the egg. Just beneath the shell is a porous membrane known as a chorion, which helps hold in the fluids while allowing oxygen to enter and carbon dioxide waste to escape.
The amniotic egg has other implications. Each egg is more costly to produce, so fewer can be laid, and each embryo will be more completely developed and independent when it hatches. In addition, the egg cannot be fertilized (as is done in most fish and amphibians) by the male swimming near the egg cluster in the water and spraying them with sperm. Instead, the amniotic egg requires internal fertilization, usually involving sexual intercourse. Males and females must copulate so that the sperm can reach the eggs inside the female, and the eggs undergo much of their development inside her, not in the water. Internal fertilization has evolved more than once, of course. Most land-living arthropods (insects, spiders, scorpions, and so on) must copulate for the same reasons that amniotes do—they can’t spread their eggs and sperm around in water. In the sea, sharks are among the few non-amniotes that use internal fertilization, and they lay smaller numbers of large eggs, or some even give birth to live young that are fully developed.
How did the transition from primitive tetrapods to the amniotes occur? We cannot tell which extinct organisms known from fossils laid an amniotic egg, because eggs are rarely preserved with their parents. Only a few fossil eggs from the time of the first amniotes are known anyway. Instead, we must work with the features of the skeleton to decide which anatomical characters will diagnose a fossil as an amniote. Once again, we have a long series of transitional forms (the paraphyletic group known as “anthracosaurs”) that show progressively more and more amniote-like characteristics, and where to draw the line in this continuous series is problematic (fig. 11.3). We can see several trends in the evolution of anthracosaurs into amniotes. One is the fact that anthracosaurs have skulls that are very deep vertically, with a narrow snout and a shortened region behind the eyes. Their skulls eventually lose the notch in the back for the eardrum. By contrast, most other primitive tetrapods (such as the temnospondyls and lepospondyls) have relatively flat skulls and bodies, with a wide snout, a large region of skull roof behind the eyes, and a well-developed notch for the eardrum. Correlated with this is evidence that limbs of the anthracosaurs were robust and held upright, so they frequently walked with their bellies off the ground. Temnospondyls and lepospondyls had a much more sprawling posture and could rarely move without dragging their bellies. The wrist and ankle bones of anthracosaurs were also modified for much more active motion than the comparable bones in other primitive tetrapods. The neck vertebrae of anthracosaurs were specialized into two bones: the atlas (which holds up the skull, as Atlas once held up the world in Greek mythology) and the second neck vertebra, the axis (the pivot joint on which the skull turns in relation to the neck). These are features found in all later amniotes and allowed anthracosaurs to swivel their heads rapidly to catch prey. Finally, the muscles and bones of the anthracosaur palate were modified so it had a much stronger bite force. By contrast, the palate of temnospondyls and lepospondyls had a much weaker “snapping” motion, with nowhere near as much bite force.
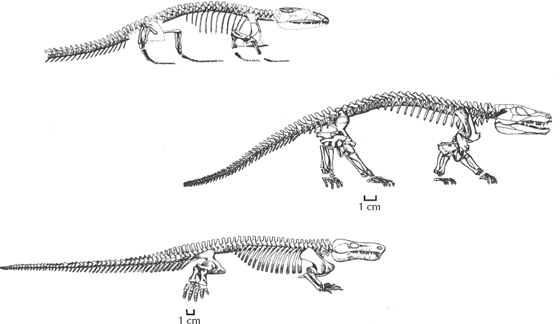
FIGURE 11.3. Skeletons of some of the many “anthracosaurs,” which are transitional fossils between “amphibians” and the most primitive amniotes. At the top is the primitive gephyrostegid Bruktererpeton. In the middle is the more advanced form Seymouria. At the bottom is the highly tetrapod-like Limnoscelis. Each one is a mosaic of primitive tetrapod and advanced amniote characters, making it very difficult to decide where the amphibians end and the amniotes begin. (From Carroll 1988: fig. 9-22; courtesy W. H. Freeman and R. L. Carroll)
As we move up the sequence of anthracosaurs, we see these features accumulating one by one in a mosaic evolution pattern (fig. 11.3). For example, the more primitive Solenodonsaurus has the primitive eardrum notch but advanced jaw muscles. Gephyrostega also retains the primitive eardrum notch and primitive vertebrae and limbs but has an advanced ankle joint. Limnoscelis and Seymouria have lost the primitive eardrum notch and also have more advanced, robust limbs. Diadectes, a pig-sized herbivore (the first herbivorous land vertebrate known), is very close to amniotes, with the atlas and axis vertebrae in its neck and strong limbs with advanced ankles, but it still retains the primitive eardrum notch.
Most of these anthracosaurs are apparently end-members of an extinct side branch representing earlier lineages that were not preserved in the fossil record because the oldest fossil that most paleontologists agree is an amniote comes from older beds of the Lower Carboniferous. Nicknamed “Lizzie the lizard” by its discoverer, Stan Wood, this fossil is officially known as Westlothiana lizziae (fig. 11.4) and comes from the famous East Kirkton beds of Scotland (Smithson et al. 1994). Slightly later, true amniote fossils known as Hylonomus come from the Middle Carboniferous beds of Joggins, Nova Scotia (about 15 million years younger than “Lizzie”). These fossils show a dramatic change from the more primitive tetrapods. They are small (about 20 centimeters long, or the size of a typical lizard) rather than the much bigger dog-sized and even pig-sized anthracosaurs. They were also delicate and slender, with a very long trunk and tail, long slender limbs and toes, and relatively small heads in comparison to most anthracosaurs. Their vertebrae are robust and fused into the arches above the spinal column, a typical feature of amniotes. Westlothiana and Hylonomus had deep skulls with effective jaw muscles and no eardrum notch in the back of the skull. They also had relatively large eyes, suggesting that they may have been night predators, catching insects and other small prey. The best specimens of Hylonomus were found preserved inside hollow rotten tree trunks in the famous Joggins locality, suggesting to some that they had been trapped in these deep holes and died there. More recently, paleontologists have argued that the long delicate limbs and fingers suggest that they were good climbers like many modern lizards and probably lived in the hollows of trees, where they were occasionally buried and fossilized.
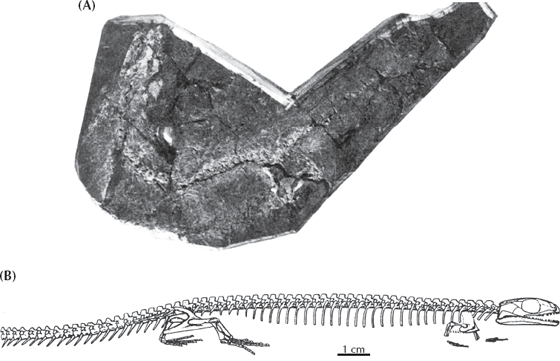
FIGURE 11.4. The oldest known true amniote, Westlothiana lizziae, showing both (A) the actual specimen and (B) a reconstruction of its skeleton. (Reproduced by permission of the Royal Society of Edinburgh and R. L. Carroll; from Smithson et al. 1994)
From early forms like Westlothiana and Hylonomus, the amniotes radiated into many different groups by the Late Carboniferous (fig. 11.1). One of the earliest lineages to branch off was the synapsids, which eventually gave rise to mammals. They will be discussed in chapter 13. The other lineage is the one we will call the true Reptilia. Its most primitive branch is the group known as Anapsida, which includes the turtles as well as several extinct groups. The next major branch is the Euryapsida, which includes most of the marine reptiles. The third branch is the Lepidosauria, which includes not only the living lizards and snakes but also extinct groups like the marine reptiles known as mosasaurs. The final branch is the Archosauria, or “ruling reptiles,” and these include a lot of familiar creatures: crocodilians, pterodactyls, dinosaurs, and birds. For space reasons, we cannot go into detail with each of these groups or look at every transitional form in every lineage. Instead, we will focus on a few examples of the many transitional forms found in certain lineages to demonstrate just how good the fossil record of these creatures has become.
Turtle on the Half Shell
Creationists often mock scientists and proclaim how impossible it is to imagine a creature that is “half a turtle.” Once again, the fossil record has answered them with a perfect transitional fossil (Li et al. 2008) that rebukes them in turn. Officially known as Odontochelys semitestacea, its name literally means “toothed turtle with half a shell.” A number of specimens have been found in Triassic deposits of China, and they are truly remarkable (fig. 11.5). They have a fully developed shell on their belly (the plastron), but their backs are just broad expanded ribs without a back shell (the carapace). They are literally “turtles on the half shell.” In addition, they are the last known turtle to have teeth. All more advanced turtles have toothless beaks. Thus, they bridge the gap between more lizard-like reptiles and turtles with fully developed shells on their back and belly.

FIGURE 11.5. Odontochelys: (A) the best of the known fossils, showing an incomplete carapace on its back (left), but a complete plastron on its belly (right); (B) reconstruction of its appearance in life. ((A) Courtesy Li Chun; (B) courtesy Nobumichi Tamura)
If a creationist replies by saying, “Where is the transition between this fossil and other reptiles?,” we have those fossils too. Eunotosaurus is an extinct amniote from the Permian of South Africa that has the broadly expanded back ribs of Odontochelys and more advanced turtles, along with some other features of the skull and skeleton, that link it to turtles—yet to the untrained eye it looks like a large, fat lizard. Then, in 2015, my friend Hans-Dieter Sues of the Smithsonian Institution announced Pappochelys (“grandfather turtle”), which not only has the broad back ribs of Eunotosaurus but also the broad flattened belly bones (“gastralia”) that would eventually fuse to become the belly plate (plastron) of Odontochelys. This makes it a nice transition from Eunotosaurus to all the rest of the turtles—but it has only broad flat ribs on its belly and back, not a fused shell.
Thus, from the very lizard-like Eunotosaurus, we now have a complete sequence to Pappochelys, Odontochelys, and then to fossils that even a creationist could recognize as a turtle. These discoveries were made just in the past decade since the last edition of this book was published. Just imagine how many more transitional fossils we might have in another 10 years!
There were no real sea serpents in the Mesozoic Era, but the plesiosaurs were the next thing to it. The plesiosaurs were reptiles who had gone back to the water because it seemed like a good idea at the time. As they knew little or nothing about swimming, they rowed themselves around in the water with their four paddles, instead of using their tails for propulsion like the brighter marine animals. (Such as the ichthyosaurs, who used their paddles for balancing and steering. The plesiosaurs did everything wrong). This made them too slow to catch fish, so they kept adding vertebrae to their necks until their necks were longer than all the rest of their body…. There was nobody to scare except fish, and that was hardly worthwhile. Their heart was not in their work. As they were made so poorly, plesiosaurs had little fun. They had to go ashore to lay their eggs and that sort of thing. (The ichthyosaurs stayed right in the water and gave birth to living young. It can be done if you know how.)
—Will Cuppy, How to Become Extinct
During the “age of the dinosaurs” or the Mesozoic Era, the seas teemed with many different types of life: enormous numbers of plankton, gigantic clams on the seafloor, as well as weird oysters like Gryphaea (fig. 8.10), many types of sea urchins and heart urchins (fig. 8.9), squid-like belemnites and ammonites, a great diversity of “holostean” and eventually teleost fish (see fig. 9.12), but the dominant predators of all these creatures were marine reptiles. These include not only huge sea turtles up to 7 meters (22 feet) long and crocodiles known as geosaurs, which had webbed feet and a tail fin, but also three major groups that were unique to the Mesozoic seas: the dolphin-like ichthyosaurs, the long-necked paddling plesiosaurs, and the seagoing Komodo dragons known as mosasaurs.
In marine beds over many parts of the world, we have excellent, often complete skeletons of these creatures. In the western Great Plains (especially South Dakota, Colorado, and Kansas), there are extensive exposures of marine sediments that were deposited in the Cretaceous when a great inland sea covered the Plains region from the Gulf of Mexico to Hudson’s Bay. Each of these creatures is so distinctive that it would clearly be a “created kind” to a creationist. And yet we have excellent evidence of the origins and relationships of all three groups both from transitional fossils and from the cladistic analysis of their relationships. If they were not extinct, we might check their molecular phylogeny as well.
The most amazing thing about all three groups is that they were clearly reptiles, so they were descended from terrestrial creatures that developed a land egg, yet these three all independently returned to the oceans (as did crocodilians, sea turtles, sea snakes, seals and sea lions, and whales, of course). Apparently, the food resources were so great in the oceans that land-dwelling reptiles found their way to reap this bountiful harvest in at least five different groups. This seems amazing in itself, yet the fact that it has happened many times shows how powerful the selection forces for this lifestyle must be. Such a radical change in ecology usually caused much convergence in body form as well, so we can see how ichthyosaurs and whales have independently evolved the streamlined torpedo-like shape that is also found in fish. Returning to the ocean makes certain reproductive and physiological demands, in addition to streamlining the body for swimming and modifying the hands and feet into flippers. For example, marine reptiles must still reproduce somehow. We know that sea turtles and saltwater crocodilians crawl out on land and lay eggs in a nest, and presumably mosasaurs and plesiosaurs could have done so too. But ichthyosaurs are so dolphin-like in body form that they could not have wriggled onto a beach and dug a nest with their flippers. We know that whales and dolphins give live birth, expelling the young from the womb and raising it up to take its first breath, after which it can swim on its own. Apparently, ichthyosaurs could also, since there are several remarkable specimens from the Jurassic Holzmaden shales in Germany that appear to have been in the process of giving birth to live young when they died and were fossilized (fig. 11.6).
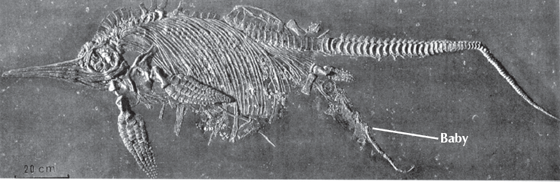
FIGURE 11.6. The famous specimen of a female ichthyosaur giving birth to a live baby, getting caught in the act, and then being fossilized in the Jurassic Holzmaden Shale of Germany. (Image courtesy of the State Museum of Natural History, Stuttgart)
Let us focus on the ichthyosaurs first. They represent the greatest challenge because they are the most highly modified and specialized for marine life. They have highly fishlike streamlined bodies with long toothy snouts for catching fish and squid, huge eyes for seeing in dark murky waters, a well-developed dorsal fin, and both the hands and feet are fully modified into flippers. Finally, their tails also have a vertically oriented tail fin. In contrast to the tailfin of most fish, the supporting spinal column of ichthyosaurs flexes downward into the lower lobe of the fin, not upward as in sharks and other primitive fish. A detailed analysis of the skeletal characters of the group shows they belong to the Euryapsida, along with the plesiosaurs, which we’ll discuss next (fig. 11.1). The Euryapsida, in turn, are the sister group of the rest of the reptiles.
A number of striking intermediate forms are known from the early Mesozoic (fig. 11.7). First, there is Nanchangosaurus from the Triassic of China. Although it has a slightly streamlined body and a long (but toothless) snout like an ichthyosaur, all of the rest of the features of the skeleton are primitive, including the vertebrae; the limbs, which are not modified into flippers but have normal proportions with all the regular wrist and ankle and toe bones; and a long straight tail with no sign of a tail fin. The original authors were not sure where to put this fossil because it is so primitive, but based on the skull, it seems to be an aquatic lizard on the way to becoming an ichthyosaur.
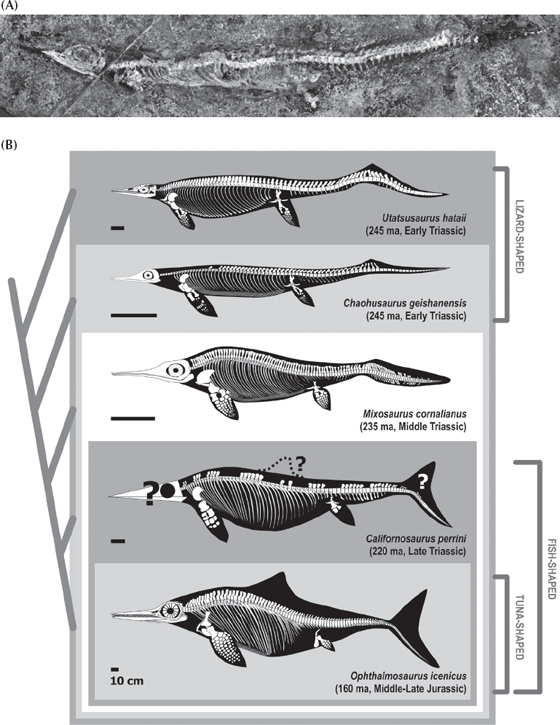
FIGURE 11.7. The ichthyosaurs evolved from lizard-like forms with asymmetric tails (Utatsusaurus and Chaohusaurus), primitive amniote skulls, and primitive hands and feet, to the highly specialized ichthyosaurs with symmetrical tails, large dorsal fins, big eyes, long fish-catching snouts with conical teeth, and highly modified flippers. (A) A complete articulated skeleton of the very primitive lizard-like ichthyosaur Chaohusaurus from the Early Triassic of China. (B) Diagram showing the evolutionary transformations from more primitive to more specialized ichthyosaurs. (© Ryosuke Motani, http://ichthyosaur.org)
The oldest known fossil that can definitely be called an ichthyosaur is Utatsusaurus from the Early Triassic of Japan (fig. 11.7B). Although it has the general body form of an ichthyosaur, it has a mosaic of primitive features found in its reptilian ancestors. These include a skull with only a short snout and unspecialized teeth, very primitive vertebrae (especially in the neck), hands and feet that are not yet highly modified into flippers but still have discrete fingers and toes, and a long straight tail with no evidence of the downward flexion to support a vertical tail fin. Another specimen, Grippia from the Early Triassic of Spitsbergen, is known primarily from the skull, but it shows a relatively short snout, small eyes, and simple knob-like teeth for crushing mollusks, not the spiky teeth of most fish-eating ichthyosaurs. Yet another Early Triassic form from China, Chaohusaurus (fig. 11.7A and B) also has a short snout, simple teeth, primitive vertebrae, and robust limbs that are beginning to form a paddle but still have discrete rows of finger bones with the normal count (not the extra bones of an advanced ichthyosaur paddle—see fig. 11.7A). Cymbospondylus from the Middle Triassic of Nevada still retains the primitive hand and foot structure as well and has a relatively short snout with small eyes, and the tail is beginning to show the downward bend that indicates the presence of a small tail fin.
The best known and best preserved of the early ichthyosaurs is Mixosaurus from the Middle Triassic of Germany (fig. 11.7B) and many other places. The body has the classic ichthyosaur shape, with the long snout, large eyes, and dorsal fin. The hands and feet are beginning to form flippers, although they have still not multiplied the finger and toe bones as in later ichthyosaurs. And the tail shows just a slight downward bend, with some specimens preserving the body outline and showing that it had a small upper lobe on its tail. Thus it is advanced in many features but still retains the primitive hands and feet and does not yet have the fully bilobed ichthyosaurian tail.
If the ichthyosaurs seem very highly specialized, the plesiosaurs are specialized in a different direction. All of the advanced forms had stout bodies with robust shoulder and hip bones and four large well-developed paddles (fig. 11.8). Some plesiosaurs (especially the elasmosaurs) had long serpentine necks and small heads, while another group (the pliosaurs) had long heads and snouts and much shorter, more robust necks. Instead of employing speedy dolphin-like swimming like ichthyosaurs, plesiosaurs were apparently adapted for slow steady swimming by rowing with their fins (like a sea turtle does) and used their long heads and necks to snap at prey that came within reach.
How could such peculiar “kinds” evolve? We have an even better series of intermediates for plesiosaurs than we do for ichthyosaurs. They start with the Late Permian fossil Claudiosaurus from Madagascar (fig. 11.8A). It is so primitive that it may actually be the first known euryapsid, and a sister group to both the plesiosaurs and the ichthyosaurs. However, it shows the condition from which these euryapsids evolved. In most features, it looks just like many other primitive reptiles of the Permian, except that the skull has the characteristic holes, features of the palate, and loss of the temporal bar that earmark it as a euryapsid. In addition, it seems to show the beginning of aquatic adaptations with the loss of the breastbone. This enables it to swim with both limbs moving at the same time in a swimming stroke, not alternating like the gait of a lizard. It also has relatively long limbs and especially long toes, indicative of webbed feet. In fact, its limb proportions closely resemble those of modern aquatic lizards, such as the Galapagos marine iguana. Finally, a large part of the skeleton was reduced to cartilage, another indication of an aquatic lifestyle, since it reduces the weight of bone in a body supported by water and not needing such a robust bony skeleton.
From Claudiosaurus we next see a group of Triassic marine reptiles known as nothosaurs (fig. 11.8B). These animals had skulls and bodies that were not noticeably different from primitive euryapsids like Claudiosaurus. The biggest difference occurs in the neck, which is much longer and anticipates the long necks of many plesiosaurs. The limbs are not much more specialized for aquatic locomotion than those of its primitive relatives, but they have further reduced a lot of the bone to cartilage, another sign of a largely aquatic lifestyle. However, the shoulder girdle and hip bones are becoming much more robust and plate-like in support of the limbs, a hallmark of later plesiosaurs.
Our final step into full-fledged plesiosaurs is Pistosaurus from the Middle Triassic of Germany (fig. 11.8C). This creature has a relatively primitive head with a slightly longer snout than nothosaurs (but still retaining the nasal bones, which are lost in plesiosaurs), but its palate is more like that of plesiosaurs. The rest of its body is also fairly advanced, with a fairly long neck, deep body, many extra bones along the belly (gastralia), and limbs that are intermediate between the unspecialized nothosaur foot and the highly specialized plesiosaur paddles, which have dozens of extra finger bones (similar to what happened with ichthyosaur paddles—see fig. 11.7).

FIGURE 11.8. A transitional sequence of fossils bridging the gap from primitive amniotes to highly derived plesiosaurs. (A) Claudiosaurus from the Permian of Madagascar, with the primitive short neck, long tail, and relatively large hands and feet not yet modified into flippers. (B) The Triassic nothosaur Pachypleurosaurus, with longer neck, shorter more robust tail, and hands and feet more highly modified for swimming. (C) The Triassic Pistosaurus, a relatively primitive plesiosaur, with longer limbs partially modified into paddles, a longer neck, shorter tail, and longer skull. (D) The advanced plesiosaurs Cryptocleidus (top) and elasmosaurid Hydrothecrosaurus (bottom), with much longer necks, smaller heads, shorter tails, and hands and feet fully modified into flippers. (From Carroll 1988: figs. 12-2, 12-4, 12-10, and 12-12; courtesy W. H. Freeman and R. L. Carroll)
Finally, we leave these two euryapsid groups and look at the third example of large marine reptiles in the Mesozoic. This group is known as the mosasaurs, and they looked essentially like gigantic Komodo dragons adapted for swimming—which they were. Mosasaurs are members of the family Varanidae, or monitor lizards, which includes not only the Komodo dragon but also all the Australian goannas of Crocodile Hunter fame. Although not as highly specialized as plesiosaurs and ichthyosaurs, mosasaurs are completely aquatic, with long bodies, fully developed flippers, and a vertical fin on the tail. Once again, we have beautiful transitional forms, known as the aigialosaurs (fig. 11.9) from the middle Cretaceous of the Adriatic region. As DeBraga and Carroll (1993) and Carroll (1997:324–325) have shown, aigialosaurs are perfectly intermediate between mosasaurs and their varanid ancestors. The aigialosaurs have at least 42 anatomical characters that make them more advanced than varanids, most of which are concentrated in the skull region and the semiaquatic limbs (but otherwise aigialosaurs retain the primitive varanid skeleton). There are another 33 character transformations between aigialosaurs and the most primitive true mosasaurs, most of which involve developing flippers, extending the body, and developing a vertical fin on the tip of the tail. The reader is invited to examine DeBraga and Carroll’s (1993) paper in detail and see how a nice transitional sequence of fossils can be documented.

FIGURE 11.9. A number of transitional snake fossils with vestigial legs and hip bones are known from the Cretaceous. (A) Eupodophis descouensi, with tiny vestigial hind legs. (B) Detail of the leg bones in the same specimen. (Photos courtesy M. Caldwell) (C) The complete articulated skeleton of the Cretaceous snake with legs known as Haasiophis. The large cubes are cork spacers to prevent the fossil from being damaged when it is turned upside down. (D) Detail of the hip region, showing the vestigial hind limbs. (Photos courtesy M. Polcyn, Southern Methodist University) (E and F) The transitional fossil Adriosaurus, which had functional hind limbs but vestigial forelimbs, and a long snake-like body. (After A. Palci and M. W. Caldwell, 2007, Journal of Vertebrate Paleontology 27:1–7)
Snakes are vertebrates and vertebrates are classified as higher animals, whether you like it or not. I mean you can be a higher animal and still be a snake. This seems to be a rather peculiar arrangement, to be sure. If you can think of a better, let’s have it…. Snakes in a word, are well worth knowing, unless you’d rather know something else. In closing, I have a little message which I wish you’d relay to some of those people who won’t read a snake article because it gives them the jumps: there are no snakes in Iceland, Ireland, or New Zealand. And no snake articles.
—Will Cuppy, How to Become Extinct
If ever there were a classic “created kind,” it would have to be the snakes. After all, the serpent supposedly tempted Eve in the Garden of Eden and was forever condemned to crawl on its belly. Most people have bad feelings about snakes for a variety of reasons on which psychiatrists have long speculated. To a great extent, it is probably because there are many different species of venomous snakes, so all sorts of animals (including ourselves) have evolved a natural fear of them. But snakes can be wonderful, too. They are amazing machines of adaptation, with the ability to completely stretch their skulls and mouths around a prey item much larger than their head, and their incredible adaptations for living in every environment, from the desert to the jungle treetops to the open ocean. Only the cold temperatures of the polar and subpolar regions prevent them from living in those habitats. They are tremendously diverse, too, with over 20 families and dozens of genera and species alive today.
The odds of finding “missing links” for the “snake kind” seem astronomical. Snake skulls and skeletons are made of hundreds of tiny, lightweight bones that break up easily when the snake dies and have a poor potential for fossilization. Only a handful of fossils of complete snake skeletons are known. Most fossil snake species are known only from isolated vertebrae. Thus we have a very patchy fossil record for snakes in general, as would be expected for animals with such poor potential for preservation.
However, occasionally we get lucky. In some extraordinary localities like the Eocene Messel lake beds in Germany, we get complete articulated snake skeletons of essentially modern-looking snakes. And for some reason, we also got lucky when snakes were first branching out from their lizard ancestors in the Cretaceous. A number of fossil snakes with hind limbs (fig. 11.9) are known from the mid-Cretaceous of Israel, Lebanon, and Croatia, including Eupodophis, Pachyrhachis, and Haasiophis. In 2006, a slightly older fossil snake, Najash rionegrina, was reported from the mid-Cretaceous of Argentina. It not only had hind limbs but fully functional hip bones as well. Then, in 2007, an even better transitional fossil was reported from rocks in Slovenia that are 95 million years old. Dubbed Adriosaurus microbrachis, it was an extremely long-bodied marine lizard with fully functional hind limbs but tiny vestigial front limbs (fig.11.10E and F), showing yet another step between normal lizards and completely legless snakes. Finally, in 2015, the fossil Tetrapodophis (“four-legged snake”) was found in beds from the Early Cretaceous of Brazil. It has four tiny limbs, but they are clearly too small to have any real function, and must be vestigial (Martill et al. 2015).

FIGURE 11.10. The evolutionary transition from small bipedal archosaurs like (A) Gracilisuchus, which has only a few crocodilian features in its anatomy, and (B) Terrestrisuchus, which is more quadrupedal with a longer snout, but still lightly built, to (C) Protosuchus, which has more typical crocodilian features, although it is still smaller and much more lightly built than any living crocodilian. (From Carroll 1988: figs. 13-22, 13-24, and 13-25; courtesy R. L. Carroll)
This should come as no surprise; we know snakes evolved from lizards that have emphasized locomotion driven by the sinuous motion of their bodies. In fact, a number of lizards, such as the skinks, have highly reduced limbs that are almost nonfunctional. And the legless snakelike body form has evolved several times in the vertebrates, not only in the snakes but also in another living group of reptiles known as amphisbaenids, in a living group of amphibians known as apodans, and in an extinct group of lepospondyl amphibians known as aistopods. Finally, the proof is found in the living snakes as well. A number of groups, such as the boids, still retain the vestiges of their hind limbs and pelvis (fig. 4.9).
Where did snakes come from? What is the nearest relative of snakes? This is a hotly debated topic. Some scientists point to evidence that links them with the marine mosasaurs (Caldwell and Lee 1997), while others cluster them with the amphisbaenid lizards (Rieppel et al. 2003), and the molecular evidence seems to link them with anguimorph and iguanid lizards (Harris 2003). Clearly, the jury is still out, and we have a lot of work to do. The biggest problem is that the snake skeleton is so specialized, with so many elements reduced or lost, that it is hard to make comparisons with any other group of reptiles. But whether we know the proper sister group or not, we can see that snakes once had legs and were descended from some group of lizards that could walk.
We do not have space to talk about the transitional forms for many other fascinating reptiles, and we will save the dinosaurs for the next chapter. But we should mention one other surprising example: the origin of crocodilians. Today we think of crocodilians as huge, armored, dangerous reptiles that haunt bodies of water, disguised at floating logs until suddenly they lunge at their prey and grab it and drag it underwater in their “death roll.” Thanks to movies like Crocodile Dundee and the TV show Crocodile Hunter with the late Steve Irwin, we have heard a lot more about crocodiles than any other reptile.
Crocodiles have a tremendously diverse fossil record, with many different forms, from 50-foot-long giants that ate dinosaurs for breakfast, to highly specialized marine crocodiles with flippers and tail fins, to the deep-jawed “bulldog” crocodilian Sebecus, to the peculiar fish-eating gavials (figs. 11.10 and 11.11). What is most surprising is that crocodiles didn’t start out large, heavy, or long-jawed. The earliest crocodiles were nothing like any of their later descendants or like any of the living species. They were small (about a half a meter to a meter, or 2–3 feet long), delicate, and long-legged with a relatively short snout and long, thin tail. In the Triassic, we have delicate creatures like Saltoposuchus. Others, like Gracilisuchus (fig. 11.10A) were apparently bipedal, walking mainly on their long hind limbs, since their front limbs were so short. Still others, like Terrestrisuchus (fig. 11.10B), were quadrupedal but extremely delicate, with long slender legs, and probably were excellent runners. Finally, by the Early Jurassic we have Protosuchus (fig. 11.10C), whose name means “first crocodile” because it was one of the first early crocodilians to be described. This creature has a more classic four-legged stance, but the limbs are still long and delicate, and the snout is still short and slender.

FIGURE 11.11. From small lightly built forms like Sphenosuchus and Protosuchus (bottom left), the crocodilians have radiated into a wide variety of body and skull shapes and ecological niches, including fully marine crocodiles with flippers and tail fins like the Thalattosuchia, crocodilians with long limbs and deep skulls like dinosaurs (Sebecosuchia), crocodilians with long narrow snouts (Tethysuchia), and the many differently shaped relatives of the living Crocodylia (upper right). (Courtesy D. Naish)
How do we know that these delicate creatures are crocodilians? They sure don’t look like it! But the superficial looks are deceiving. Crocodilians have a whole suite of distinctive anatomical features, particularly in the skull and ankle, that are unique and unmistakable. We don’t recognize something as a crocodilian fossil by its long snout with lots of teeth but instead by these more subtle and more reliable features.
All of these animals have body proportions not much different from the other primitive archosaurs of the Triassic, to which they are closely related. Thus we can trace the origin of crocodilians from the great branching event of many archosaur lineages in the Early Triassic through a variety of delicately built smaller forms to Protosuchus, and by the mid-Jurassic to crocodilians that resemble something we might recognize as such (fig. 11.11).
Finally, you might be wondering why crocodilians remained so small and delicate until the Jurassic. The answer probably lies in competition: during the Triassic, there were huge armored semiaquatic archosaurs, known as phytosaurs, which filled the niche that crocodilians would eventually occupy. In most respects, phytosaurs looked like dead ringers for crocs, from the large body size and armor, sprawling gait, and long tooth-filled snouts. But if you look closely, you can tell in an instant that it’s not a crocodilian. All crocodilians have their nostrils on the tips of their snouts. Phytosaurs have shifted the nostril openings to the top of the skull, just in front of the eyes. Apparently, phytosaurs prevented crocodilians from occupying that “croc niche” until they died out in the Late Triassic, and then the delicate Protosuchus-like crocodilians quickly evolved into large aquatic predators once the niche was open.
For Further Reading
Benton, M. J., ed. 1988. The Phylogeny and Classification of the Tetrapods. Vol. 1, Amphibians, Reptiles, Birds. Oxford, U.K.: Clarendon.
Benton, M. J. 2014. Vertebrate Palaeontology. 4th ed. New York: Wiley-Blackwell.
Caldwell, M. W., and M. S. Y. Lee. 1997. A snake with legs from the marine Cretaceous of the Middle East. Nature 386:705–709.
Callaway, J. M., and E. M. Nicholls. 1996. Ancient Marine Reptiles. San Diego, Calif.: Academic.
Carroll, R. L. 1988. Vertebrate Paleontology and Evolution. New York: Freeman.
Carroll, R. L. 1992. The primary radiation of terrestrial vertebrates. Annual Review of Earth and Planetary Sciences 20:45–84.
Carroll, R. L. 1996. Mesozoic marine reptile as models of long-term large-scale evolutionary phenomena. In Ancient Marine Reptiles, ed. J. M. Callaway and E. M. Nicholls. San Diego, Calif.: Academic, 467–487.
Carroll, R. L. 1997. Patterns and Processes of Vertebrate Evolution. New York: Cambridge University Press.
DeBraga, M., and R. L. Carroll.. 1993. The origin of mosasaurs as a model of macroevolutionary patterns and processes. Evolutionary Biology 27:245–322.
Gauthier, J. A., A. G. Kluge, and T. Rowe. 1988. The early evolution of the Amniota. In The Phylogeny and Classification of the Tetrapods. Vol. 1, Amphibians, Reptiles, Birds. M. J. Benton, ed. Oxford, U.K.: Clarendon, 103–155.
Laurin, M., and R. R. Reisz. 1996. A reevaluation of early amniote phylogeny. Zoological Journal of the Linnean Society of London 113:165–223.
Li, Chun, Xiao-Chun Wu, Olivier Rieppel, Li-Ting Wang, and Li-Jun Zhao. 2008. An ancestral turtle from the Late Triassic of southwestern China. Nature 456: 497–501.
Martill, D. M., H. Tischling, and H. R. Longrich. 2015. A four-legged snake from the Early Cretaceous of Gondwana. Science 349:416–419.
McGowan, C. 1983. The Successful Dragons: A Natural History of Extinct Reptiles. Toronto: Stevens.
Prothero, D. R. 2013. Bringing Fossils to Life: An Introduction to Paleobiology. 3rd ed. New York: Columbia University Press.
Rieppel, O. 1988. A review of the origin of snakes. Evolutionary Biology 25:37–130.
Rieppel, O., et al., 2003. The anatomy and relationships of Haasiophis terrasanctus, a fossil snake with well-developed hind limbs from the mid-Cretaceous of the Middle East. Journal of Paleontology 77:536–558.
Schoch, R., and H. D. Sues. 2015. A Middle Triassic stem-turtle and the evolution of the turtle body plan. Nature 523:584–587.
Schultze, H.-P. and L. Trueb, eds. 1991. Origins of the Higher Groups of Tetrapods: Controversy and Consensus. Ithaca, N.Y.: Cornell University Press.
Smithson, T. R., R. L. Carroll, A. L. Panchen, and S. M. Andrews. 1994. Westlothiana lizziae from the Visean of East Kirkton, West Lothian, Scotland, and the amniote stem. Transactions of the Royal Society of Edinburgh 84:383–412.
Sumida, S., and K. L. M. Martin, eds. 1997. Amniote Origins: Completing the Transition to Land. San Diego, Calif.: Academic.